1. INTRODUCTION
A biofilm is a collective microbial community surrounded by an extracellular matrix consisting mainly of polysaccharides, proteins, and extracellular DNA (Flemming et al., 2023). Biofilms inhibit physical removal, such as washing, and the diffusion of disinfectants. Through these mechanisms, biofilms protect cells and help them withstand various stresses, including antibiotic treatment (Ham et al., 2023; Park et al., 2015; Shree et al., 2023). At the same time as the cells on the surface of the biofilm consume nutrients, the cells inside the biofilm have a stress physiology with limited nutrient supply, which also confers resistance to various bactericidal stresses. Most bacteria grow by forming biofilms in nature, so controlling biofilm formation is important for maintaining hygiene and treating infections by eliminating bacterial contamination (Liu et al., 2023). In infectious diseases caused by biofilm-forming cells, the biofilm increases their survivability over planktonic cells under antibiotic treatment, leading to disease recurrence and chronic disease (Su et al., 2022). Continuous exposure to antibiotics for chronic infectious diseases provides an opportunity for the emergence of resistant strains, making the treatment of microbial infectious diseases more difficult. Therefore, there is a need for efficient methods to remove bacterial biofilms.
Plant extracts provide a wide range of bioactive substances that exhibit antifungal effects (Na and Kim, 2022; Yoon and Kim, 2021, 2023) and antibiotic effects (Ham et al., 2020); eliminate persister cells (Kim and Kim, 2024); and alleviate inflammation, diabetes, and obesity in humans (Myeong et al., 2023). They also exhibit antioxidant effects (Huh et al., 2022; Yang et al., 2022) and antitermite effects (Adfa et al., 2023; Zalsabila et al., 2024). Licorice is a herb that has been widely used for a long time worldwide (Yan et al., 2023). Glycyrrhizin, a major component of licorice, is approximately 50 times sweeter than sugar (Kinghora et al., 1986; Lee, 2018). It also has many beneficial biological properties, such as anticancer activity (Tang et al., 2015; Wang et al., 2023), anti-inflammatory activity (Leite et al., 2022; Wang et al., 2019, 2021; Yang et al., 2017), antiviral activity (Wang et al., 2015; Zuo et al., 2023), and antimicrobial activity (Wang et al., 2015). Furthermore, licorice can effectively prevent tooth decay (Ahn et al., 2015; Messier et al., 2012). The beneficial effect of licorice on oral health is based on its bactericidal effect on oral bacteria. In prior studies, lollipops prepared using licorice have been used to prevent tooth decay (Almaz et al., 2017; Brand et al., 2009; Hu et al., 2011). However, it is important to note that overconsumption of licorice can lead to a variety of side effects, such as pseudohyperaldosteronism (Sabbadin et al., 2024). Ham and Kim (2019) found that glycyrrhetinic acid, a pentacyclic triterpenoid derivative of glycyrrhizin, inhibited biofilm formation in Streptococcus mutans without exhibiting bactericidal activity. Moreover, in previous studies, licorice extract inhibited biofilm formation in various bacteria, including Staphylococcus aureus and Pseudomonas aeruginosa (Peng et al., 2017; Rohinishree and Negi, 2016). However, the effect of licorice extract on biofilm formation in Aeromonas hydrophila and Yersinia enterocolitica has not been thoroughly investigated.
A. hydrophila is an opportunistic pathogenic bacterium that causes diseases in freshwater fishes and amphibians (Semwal et al., 2023). In humans, it generally does not cause illness in healthy individuals; however, it may cause gastroenteritis in immunocompromised individuals or young children. A. hydrophila produces biofilms to be naturally retained in the host. A. hydrophila in biofilms is more resistant to cold oxygen plasma and ethanol than planktonic cells (Jahid et al., 2014; Park et al., 2015).
Y. enterocolitica is a pathogenic bacterium that causes food poisoning, known as yersiniosis, in humans (Robins-Browne, 2012). In healthy individuals, Y. enterocolitica may cause mild symptoms of gastroenteritis that can be naturally healed. However, the incidence of yersiniosis has been mainly reported in children under 5 years of age (Marimon et al., 2017). Y. enterocolitica can become resistant to ethanol and sodium hypochlorite by producing biofilms (Ham et al., 2023; Park et al., 2015). Biofilm formation in Y. enterocolitica could be inhibited by edible plant extracts (Ham and Kim, 2022).
