1. INTRODUCTION
Wood from mature trees is considered one of the most valuable forest products and is widely used as a raw material for the highest-quality wooden furniture because of its decorative quality and the strength, durability, and stability of the wood material. However, the market availability of high-quality wood from mature stands is limited; such wood has become more expensive over the last 10–15 years (Ozdemir et al., 2023; Savero et al., 2020; Song and Kim, 2023). High demand from the wood-processing industry is compounded by the shortage of raw materials from natural and community forests due to deforestation and slow wood growth. Consequently, there have been growing efforts to identify and produce fast-growing varieties of trees as substitutes to meet these needs (Hwang and Oh, 2023; Lee et al., 2024; Sumardi et al., 2022). Currently, nearly 90% of the wood utilized in the wood-processing industry comes from plantations of fast-growing tree species (KLHK, 2021; as cited in Basri et al., 2023). Teak (Tectona grandhis L.f) as one species that has been developed as a fast-growing species, holds great significance in Indonesia due to its superior quality, ornate decoration, and exceptional durability (Sutapa et al., 2024).
The national teak breeding program in Indonesia, initiated in the period 1983–1997, was a collaboration between the Indonesian State Forest Enterprise (Perum Perhutani) and the Faculty of Forestry, Gadjah Mada University (Na`iem, 2000). The ultimate goal was to increase teak plant productivity. This program included the collection of up to 600 teak trees from various locations in Indonesia. These collections were distributed across several places in Java, namely Ciamis, Cepu, Bojonegoro, and Ngawi, to carry out tests of the progeny of these specimens. Of the collection of 600 specimen plants, only 150 parent trees (ortets) showed good rooting and sprouting abilities. These were developed using a novel shoot-cutting method. Eventually, 20 superior clones were identified, of which the two best performing clones, named Jati Plus Perhutani, were selected and widely planted in numerous clonal forests in Indonesia until recently. The clones show good growth performance wherever they are cultivated and have a high growth rate, measured as the increment in stem diameter at breast height, which exceeds 3 cm (Budiadi et al., 2017; Na`iem, 2000, 2012).
Genetic traits and physiological conditions of trees are two of the many factors that significantly influence their growth rates and physical properties (Latutrie et al., 2015). Even within the same species, wood properties can vary based on the growth area or tree age. Therefore, to use wood materials efficiently, it is important to have a proper understanding of the characteristics of specific wood species and how they are used (Park et al., 2024). Wood from young plantations of fast-growing species is typically low-quality wood (Hadiyane et al., 2018). Thus, the acceleration in the growth rate of the clonal teak trees may be detrimental to wood quality. Zahabu et al. (2015) also stated that the growth rate increment of teak is often followed by changes in wood properties. Therefore, it is very important to evaluate wood quality to determine its utilization as a benchmark for the success rate of teak breeding programs.
Hidayati et al. (2015) stated that wood quality is determined by wood properties, both physical and mechanical. According to Martawijaya et al. (2005), the basic density (BD) of Indonesian teak ranges from 620 to 750 kg/m3, and the radial and tangential shrinkage values are 2.8% and 5.2%, respectively. In terms of mechanical properties, the modulus of rupture (MOR) value of typical teak wood in Indonesia is 101.11 MPa and the modulus of elasticity (MOE) is 12.52 GPa. The average value of compressive strength (CS) parallel to the grain is 53.94 MPa, and the average value for hardness is 41.97 MPa. Furthermore, physical conditions, such as heartwood percentage, are also important factors because of their relationship with the natural durability of wood. In contrast, color is one of the quality attributes that influence perceptions by customers of wood products (Sandoval-Torres et al., 2010). Various studies have been conducted on the physical and mechanical properties of teak (Basri et al., 2015; Darmawan et al., 2015; Hidayati et al., 2015; Zulkahfi et al., 2020) and its physical conditions (Basri and Wahyudi, 2013; Hidayati et al., 2015, 2022; Lukmandaru et al., 2018). Several specific studies of the wood properties of clonal teak trees less than 15 years old have also been conducted by many researchers (Basri and Wahyudi, 2013; Hidayati et al., 2015; Seta et al., 2023) with various results. Researchers have generally reported that some of the properties of clonal teak were similar to those of conventional teak properties, whereas the values of other properties were either higher or lower.
