1. INTRODUCTION
The current lifestyle trend leans towards natural-based practices, including healthcare and medicine (Coutinho Moraes et al., 2015; Elkordy et al., 2021; Insuasti-Cruz et al., 2022). This shift is driven by a desire for holistic well-being and a growing recognition of the benefits of plant-derived substances. Plant-derived medicines offer sustainable alternatives to synthetic drugs (Elkordy et al., 2021), align with environmental concerns (Theodoridis et al., 2023), and reduce reliance on non-renewable resources (Verpoorte et al., 2008). Advances in scientific research further support the importance of plant-based medicines as they uncover bioactive compounds with potential therapeutic applications (Gautam et al., 2023; Nyakudya et al., 2020). Eurycoma longifolia commonly referred to as pasak bumi or tongkat ali in the Indonesian and Malay languages is renowned for its medicinal properties in Southeast Asian countries such as Malaysia, Indonesia, and Vietnam (Bhat and Karim, 2010; Chua et al., 2005; Evans Schultes, 1980; Kassim et al., 2002). E. longifolia possesses a diverse array of bioactive compounds. These include quassinoids, β-carboline alkaloids, canthin-6-one alkaloids, triterpene-type tirucallane, derivatives of squalene, eurycolactone, eurycomalactone, laurycolactone, biphenyl neolignan, and bioactive steroids (Ang et al., 2000; Kuo et al., 2004; Mahfudh and Pihie, 2008; Miyake et al., 2009; Tran et al., 2014). The presence of these compounds contributes to the diverse pharmacological activities of E. longifolia, making it a subject of great interest for research and development of herbal products. This plant species grows naturally in Cambodia, Myanmar, and Thailand. In addition to its antimalarial, antipyretic, antiulcer, cytotoxic, and aphrodisiac properties (Bhat and Karim, 2010; Chua et al., 2005; Evans Schultes, 1980; Kassim et al., 2002) the root extracts have been traditionally used to enhance testosterone levels in men.
The significant value and affordability of this species has led to an increased market demand and intensive trade, both domestically and internationally (Sihotang and Rahmawati, 2019). Unfortunately, this high demand has led to illegal export practices and the potential overexploitation of E. longifolia in Indonesia (Susilowati et al., 2021). The sustainable utilisation of this species is compromised by the persistence of current practices. Consequently, it is imperative to establish systems capable of monitoring and delineating the origins of plants and their derived products, as demonstrated by DNA barcoding (Abubakar et al., 2018).
Molecular identification techniques, particularly DNA-based methods, play a pivotal role in the authentication and traceability of herbal products derived from E. longifolia. DNA markers have proven to be powerful tools for validating the authenticity and traceability of herbal and food products (Ganie et al., 2015) as in the case of Korean ginseng (Jung et al., 2014), manuka honey (McDonald et al., 2018), Orthosiphon stamineus herbal medicine (Liow et al., 2021), and processed hairtail fish products (Abdullah et al., 2019). These methods provide a reliable means of verifying the presence and authenticity of plant materials in various commercial products, including dietary supplements, traditional medicine, and herbal preparations (Fadzil et al., 2018; Gao et al., 2010; Han et al., 2016; Howard et al., 2019; Noh et al., 2018). By analysing specific genetic markers such as DNA barcodes, it is possible to differentiate E. longifolia from other related species and detect any adulteration or substitution that may occur in the market (Gao et al., 2010; Han et al., 2016).
Molecular identification methods have been widely used to confirm the presence of E. longifolia in commercial products. Studies have successfully employed techniques such as DNA sequencing, polymerase chain reaction (PCR), and DNA barcoding to assess the quality and authenticity of E. longifolia-based products (Abubakar et al., 2018; Vejayan et al., 2018). These molecular techniques provide accurate and reliable results, allowing for better regulation and control of the trade of E. longifolia products and ensuring consumer safety.
Although previous studies have authenticated E. longifolia products, the derivative products of this species have become increasingly diverse (Abubakar et al., 2018). In Indonesia, raw materials are extracted from the wild because of the species’ lack of cultivation or domestication (Pasaribu et al., 2021; Suwardi et al., 2022). This has led to a decrease in authentic raw materials, prompting the use of species falsely branded as E. longifolia (Li et al., 2013; Mohammed Abubakar et al., 2017). Consequently, the need for broader authentication efforts has been emphasised. Moreover, the diversity of adulterated materials varies between localities, regions, and countries based on the occurrence of complementary species (Soares et al., 2017), necessitating specific authentication methods tailored to each geographical context. This study aimed to authenticate the products or derivatives of E. longifolia (pasak bumi) produced, marketed, and consumed in Indonesia using molecular identification techniques.