In this study, we assessed the effect of licorice extract on biofilm formation in A. hydrophila and Y. enterocolitica. The strain-specific inhibitory effect of licorice on biofilm formation and its cell growth inhibitory effect suggest its potential for therapeutic and hygienic bacterial control and lay out a foundation for strain-specific biofilm formation research.
2. MATERIALS and METHODS
A. hydrophila was obtained from the Research Institute of LG Household & Health Care (Daejeon, Korea). Y. enterocolitica (KCCM 41657) was obtained from the Korean Culture Center of Microorganisms (Seoul, Korea). Dried licorice (Glycyrrhizae Radix) was purchased from Jiwoondang Herbal Medicine Store (Seoul, Korea). Glycyrrhizin (catalog number: G0150) was purchased from Tokyo Chemical Industry (Tokyo, Japan). Glycyrrhizin was dissolved in 50% ethanol before use. Luria–Bertani (LB) medium was prepared with 20 g/L Difco LB broth, Miller (ref: 244520, Becton, Dickinson, and Company, Franklin Lakes, NJ, USA). Tryptic soy broth with yeast extract (TSBY) medium was prepared with 30 g/L tryptic soy broth (ref: 211825, Becton, Dickinson, and Company) and 6 g/L yeast extract (ref: 212750, Becton, Dickinson, and Company). Tryptone yeast extract (TYE) medium was prepared with 10 g/L tryptone (ref: 211705, Becton, Dickinson, and Company) and 5 g/L yeast extract. M63G medium was prepared with 200 mL/L 5 × minimal M63 medium [15 g/L KH2PO4, 35 g/L K2HPO4, 10 g/L (NH4)2SO4, and 2.5 mg/L of FeSO4], 1 mL 20% glucose, and 0.1 mL 20% MgSO4 and filled with water.
Dried licorice was ground to a size of ≤ 1 mm. In total, 10 g of ground licorice was mixed with 50 mL of extract solvent and incubated at 50°C for 3 h with periodic shaking every 30 min. The extract solvent consisted of water and 20%–95% ethanol. After extraction, licorice residue was removed by filtration with Whatman filter paper, grade 1 (catalog number: 1002 110, Cytiva, Sigma-Aldrich, Seoul, Korea). Ethanol in the filtered extract was evaporated using a reduced-pressure rotary evaporator (RV10, IKA® Korea, Seoul, Korea). The original volume of the pre-concentrate extract was restored by adding distilled water. Insoluble material was removed by centrifugation at 16,810 ×g for 20 min and another filtration with a syringe filter (catalog number: FJ25ASCCA002DL01, ABLUO syringe filter 25 mm, GVS Kore, Namyangju, Korea). The final extracts were stored at –80°C.
For biofilm formation, A. hydrophila cells stored at –80°C were streaked on LB agar plates and incubated at 30°C for 1 day to obtain a single colony. The single colony was inoculated into LB medium and incubated at 30°C for 1 day. The precultured cells were inoculated into each well of 96-well polyvinyl chloride (PVC) microplates (catalog number: 2797, Corning Korea Company, Seoul, Korea) containing 100 μL TSBY medium. The inoculated cell density was 0.05 at Abs600. After incubation at 30°C for 1 day, the amount of biofilm formed was measured using 1% crystal violet, as described in the subsequent section.
Y. enterocolitica biofilms were produced according to a previously described method (Kim et al., 2008). In brief, cells stored at –80°C were streaked on LB agar plates and incubated at 26°C for 2 days to obtain a single colony. The single colony was inoculated into TYE medium and incubated at 26°C for 1 day. The precultured cells were inoculated into each well of 96-well PVC microplates containing 100 μL M63G medium. The inoculated cell density was 0.05 at Abs600. After incubation at 26°C for 1 day, the amount of biofilm formed was measured using 1% crystal violet, as described in the subsequent section.
The effect of licorice extracts or glycyrrhizin on biofilm formation was measured by adding the samples to the wells of 96-well PVC microplates before cell inoculation. The inhibition of biofilm formation of two strains by the addition of a sample is assessed by comparing the amount of biofilm formed with the addition of the sample to the amount of biofilm formed without the addition of the sample.
After 24 h of biofilm formation in 96-well PVC microplates, the optical density of each well at 595 nm was measured using an Opsys MR microplate reader (Dynex Technologies, Chantilly, VA, USA). Subsequently, quantitative analysis of the biofilms was performed using the crystal violet assay as described previously (Kim et al., 2008), with a slight modification: the incubation time was revised from 20 to 15 min. The optical density of the residual crystal violet was also measured at 595 nm using an Opsys MR microplate reader (Dynex Technologies).