The oldest existing clonal teak plantation in Indonesia is 20 years old, and the physical and mechanical properties of its wood have not yet been investigated. It is important to evaluate the improvement in wood quality from the older clonal teak to determine its proper utilization. The specific aim of this study was to investigate the physical conditions and physical and mechanical properties of 20-year-old clonal teak planted in Ngawi, East Java, Indonesia. The properties were measured based on the axial and radial variations. Given the shorter rotation and greater growth rate of clonal teak, it is expected to have properties similar to or higher than those of conventional teak. If the values of any of the key properties are lower in clonal teak than in conventional teak, further work is necessary to improve the teak being grown in plantations. Thus our results provide important information for future silviculture and plantation management.
2. MATERIALS and METHODS
Three clonal teak trees were harvested from the Perum Perhutani clonal teak plantation, at compartment 7H, located in Gendingan, Ngawi, East Java, Indonesia (7°22’26.7”S 111°12’29.0” E, 108 masl) in October 2022. The clonal plantation covers an area of 27 ha and consists of clonal teak plants from a teak breeding program established in 1997. The average temperature of the location is 25.35°C, and relative humidity is 80%–90%. Annual precipitation is 1,500 mm/year. The topography of the location is flat and the soil is Grumusol. The average diameter of the trees was 24.83 cm and the average height 23.07 m. Wood disks 5–6 cm in thickness and short logs 50 cm in length were cut from the bottom, middle, and top parts of the tree stems (18 disks and 9 logs in total). The bottom, middle, and top parts were assessed in the axial direction, whereas those near the pith, middle, and near the bark were assessed in the radial direction. The disks were then used to investigate their physical condition (wood color and heartwood percentage), physical properties [moisture content (MC), BD, shrinkage, and tangential/radial shrinkage (T/R) ratio], and mechanical properties: MOE, MOR, and CS parallel and perpendicular to the grain, hardness, and cleavage strength.
The wood color and heartwood percentage were measured from a 5 cm thick disk. Among all the parameters, in wood color, we only used radial variation, outer heartwood, and sapwood. The percentage of heartwood can only be performed in terms of axial variation.
The wood color was measured in the transverse section of a 5 cm air-dried disk using a colorimeter (NF333, Nippon Denshoku, Tokyo, Japan). The CIELAB (L*, a*, b*) system was employed to investigate the wood color, with L* indicating psychometric lightness with a maximum scale of 100 that shows white or brightness, and a minimum scale of 0 that shows black or darkness, a* denotes a color parameter on the red (+) or green (–) axis, and b* denotes a color parameter on the yellow (+) or blue (–) axis. To determine the percentage heartwood, the total area of heartwood was measured in the transverse section using the image analysis software Fiji ImageJ with a polygonal line feature. The heartwood percentage (%) was calculated as follows:
Where Hw is the heartwood area and Wt is the total wood area (including heartwood and sapwood).
The physical properties were tested according to British Standard 373:1957, “Methods for Testing Small Clear Specimens of Timber”. A total of 27 specimens were prepared for the BD, MC, and shrinkage measurements. Samples 2 cm × 2 cm × 2 cm (radial × tangential × longitudinal) were cut from a 5 cm thick disk for BD and MC measurements, and samples 2 cm × 2 cm × 4 cm (radial × tangential × longitudinal) were cut from a 6 cm thick disk for shrinkage measurements. BD and MC were calculated as follows:
Where BD is the basic wood density (g/cm3 converted to kg/m3), Wod is the oven-dried weight (g), Vg is the green volume (cm3), MC is the moisture content (%), and Wad is the air-dried weight (g).