2. MATERIALS and METHODS
A total of 37 leaf samples of E. longifolia were collected from the Sumatran mainland and Riau Islands, Indonesia (Table 1). Leaf samples were labelled, wrapped in sample envelopes, and dried with silica gel. In addition, six herbal products from the roots of E. longifolia (HPs) in various forms were also used (Table 2). In the present study, leaf samples of E. longifolia were used as the primary reference for authentication. These leaf samples served as crucial benchmarks for comparison with claimed derivative products of E. longifolia, including wood and roots. This approach allowed us to comprehensively evaluate and verify the authenticity of a wide range of E. longifolia products and provided valuable insights into the product origins and potential falsifications. The molecular analyses were performed at the Laboratory of Molecular Systematics, National Research and Innovation Agency, Indonesia.
Leaf samples of E. longifolia were isolated using a Genomic DNA Mini Kit (Plant) from GeneAid, and products from E. longifolia were isolated using a DNeasy Plant Mini Kit (QIAGEN, Valencia, CA, USA; HB-0542-003+1101205_PCard_DNY_Plant_Spi) following the manufacturer’s protocol. Two molecular markers, namely trnL-trnF and the whole internal transcribed spacer (ITS) regions, were selected for PCR amplification and DNA sequencing. The PCR amplification of the trnL-trnF region in E. longifolia leaf samples employed a set of universal primers, with the forward primer ‘c’ (5’-CGAAATCGGTAGACGCTACG-3’) and reverse primer ‘f’ (5’-ATTTGAACTGGTGACACGAG-3’; Taberlet et al., 1991). For the E. longifolia product sample, universal forward primer pair ‘c’ (5’-CGAAATCGGTAGACGCTACG-3’) and reverse primer ‘d’ (5’-GGG GATAGA GGGACTTGAAC-3’), forward primer ‘e’ (5’-GGTTCA AGTCCCTCTATCCC-3’), and reverse primer ‘f’ (5’-AT TTGAACTGGTGACACGAG-3’; Taberlet et al., 1991) were used. The PCR amplification of genomic DNA from E. longifolia leaf samples and its products from the ITS region was performed using universal primers. The forward primer pair ‘ITS 5P’ (5’-GGAAGGAGAAGTC GTAACAAGG-3’) and reverse primer ‘ITS 8P’ (5’-CACGCTTCTCCAGACTACA -3’) were utilised in this procedure (Möller and Cronk, 1997).
The preparation of the PCR mixture for genomic DNA from leaf samples involved a total volume of 12.5 μL. This comprised 6.25 μL of 2x My Taq HS Red Mix PCR buffer (Bioline, Meridian Bioscience, Newtown, OH, USA), 0.25 μL each of the forward and reverse primers, 4.75 μL of nuclease-free water (QIAGEN), and 1 μL of the DNA template. The PCR mixture for genomic DNA derived from E. longifolia products had a total volume of 13.5 μL. This included 0.375 μL of both the forward and reverse primers (5 pmol each), 0.25 μL of Taq DNA polymerase (Toyobo, Tokyo, Japan), 2.5 μL of dNTP (Toyobo), 6.25 μL of PCR buffer KOD FX Neo (Toyobo), 2.75 μL of nuclease-free water (QIAGEN), and 1 μL of the DNA template (10 ng/μL). The amplification reactions were conducted using the Sedi G Thermo Cycler (Wealtec Bioscience, New Taipei City, Taiwan) under optimal conditions: initial denaturation at 94°C for 3 min, denaturation at 94°C for 30 secs, annealing at 55°C for 30 secs, extension at 72°C for 1 min and 30 secs, and a final extension at 72°C for 4 min. The PCR amplification process was done over 35 cycles.
PCR products were visualised on a 1% agarose gel (Agarose Vivantis, Shah Alam, Malaysia) and stained with GelRed (Biotium, Hayward, CA, USA) using an electrophoretic process that lasted 30 min at 100 volts. After the electrophoresis process was completed, the target bands were photographed using a gel documentation system (Bioinstrument, ATTO Biosystems, Amherst, NY, USA). The amplified PCR products were sent to a 1st Base company for Sanger sequencing.
The compiled sequences from the forward and reverse sequences were integrated using ATGC version 4.3.5. The nucleotide composition of the trnL-trnF and ITS 5P-8P genes was assessed using MEGA X software (Kumar et al., 2016). To ascertain homology and identity, samples were aligned with the GenBank DNA database using BLASTn (https://blast.ncbi.nlm.nih.gov/ Blast.cgi). The BLASTn analysis identified the sequence with the highest percentage of similarity as the one most closely related to the query sequence. To reconstruct the phylogenetic tree, samples from this study were juxtaposed with reference sequences obtained from NCBI GenBank (https://www.ncbi.nlm.nih.gov/). Bayesian inference was conducted using MrBayes 3.6 (Huelsenbeck and Ronquist, 2001) integrated as plugins in Geneious Prime version 2021.0, with the HKY85 substitution model and a distributed gamma rate variation. The analysis involved two independent Markov chain (MCMC) runs of four chains for 1,100,000 generations, with tree topologies sampled every 200 generations. Burn-in periods, determined by the SD of split frequency values, were set to 100,000 generations, considering values < 0.95 as strongly and weakly supported (Devecchi et al., 2018).