3. RESULTS and DISCUSSION
From a library of 140 methanolic extracts of edible plants, we screened extracts that inhibited biofilm formation of microorganisms (data not shown). Among them, the methanolic extract of licorice inhibited biofilm formation of Y. enterocolitica by 66.7% while inhibiting cell growth by only 17.1% (Table 1). The methanol extract of licorice had no effect on cell growth against A. hydrophila, but enhanced its biofilm formation.
Although methanol offers advantages in the preparation of extracts, its use is limited by safety concerns. For further experiments, the extraction solvent was changed to ethanol. The solids yield of licorice extract as a function of ethanol concentration showed no significant change in solids yield with increasing ethanol concentration up to 70%, which was around 25%, but above 70%, the solids yield decreased with increasing ethanol concentration in a previous study (Ham and Kim, 2019). Ethanol is classified as a hydrophilic solvent; however, high concentrations of ethanol reduce the solubility of proteins, lipids, and polysaccharides and even cause denaturation on the latter (Xiong et al., 2024; Yu et al., 2024). If the outer layer of the particles to be extracted is first denatured by the solvent, it will hinder the penetration of the extraction solvent and make it more difficult to extract the inside of the particles, resulting in lower extraction yields and possibly changing the chemical composition of the extract. Therefore, the concentration of ethanol required for effective sterilization is approximately 70%. Lower concentrations of ethanol have been proposed to maximize the effectiveness of biofilm removal while eliminating bacteria (Park et al., 2015).
The ability of licorice extracts to inhibit biofilm formation in A. hydrophila and Y. enterocolitica as a function of ethanol concentration in the extraction solvent (Fig. 1). Licorice extracts prepared in 50% ethanol maximally inhibited biofilm formation in A. hydrophila by 55% [Fig. 1(a)]. When the ethanol concentration was decreased or increased beyond 50%, the greater the change in concentration, the less effective was the inhibition of biofilm formation. However, the cell growth of A. hydrophila was not affected by the licorice extract regardless of the ethanol concentration [Fig. 1(b)].
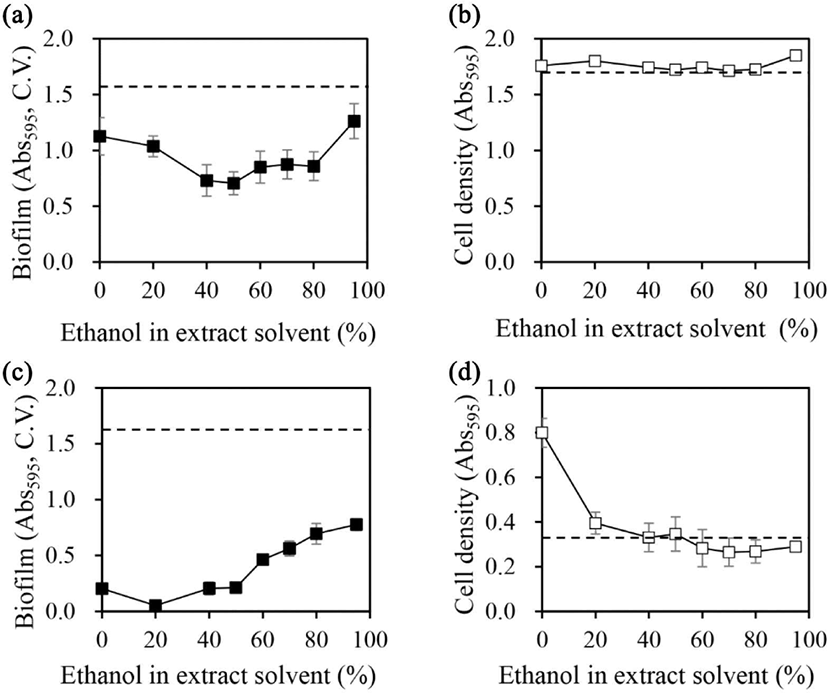
In Y. enterocolitica, licorice extracts prepared in 20% ethanol caused up to 97% inhibition of biofilm formation. Moreover, the inhibitory effect on biofilm formation decreased as the ethanol concentration increased, with licorice extracts prepared in 95% ethanol causing 52% inhibition of biofilm formation [Fig. 1(c)]. The water extract (prepared in 0% ethanol) exhibited a lower inhibitory effect than the 20% ethanol extract. Cellular growth of Y. enterocolitica was promoted by water extract [Fig. 1(d)], which is consistent with previous findings of the growth promotion on S. mutans (Ham and Kim, 2019, 2023). However, when extracts were prepared in more than 40% ethanol, the cell growth-promoting effect disappeared. Licorice extracts prepared in up to 95% ethanol did not inhibit cell growth.