Shrinkage measurements were carried out by measuring the volumetric changes daily from green to air-dried conditions. The radial, tangential, and longitudinal sections were marked for length measurements using a pair of digital calipers. The final shrinkage (%) and T/R ratio were determined as follows:
Where Lg is the wood-green length (mm), Lad is the wood-air-dried length (mm), and T/R is the wood tangential and radial shrinkage ratio. TS is the wood tangential shrinkage (%) and RS is the wood radial shrinkage (%).
The mechanical properties were tested using the British Standard 373:1957. Seven tests were conducted to determine the static bending strengths of the MOR and MOE, as well as CS parallel and perpendicular to the grain, hardness, and cleavage strength. For each type of test, 27 specimens were prepared from 50-cm short logs, of standard dimensions (radial × tangential × longitudinal, respectively): 2 cm × 2 cm × 30 cm for static bending tests, 2 cm × 2 cm × 6 cm for CS parallel and perpendicular to grain, 2 cm × 2 cm × 4 cm for hardness, and 2 cm × 2 cm × 4.5 cm for cleavage strength. The MOE, MOR, and CS values parallel and perpendicular to the grain, hardness, and cleavage were calculated from the following equations:
Where MOE is the wood modulus of elasticity (kg/cm2 converted to GPa), ΔP is the load changes in proportion limit area (kg), L is the span length of the wood sample (cm), ΔY is the deflection at mid-length at the limit of area (cm), b is the wood sample width (cm), h is the wood sample thickness (cm), MOR is the wood modulus of rupture (kg/cm2 converted to MPa), CS is the wood compressive strength (kg/cm2 converted MPa), Pmax is the maximum load (kg), Aparallel is the wood sample surface parallel to the grain (cm2), Aperpendicular is the wood sample surface perpendicular to the grain (cm2), and A is the wood sample pressed surface area (cm2). The hardness and cleavage units are MPa (converted from kg/cm2) and kg/m (converted from kg/cm), respectively.
The tests were performed using a Universal Testing Machine (3369, Instron, Norwood, MA, USA) with a load speed of 0.25 cm/min for static bending strength and hardness, 0.06 cm/min for CS parallel and perpendicular to grain, 0.64 cm/min for hardness, and 0.1 cm/min for cleavage strength. The results were then calculated using the software Bluehill 3 (Instron).
Data from each parameter obtained were then analyzed with statistical analysis software SPSS 25 (IBM, Armonk, NY, USA) with a 95% confidence level. The results were analyzed using two-way analysis of variance (ANOVA), and the significance of differences between axial and radial variation of physical condition, physical properties, and mechanical properties were calculated at α = 0.05.
3. RESULTS and DISCUSSION
The results of the statistical analysis of the physical conditions are listed in Table 1. The mean values of the wood color for L*, a*, and b* were 39.82, 11.43, and 22.14, respectively, and the mean heartwood percentage was 64.54%. The statistical analyses of wood color and heartwood percentage is summarized in Table 1.
The L* parameter showed significant differences in both the axial and radial variations. The a* and b* parameters showed different results, with significant differences found only in the axial direction. The color measurement results are shown in Fig. 1. The highest L* value in the axial direction was 42.94 in the bottom part of the stem and the lowest was 34.60 in the top part of the stem. In contrast, the highest L* value in the radial direction was 47.66 in the sapwood and the lowest was 33.20 in the outer heartwood. Furthermore, the highest a* and b* values in the axial direction were 13.24 and 25.88, respectively, in the same position on the bottom part of the stem, and the lowest value were 8.90 and 15.90, respectively, in the same position on the top part of the stem. The axial variations in L*, a*, and b* showed the same decreasing trend from the bottom to the top of the stems, whereas the radial variation in L* did not exhibit any particular trend.