3. RESULTS and DISCUSSION
The amplicon sizes of the trnL-trnF chloroplast and ITS genes from the 37 leaf samples were 957 and 845 bp, respectively. The results of the BLASTn analysis of the trnL-trnF and ITS regions showed that the E. longifolia leaf samples in the study had 99%–100% similarity with the Eurycoma sample in GenBank (Table 3). Thus, the BLASTn analysis results confirmed the identity of E. longifolia samples collected from the Sumatra mainland and Riau Islands used in this study.
No | Region | Similarity | Accession | % Similarity | % Query cover | Reference |
---|---|---|---|---|---|---|
1 | trnL-trnF | Eurycoma apiculata | GU593014 | 100.00 | 100 | Muellner-Riehl et al., 2016 |
E. longifolia | MH751519 | 99.79 | 100 | Ng et al., 2019 | ||
Simarouba versicolor | MG599400 | 99.16 | 99 | Devecchi et al., 2018 | ||
Perriera madagascariensis | GU593020 | 98.96 | 100 | Muellner-Riehl et al., 2016 | ||
Homalolepis morettii | MG599405 | 98.96 | 100 | Devecchi et al., 2018 | ||
2 | ITS | E. longifolia | MN715379 | 98.16 | 83 | Unpublished |
E. longifolia | MG643109 | 98.98 | 81 | Devecchi et al., 2018 | ||
Ailanthus altissima | OX327691 | 88.62 | 100 | Unpublished | ||
Simaba guianensis | MG643102 | 93.19 | 81 | Devecchi et al., 2018 | ||
Homalolepis cuneata | MG643083 | 93.18 | 81 | Devecchi et al., 2018 |
Sequencing attempts for some root or root chip products (GLPB and SAPB; Table 2) using the trnL-trnF region were unsuccessful despite three repetitions of the DNA extraction procedure with several modifications. The sequence readings generated for both products were highly unreadable, resulting in only four products being displayed in the amplification results using the trnL-trnF region (Table 4). These unreadable products were excluded from further analysis. GLPB and SAPB are products that physically consist of solid woody root or root chips and are sold as E. longifolia woody root. DNA extraction from wood is more challenging than that from fresh leaves (Liepelt et al., 2006; Swetha et al., 2014), thus potentially complex modifications in the DNA extraction process may be required to yield more pure genomic DNA, leading to higher success rates during sequencing. This is especially important if the wood material used in product manufacturing is not authentic E. longifolia but rather substitute species that are more abundant and easily accessible. To mask the characteristics of the substitute species, manufacturers often employ additional processes involving various chemicals that can affect the appearance of the substitute material (Psifidi et al., 2015). With such processes, DNA extraction becomes more difficult because of the need to eliminate numerous chemical compounds to obtain pure DNA for amplification in the subsequent stages. Modifications to the DNA extraction process are crucial for enhancing the purity and quality of genomic DNA extracted from wood products (Fatima et al., 2018; Finkeldey et al., 2010). These modifications include optimising the extraction protocol for wood samples, pre-treating samples to remove contaminants, employing physical disruption methods, and using specialised DNA purification kits (Dilley and Chapman, 2019; Rachmat et al., 2024; Rachmayanti et al., 2006; Verbylaitė et al., 2010).
Region | No. | Sample code | No. Accession | % Similarity | % Query cover | Species | References |
---|---|---|---|---|---|---|---|
trnL-trnF | 1 | APBC | NC_063303.1 | 98.89 | 99 | Dalbergia obtusifolia | Unpublished |
MH547571.1 | 100.00 | 95 | Dalbergia latifolia | Lee et al., 2019 | |||
MN251247.1 | 98.70 | 99 | Dalbergia cochinchinensis | Song et al., 2019 | |||
MN251245.1 | 98.70 | 99 | Dalbergia hupeana | Song et al., 2019 | |||
MN251242.1 | 98.70 | 99 | Dalbergia sissoo | Song et al., 2019 | |||
2 | GDPB | MG599402.1 | 92.65 | 99 | Simaba monophylla | Devecchi et al., 2018 | |
MG599406.1 | 92.48 | 99 | Simarouba glauca | Devecchi et al., 2018 | |||
MG599436.1 | 92.46 | 99 | Homalolepis subcymosa | Devecchi et al., 2018 | |||
MG599404.1 | 92.46 | 99 | Homalolepis glabra | Devecchi et al., 2018 | |||
MG599403.1 | 92.46 | 99 | Homalolepis pumila | Devecchi et al., 2018 | |||
3 | KPB | GU818102.1 | 98.83 | 100 | Vernonia gigantea | Pelser et al., 2010 | |
NC_061907.1 | 98.83 | 99 | Elephantopus scaber | Unpublished | |||
NC_053851.1 | 98.44 | 99 | Gymnanthemum amygdalinum | Zhou et al., 2021 | |||