When calculating the amount of biofilm formation per cell, for the licorice extract made with 0% ethanol solvent, which was a water extract, the biofilm production per cell was reduced due to the increased cell growth. For extracts made with more than 40% ethanol as extraction solvent, the inhibitory effect on biofilm formation per cell was partially offset by a partial increase in biofilm formation, since the effect of stimulating cell growth disappeared. However, if the biofilm-forming capacity per cell of Y. enterocolitica was calculated using the same method in the case of cell cultures without sample treatment, the biofilm-forming capacity of Y. enterocolitica was estimated to be almost twice that of the highest biofilm-forming extract, even when compared with the highest biofilm-forming extract, so that it can be concluded that all extracts inhibited the biofilm-forming physiology of the cells, regardless of the ethanol concentration in the extraction solvent.
Compared with our previous findings on the effects of licorice extract on S. mutans (Ham and Kim, 2019), the present findings suggest that the effects of ethanol concentrations used in the preparation of licorice extracts on biofilm formation and cell growth are species-specific. We propose that this species specificity is attributed to the different biological mechanisms and types of organic substances that contribute to biofilm formation in different bacterial species, in addition to the variations in compound contents in licorice extracts according to ethanol concentrations.
Licorice extracts that prepared in 50% and 20% ethanol presented maximal biofilm inhibition in A. hydrophila and Y. enterocolitica, respectively (Fig. 1). The effects of the prepared extracts on the biofilm formation ability and cell growth of A. hydrophila and Y. enterocolitica were evaluated (Fig. 2).
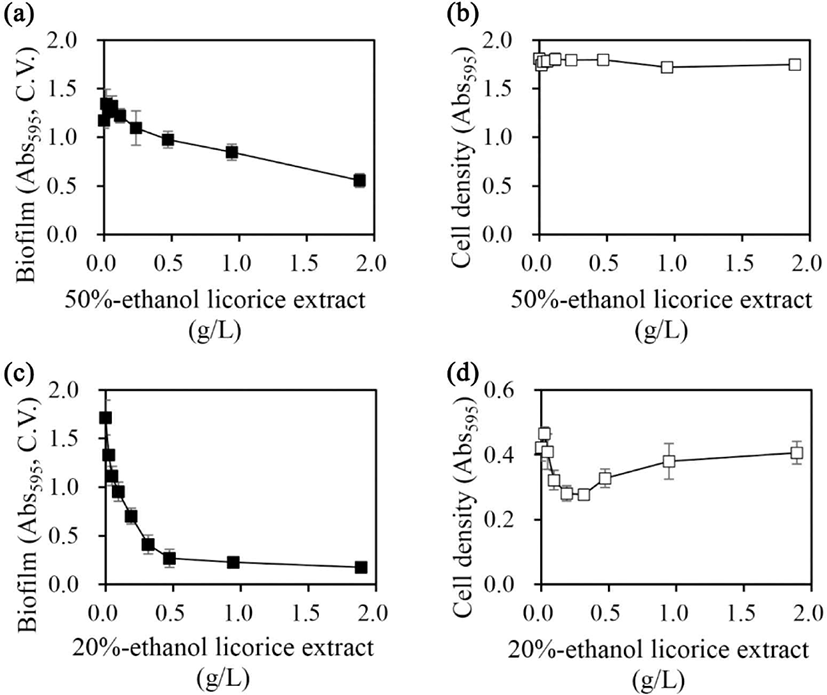
In A. hydrophila, biofilm formation was inhibited with an increase in the concentration of the 50% ethanol extract, with 53% inhibition noted at a concentration of 1.9 g/L [Fig. 2(a)]. However, cell growth was not affected at any concentration [Fig. 2(b)].