MZ958830.1 | 98.05 | 99 | Cyanthillium cinereum | Unpublished | |||
OK040129.1 | 98.05 | 99 | C. cinereum | Unpublished | |||
4 | TCPB | MH751519.1 | 99.67 | 100 | E. longifolia | Ng et al., 2019 | |
MG599405.1 | 99.49 | 97 | Homalolepis morettii | Devecchi et al., 2018 | |||
MG599406.1 | 98.81 | 97 | S. glauca | Devecchi et al., 2018 | |||
MG599407.1 | 98.48 | 97 | Homalolepis suffruticosa | Devecchi et al., 2018 | |||
MG599416.1 | 98.80 | 97 | Simaba pubicarpa | Devecchi et al., 2018 | |||
ITS 5P-8P | 1 | KPB | JX564720.1 | 98.82 | 89 | E. scaber | Devecchi et al., 2018 |
MT914283.1 | 97.70 | 92 | Potentilla lineata | Liang, 2020 | |||
DQ813304.1 | 97.18 | 94 | E. scaber | Unpublished | |||
KP052671.1 | 97.28 | 92 | E. scaber | Unpublished | |||
JN407429.1 | 97.52 | 90 | E. scaber | Unpublished | |||
2 | SAPB | OX327710.1 | 85.89 | 100 | Ailanthus altissima | - | |
OX327691.1 | 85.89 | 100 | A. altissima | - | |||
DQ787413.1 | 86.38 | 93 | Simarouba amara | Unpublished | |||
MN082855.1 | 83.69 | 99 | Geijera paniculata | Duretto et al., 2020 | |||
MN715379.1 | 85.73 | 87 | E. longifolia | Unpublished | |||
3 | TCPB | MG643109.1 | 98.06 | 88 | E. longifolia | Devecchi et al., 2018 | |
MN715379.1 | 96.98 | 87 | E. longifolia | Unpublished | |||
MG643102.1 | 92.39 | 88 | Simaba guianensis | Devecchi et al., 2018 | |||
MG643083.1 | 92.24 | 88 | Homalolepis cuneata | Devecchi et al., 2018 | |||
MG643108.1 | 92.09 | 88 | Hannoa chlorantha | Devecchi et al., 2018 | |||
4 | GLPB UPPER | CP115837.1 | 100.00 | 99 | Klebsiella pneumoniae | Unpublished | |
CP115930.1 | 100.00 | 99 | K. pneumoniae subsp. pneumoniae | Unpublished | |||
CP095426.1 | 100.00 | 99 | K. pneumoniae | Tang et al., 2023 | |||
CP095428.1 | 100.00 | 99 | K. pneumoniae | Tang et al., 2023 | |||
CP115714.1 | 100.00 | 99 | K. pneumoniae | Unpublished |
The trnL-trnF BLASTn sequence of E. longifolia products showed that only the TCPB (root chips) product was similar to E. longifolia MH751519.1 (99.67%). However, the GDPB product was similar to Simaba monophylla MG599402.1 (92.65%), Simarouba glauca MG599406.1 (92.48%), and Homalolepis species such as Homalolepis subcymosa MG599436.1 (92.46%), Homalolepis glabra MG599404.1 (92.46%), Homalolepis pumila MG599403.1 (92.46%), which belongs to the same family as E. longifolia, namely Simaroubaceae (Table 4). The two products, TCPB and GDPB, were similar to E. longifolia and the Simaroubaceae family sequences from GenBank in the trnL-trnF sequence, indicating that these products were most likely derived from E. longifolia. Meanwhile, the other root powder products (APBC and KPB) had dissimilarities with the sequences of E. longifolia or other species in the Simaroubaceae family in GenBank, indicating that the products may not be derived from E. longifolia.
The ITS region amplification was only successful for four products: TCPB, GLPB, KPB, and SAPB. However, the two remaining E. longifolia herbal products, GDPB and APBC, failed to be amplified, resulting in messy and unreadable sequences. The results of the ITS BLAST sequencing of E. longifolia products showed that only TCPB was similar to E. longifolia MG643109.1 (98.06%) and MN715379.1 (96.98%) from GenBank. In addition, the TCPB product was similar to Simaba guianensis MG643102.1 (92.39%), Homalolepis cuneata MG643083.1 (92.24%), and Hannoa chlorantha MG643108.1 (92.09%) which belong to the Simaroubaceae family (Table 4). The other products (KPB, SAPB, and GLPB) were dissimilar to the E. longifolia GenBank sequence. This pattern indicates that the TCPB product was most likely derived from E. longifolia and other products were derived from other species that are not found in the Simaroubaceae family. ITS analysis in this study also indicates the identification of Klebsiella pneumonia bacteria in GLPB (wooden cup product), urging consumers to exercise caution in the purchase and use of wooden cups as consumable items, given their potential to host such bacteria.
Of the six products analysed, only TCPB (an herbal tea product) was identified as an E. longifolia derivative based on the trnL-trnF and ITS regions, whereas the other products were derived from different plant species, indicating the potential for product mislabelling or falsification. Therefore, the DNA markers used in this study (trnL-trnF and ITS regions) were effective in tracing the authenticity of the product.