In Y. enterocolitica, the inhibitory effect of licorice extract prepared in 20% ethanol on biofilm formation increased in a concentration-dependent manner with an increase in concentrations up to 0.47 g/L, with 84% inhibition noted at 0.47 g/L [Fig. 2(c)]. However, the inhibitory effect did not increase further when extract concentrations were applied beyond 0.47 g/L. Cell growth was inhibited with an increase in concentration up to 0.32 g/L, with 34% growth inhibition noted at 0.32 g/L [Fig. 2(d)]. At concentrations higher than 0.32 g/L, the cell growth began to recover. No cell growth inhibition was noted up to 1.9 g/L.
The inhibition of biofilm formation inhibits the adhesion of cells to solid surfaces, making it easier to remove them by physical methods, such as washing. This is an easy way to remove microorganisms from an infected area. It also facilitates the elimination of bacterial contamination. The simplest way to inhibit biofilm formation is to inhibit cell growth. The inhibition of cell growth reduces the number of cells and the amount of biomass produced, in turn reducing the biofilms formation. However, the inhibition of cell growth can serve as a selective factor that can facilitate the emergence of resistant strains. Continuous treatment with a compound that inhibits bacterial growth can act as a selective force for mutations that confer resistance to the bactericidal action of the compound, thereby facilitating the emergence of resistant strains. The emergence of these resistant strains can neutralize the antibacterial activity of the compound; therefore, a bacterial elimination method other than sterilization is required. One such method involves the inhibition of biofilm formation without inhibiting cell growth. This approach would not inhibit cell growth and would thus not confer selectivity to facilitate the emergence of resistant bacteria. Moreover, it would facilitate the removal of bacterial cells through physical methods. The ethanolic licorice extract proposed in this study could inhibit biofilm formation without inhibiting the cell growth of A. hydrophila, a strain that causes infectious disease in fishes. It could also inhibit biofilm formation in Y. enterocolitica, a strain pathogenic to humans, exhibiting a very strong inhibitory effect on biofilm formation despite having a lesser inhibitory effect on cell growth (Fig. 1).
Glycyrrhizin is the main active compound in licorice (Gibson, 1978). In a previous study, glycyrrhizin exhibited a potent inhibitory effect on biofilm formation in S. mutans (Ham and Kim, 2019). In this study, the effects of glycyrrhizin on the biofilm formation ability and cell growth of A. hydrophila and Y. enterocolitica were evaluated (Fig. 3).
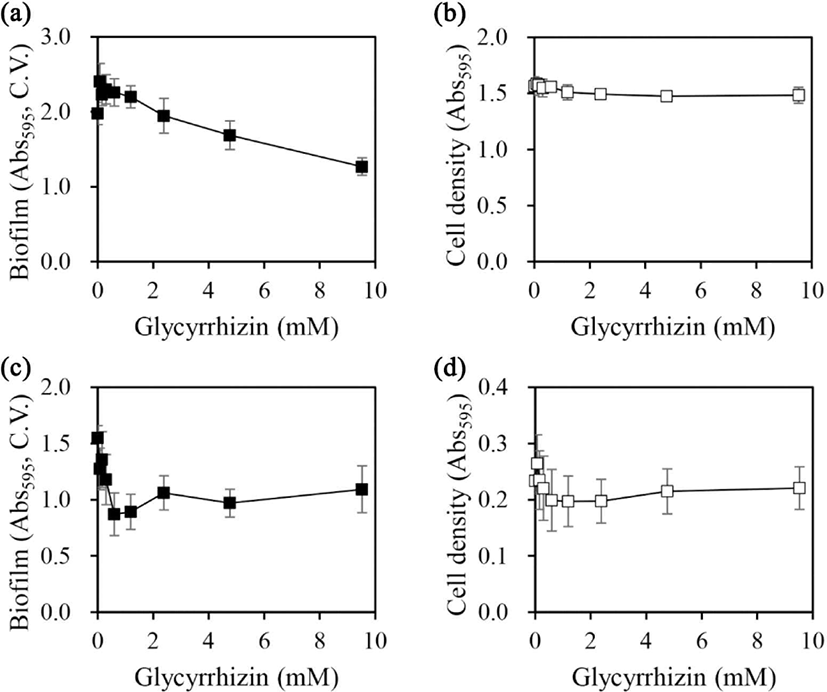
In A. hydrophila, biofilm formation was inhibited in a concentration-dependent manner at glycyrrhizin concentrations up to 9.5 mM; however, only 36% inhibition was observed at 9.5 mM [Fig. 3(a)]. On the other hand, cell growth was not affected at any of the concentrations tested [Fig. 3(b)]. The contribution of glycyrrhizin to the inhibitory effect of licorice extract on A. hydrophila biofilm formation was also assessed. Licorice extract prepared in 50% ethanol caused 53% inhibition at a concentration of 1.9 g/L [Fig. 2(a)]. The glycyrrhizin concentration in licorice extract with 50% ethanol was 15.3% (Ham and Kim, 2019), which corresponds to a glycyrrhizin concentration of 0.29 g/L (0.35 mM) in 1.9 g/L licorice extract. Moreover, the amount of biofilm formed on treatment with 0.35 mM glycyrrhizin was higher than that without glycyrrhizin treatment but slightly lower than that on treatment with 0.075 mM glycyrrhizin (the concentration at which the maximum biofilm formation was observed).