Compared to fresh plant DNA, neither the trnL-trnF nor the ITS regions were successfully amplified for all products. This inability to achieve successful DNA amplification may be attributed to DNA degradation during the manufacturing process. Various secondary metabolites, including polysaccharides, glycoproteins, polyphenolic compounds, and pigments, originating from raw materials in diverse plant tissues and organs, have been established as factors that impede DNA extraction, amplification, and sequencing processes (Zahra et al., 2016). Additionally, the degradation of DNA at primer annealing sites may contribute to unsuccessful amplification of the targeted DNA barcode regions, leading to reaction failure (Newmaster et al., 2013).
Various plant species which were mixed and packaged in powder form, chips, or fine particles also contributed to amplification failure using the two selected DNA regions or products that failed to be amplified with the previous primer pairs, which had a sequence length of approximately 900 base pairs, and the use of a new primer pair for both targeted regions may be necessary to amplify shorter lengths (Little and Jeanson, 2013). Similar outcomes were observed in a previous study that had successful amplification using a shorter fragment of 500bp (Abubakar et al., 2018). Several studies have suggested that shorter DNA barcodes are more efficacious for the identification and authentication of herbal products because of their enhanced amplification efficiency (Little, 2014; Little and Jeanson, 2013).
Both products, presented as E. longifolia herbal tea with the code TCPB, were conclusively identified as E. longifolia through both the trnL-trnF region and ITS. This unequivocally establishes that the tea in question was sourced from the botanical elements of E. longifolia. The raw material for tea, comprising leaves, is notably more abundant than the E. longifolia products derived from the roots. E. longifolia tea products, distinguished by their leaf utilisation, have emerged as environmentally friendly options. This is primarily because of the sustainable nature of leaf harvesting, setting it apart from products relying on root extraction, an inherently destructive process that leads to the demise of the plant. Consequently, E. longifolia tea products derived from leaves have heightened potential for authenticity when juxtaposed with their counterparts that utilise root organs or stems.
The claim that the wooden cup originated from the root of E. longifolia remains unsubstantiated, as evidenced by both the trnL-trnF marker and ITS analysis. The cup, which measures 9 cm in diameter, requires raw material from E. longifolia roots with diameters exceeding 9 cm. Morphologically and botanically, the assertion that the cup is derived from the roots of E. longifolia appears exaggerated. Considering the morphological aspects and botanical characteristics observed during field surveys on the Sumatran mainland and the Riau Islands, E. longifolia is a small tree with an average diameter of less than 15 cm and a height not exceeding 15 m. The production of a wooden cup with a 9 cm diameter would necessitate an extensive supply of large E. longifolia roots, which is inconsistent with the abundance of E. longifolia individuals in their natural habitat in Sumatra. Molecular identification of the wooden cup product revealed that the substituted wood used in its construction belongs to a different genus within the same family. From the perspectives of availability, rarity, and conservation status, the substituted type was more accessible and abundant.
The claim regarding E. longifolia root tea in powdered form (APBC) has also been identified as not originating from the E. longifolia plant but rather from the wood organ of the rosewood plant (Dalbergia). The falsification of the powdered form is particularly straightforward, given that the powder lacks distinctive morphological features. The introduction of a bitter taste into the product can be artificially achieved, or by natural substitution of ingredients, such as those found in various species within the Dalbergia genus, which already possess a naturally bitter taste.
The claim that the herbal capsules contained E. longifolia in powdered form was disproved, as confirmed by both the trnL-trnF region and ITS analysis. Both regions recognised the product as potentially derived from different plant species, including Vernonia gigantea, Elephantopus scaber, Chanthilium cinereum, Gymnanthemum amugdalium, and Potentilla cireata. DNA marker-based identification suggested that the herbal capsules were a composite of different plant species. In Indonesia, the identified plant species constituting the herbal capsules are commonly used by the public as traditional herbal medicines or supplements. These plants are abundant on forest floors because of their herbaceous nature, and some have been cultivated intensively in home gardens or on larger scales. Despite being derived from plant species with similar herbal properties, labelling the product as E. longifolia is misleading and constitutes a fraudulent practice. Labelling products originating from E. longifolia leads to a significantly higher market value, creating a deceptive economic advantage, as products labelled based on factual information about actual plant ingredients may have a lower market value.
Similarly, the claim regarding the herbal tea made from E. longifolia roots, presented in the form of thin root shavings, lacks support from the molecular identification conducted in this study. The results of our molecular analysis indicated that the source of the raw material for the product resembled Alianthus altissima, Simarouba amara, and Geijera paniculata. Additionally, the product is suggested to be a composite of several types of raw materials, all of which are known for their herbal properties in Indonesia.
Following the sequence identity results of all samples, these results were verified by phylogenetic analysis. The results of the phylogenetic tree reconstruction using the trnL-trnF gene (Fig. 1) with Castela erecta (GU593013.1) as the outgroup (Clayton et al., 2010, unpublished) indicated that TCPB clustered together with the E. longifolia leaf sample sequence, with a branch support of 0.9298. Additionally, the construction of trees in Mr. Bayes using the ITS gene (Fig. 2) with Castela emoryi (MF963835.1) as the outgroup (Thornhill et al., 2017) revealed that TCPB clustered with E. longifolia leaf samples and E. longifolia (MG643109.1) sequences from GenBank, with a branch support of 0.5944. Conversely, other product samples, namely KPB, GDPB, GLPB, SAPB, and APBC, were not grouped together with the E. longifolia leaf samples used in this study or E. longifolia in the NCBI database.