The trends of changes in the inhibition of biofilm formation and the absence of changes in cell growth on treatment with increasing concentrations of glycyrrhizin were very similar to those on treatment with licorice extract prepared in 50% ethanol. Nevertheless, our findings suggest that glycyrrhizin contributes in part to the inhibitory effect of licorice extract on A. hydrophila biofilm formation and additional active constituents might exist in the extract to coordinate the biofilm inhibition.
In Y. enterocolitica, biofilm formation was inhibited in a concentration-dependent manner at glycyrrhizin concentrations up to 0.6 mM; however, no further inhibition was observed at higher concentrations [Fig. 3(c)]. Regarding cell growth, a slight decrease in the mean value was observed up to a concentration of 0.6 mM; however, the change in cell growth was not significant [Fig. 3(d)]. The contribution of glycyrrhizin to the inhibitory effect of licorice extract on Y. enterocolitica biofilm formation was also assessed. Licorice extract prepared in 20% ethanol caused 84% inhibition of Y. enterocolitica biofilm formation at a concentration of 0.47 g/L [Fig. 2(c)]. The glycyrrhizin concentration in licorice extract with 20% ethanol was 13.3% (Ham and Kim, 2019). Hence, 0.47 g/L of this extract contained 0.063 g/L (0.076 mM) glycyrrhizin. Although glycyrrhizin inhibited Y. enterocolitica biofilm formation [Fig. 3(c)], the contribution of glycyrrhizin to the inhibitory effect of licorice extract prepared in 20% ethanol on Y. enterocolitica biofilm formation was not significant, suggesting that additional components of this extract contribute to the inhibition of Y. enterocolitica biofilm formation.
Based on its content in ethanol extracts and its inhibitory effect on biofilm formation, glycyrrhizin was proposed to be the main active chemical for the inhibition of biofilm formation in S. mutans (Ham and Kim, 2019). In A. hydrophila and Y. enterocolitica, glycyrrhizin itself exhibited inhibitory effects on biofilm formation; however, its content in licorice extracts suggests the presence of additional major inhibitory chemicals for biofilm formation. The ethanol contents in extraction solvents for maximally inhibiting biofilm formation and the activity of glycyrrhizin for inhibiting biofilm formation were strain-specific. Previous studies have shown that licochalcone A inhibited biofilm formation of S. aureus (Shen et al., 2015), and glabridin also inhibited biofilm formation of E. coli and P. aeruginosa (Tsukatani et al., 2022). Evaluating the inhibitory effects of the proposed licorice extract chemicals on bacterial biofilm formation and further identifying new strain-specific inhibitory chemicals in licorice will help to elucidate the mechanisms by which they inhibit biofilm formation.
4. CONCLUSIONS
Licorice extract, which strongly inhibited biofilm formation in S. mutans (a strain that causes dental plaque), was found to inhibit biofilm formation in A. hydrophila (a pathogenic bacterium that causes illness in fishes). In particular, licorice extract prepared in 50% ethanol caused 53% inhibition at a concentration of 1.9 g/L. Moreover, licorice extract prepared in 20% ethanol inhibited biofilm formation in Y. enterocolitica, a human pathogen, by 84% at a concentration of 0.47 g/L. Glycyrrhizin, a major component of licorice, exhibited inhibitory activity against biofilm formation in both pathogenic strains tested in this study. However, the glycyrrhizin content in licorice extracts suggests that active components other than glycyrrhizin inhibit biofilm formation in both strains. These results confirm that the mechanism of biofilm formation is strain-specific. This study suggests an edible licorice extract as a new way to inhibit the formation of biofilms and facilitate their removal from contaminated sites without imposing selectivity on antibiotic-resistant mutant strains.