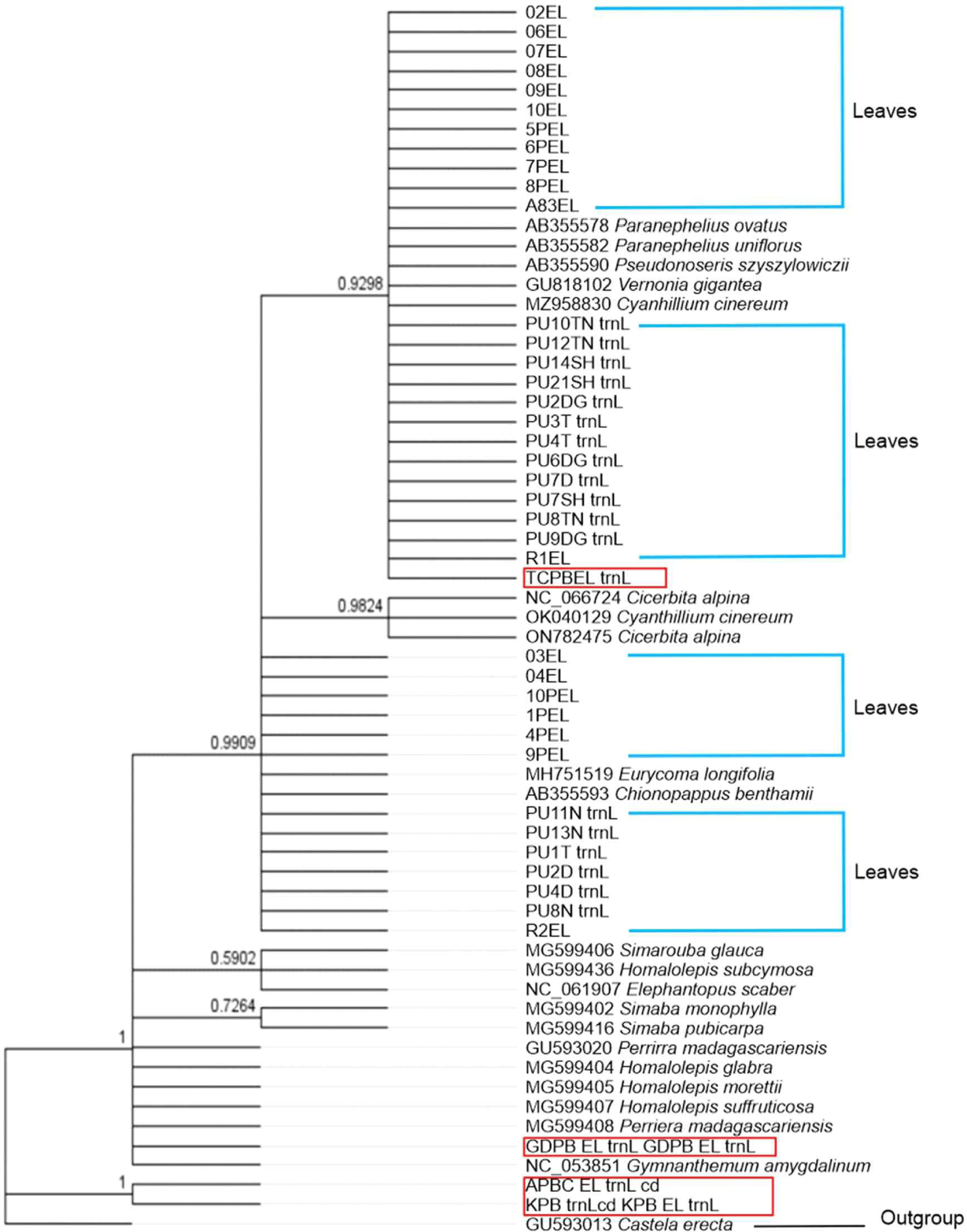
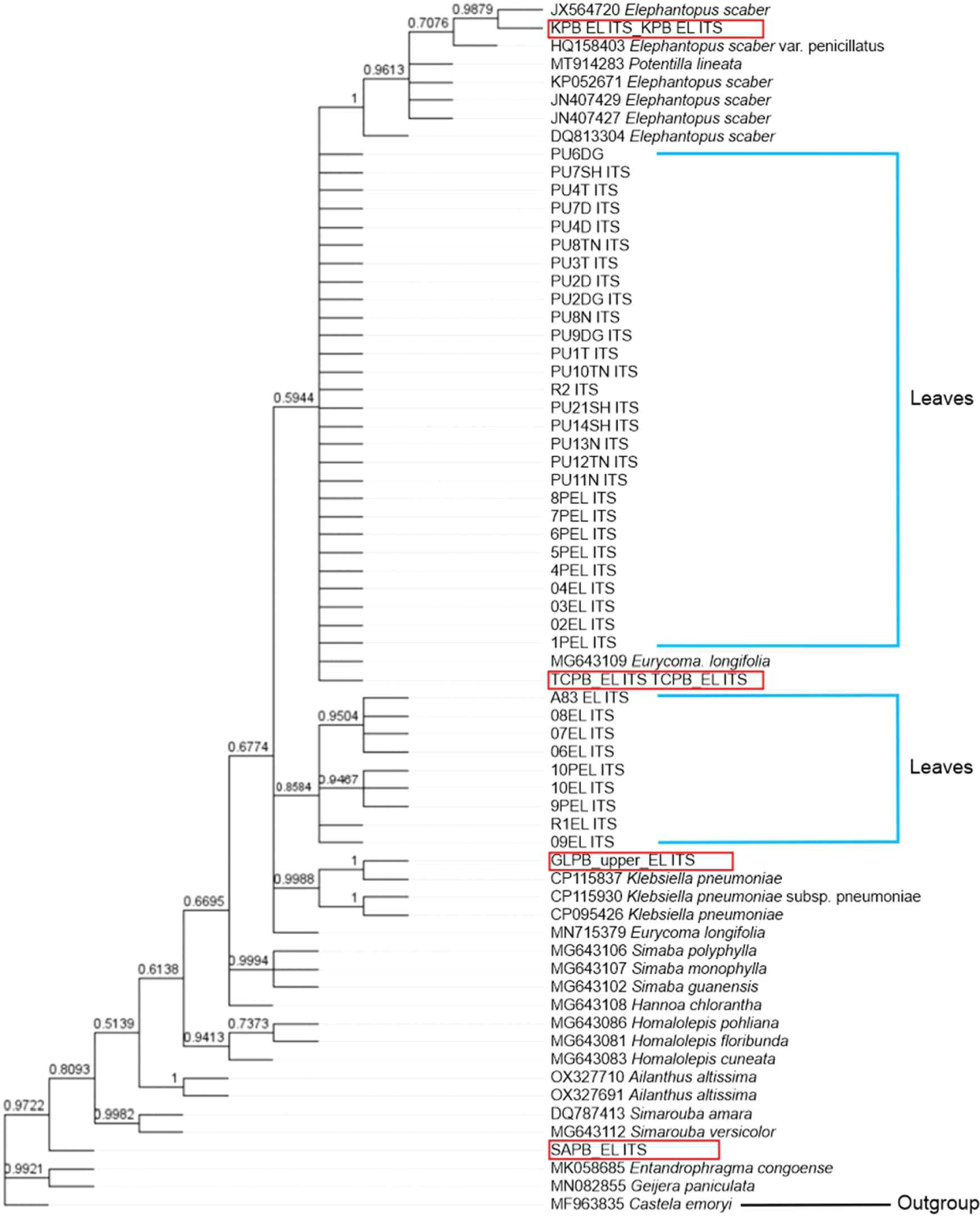
Using trnL-trnF primers, BLASTn analysis revealed that among those tested, only the TCPB sample exhibited 100% similarity to E. longifolia species (Ng et al., 2019; MH751519). BLASTn also identified other TCPB species (Table 4), indicating the possibility of adulteration with other substitutes or products. The presence of substituted products may result in barcodes that differ from the labels assigned to the tested herbal products (Abubakar et al., 2018; Newmaster et al., 2013). As shown in Fig. 1, Bayesian analysis indicated that among the tested herbal products samples, only TCPB formed a monophyletic clade that was strongly supported (0.9909) by the E. longifolia reference sequence derived from this study. 02EL, 06 EL, 07 EL, 08 EL, 09EL, 10EL, 5PEL, 6 PEL, 7 PEL, A83EL, PU 10TN, PU12TN, PU14 SH, PU21 SH, PU2DF, PU3T, PU4T, PU6DG, PU7D, PU7SH, PU8TN, PU9DG, R1EL and other sequences of E. longifolia obtained from the GenBank repository (MH751519.1). This study demonstrated the authenticity of the tested herbal product samples. However, several species were clustered in the same clade: Paranephelius ovatus AB355578, Paranephelius uniflorus AB355582, Pseudonoseris szyszylowiczii AB355590, V. gigantea GU818102, C. cinereum MZ958830. These findings suggest that while trnL-trnF can be adequately amplified across a diverse range of plants, its variability may not be sufficient for species discrimination, as suggested by de Groot et al. (2011).
BLASTn analysis of the ITS barcoding regions indicated that only one of the tested herbal product samples (TCPB) exhibited a > 88% match with E. longifolia (Table 4). Nevertheless, the BLASTn results revealed that TCPB harboured a DNA barcode from a different species, contrary to the labelled sample information. TCPB also has 87%–88% similarity with S. guianensis MG643102.1, H. cuneata MG643083.1, and H. chlorantha MG643108.1. Referring to Fig. 2, the Bayesian analysis of the ITS region in E. longifolia revealed that one of the tested HP samples (TCPB) formed a cluster with the E. longifolia reference sequence established in the current study (PU6DG, PU7SH, PU4T, PU7D, PU4D, PU8TN, PU3T, PU2D, PU2DG, PU8N, PU9DG, PU1T, PU10TN, R2, PU21SH, PU14SH, PU13N, PU12TN, PU11N, 8PEL, 7PEL, 6PEL, 5PEL, 4PEL, 04, 03, 02, 1 PEL) and other E. longifolia sequences retrieved from the GenBank database (MG643109). This result indicated that TCPB did contained E. longifolia. Other tested HP samples were positioned outside the clade of the reference samples, suggesting that these herbal medicinal products (HMPs) harboured plant species that were different from those declared on their packaging labels.
The Bayesian results for both trnL-trnF and ITS demonstrated that the other five HP-tested samples clustered not with E. longifolia but with S. glauca, Homalolepis subcymora, E. scaber, and Simaba. S. glauca is a flowering tree that is native to Florida, South America, and the Caribbean. The tree is well suited to warm, humid, and tropical regions such as Indonesia. E. scaber (locally known as tapak liman) is a plant that can be easily found in Indonesia, including Southeast Celebes (Choi and Hwang, 2005).
The Bayesian analysis results aligned with those of the BLASTn, demonstrating that the tested HPs containing E. longifolia clustered together, while those lacking E. longifolia, contrary to the labelled information, formed a distinct clade. Thus, both the BLASTn and Bayesian tree methods effectively differentiated between HMPs containing E. longifolia and those without HMPs (Zhao et al., 2013).
In the context of DNA barcoding in this study, only one of the six tested HPs (TCPB) was identified as authentic based on the criteria from BLASTn and Bayesian tree analyses, indicating possible counterfeiting or adulteration in five of the tested HPs. As emphasised by Zhao et al. (2013), any new method for identifying traditional medicines should prioritise accuracy, digitisation, repeatability, simplicity, and practicality. Compared with traditional methods, holds significant importance because of its ability to identify species in various forms, such as dried herbs, leaves, and roots, without being restricted by morphological characteristics.
Our study suggests that the trnL-trnF and ITS regions are promising DNA barcodes for authenticating E. longifolia HMPs because of their short sizes. An ideal marker should be short enough to amplify degraded DNA, produce high-quality sequences, and possess robust variability for sample discrimination (Li et al., 2015; Vijayan and Tsou, 2010). The ITS region, confirmed by various researchers, including (Gao et al., 2010; Han et al., 2016), stands out as one of the best DNA barcode regions for the identification and authentication of medicinal plants. Chen et al. (2010) conducted an extensive study to identify the ITS2 region as the most suitable for medicinal plant identification, with a success rate of 92.7% in 6,600 samples and closely related species. In another study by He et al. (2012), which analysed 109 samples with five DNA barcode regions, the ITS and ITS2 regions were found to be the most effective among the tested regions.
This study marks the pioneering use of DNA barcode molecular identification technology to identify E. longifolia and its adulterants, thereby extending the application of trnL-trnF and ITS sequences to medicinal plants, particularly in Indonesia. Mislabelling observed in certain products constitutes a form of fraud, as consumers rely on product labels for expected benefits. Olivar et al. (2016) exemplified this by documenting instances where Vitex negundo (Lamiaceae) was substituted with contaminants, such as Moringa olifeira (Moringaceae) and Mormodica charantia (Cucurbitaceae), despite being medicinal plants, as revealed through DNA barcoding. Similar occurrences emerged in the current study, where products labelled as E. longifolia actually contained other species, such as Homalolepis, Elephantopus, and Simaba, potentially harbouring different natural compounds compared to E. longifolia. Notably, the identification of Dalbergia sp. as a substituted species in a product claimed to be derived from the roots of E. longifolia is intriguing, given its classification into a different plant family and its lack of known aphrodisiac benefits. This indirect form of adulteration, which involves the use of abundant and easily accessible materials to maximise profits, has been documented by Satheeshkumar et al. (2016).
Authenticating commercial products with incorrect species poses challenges, particularly when their forms are altered, such as powdered capsules or dried plant materials for tea. These alterations compromised the morphological characteristics and diminished the aroma due to volatile evaporation. Nevertheless, utilising an appropriate DNA extraction method capable of yielding high-purity DNA allows for amplification even from minute DNA samples. The Bayesian tree analysis in the present study effectively distinguished between E. longifolia and the other species.
4. CONCLUSIONS
DNA barcoding is a rapid and reliable method for authenticating herbal products derived from E. longifolia. This study showed that herbal products of E. longifolia available in the Indonesian market are subject to adulteration or substitution potentially stemming from inaccuracies in species identification. This study also strongly advocates the prompt integration of DNA barcoding as a mechanism for identifying instances of species adulteration to improve the production of high-quality herbal products. Consequently, DNA barcoding should be included in the quality control measures of government certification bodies to facilitate a comprehensive evaluation of product authenticity and to ensure the safety of the herbal products consumed. This will provide nuanced insights into the safety and efficacy of these products.