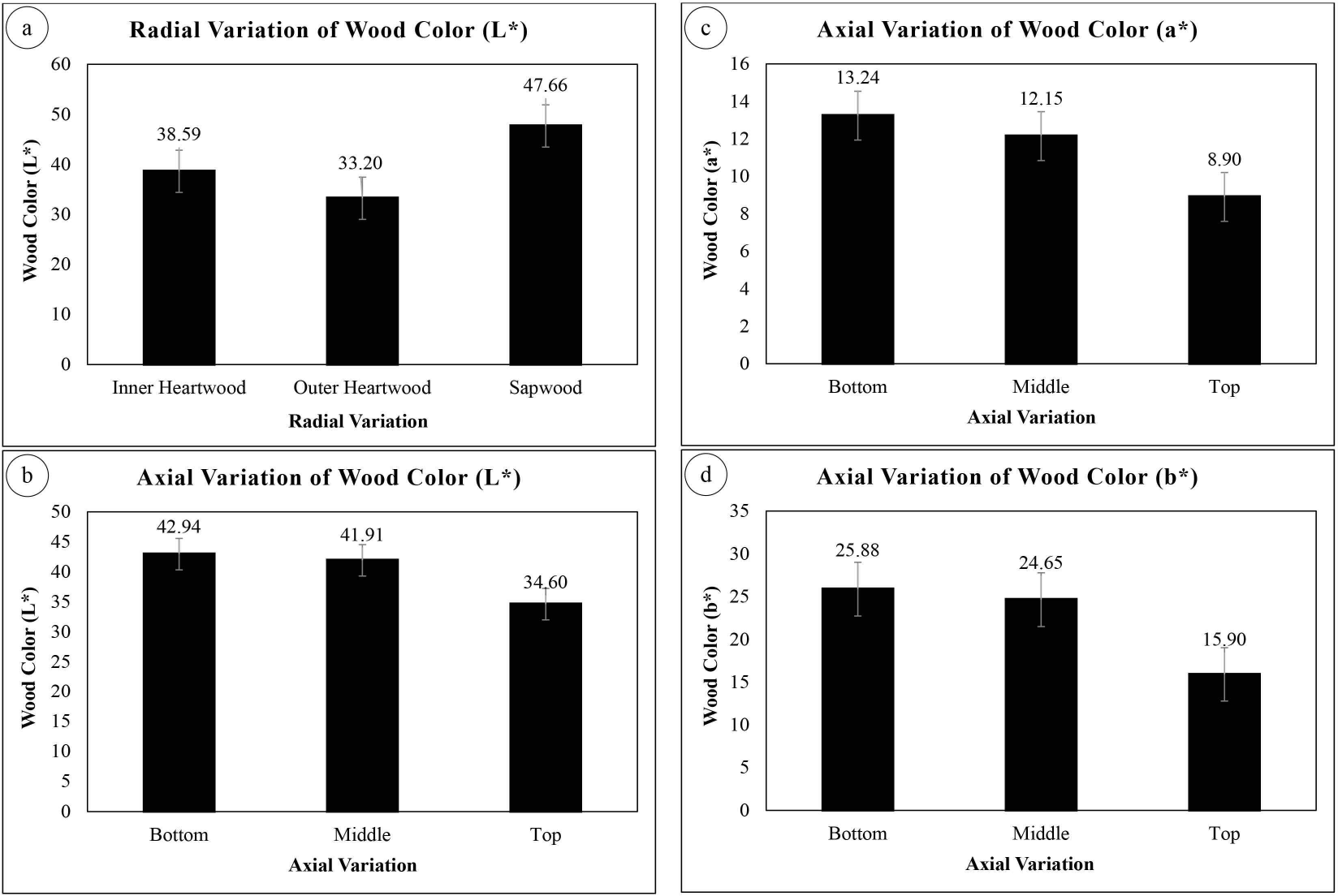
The results of the wood color measurement L*, a*, and b* of the sampled clonal teak were compared with different reference groups of teak trees: 10-yr-old clonal teak (Hidayati et al., 2015), 15-yr-old clonal teak (Hidayati et al., 2022), and 32-yr-old conventional teak (Lukmandaru et al., 2009). The results indicate that the teak in this study appeared darker, less red, and less yellow. The teaks was greener and bluer in color than teaks from other studies. However, these differences must be interpreted with caution because many factors influence color, such as age, soil fertility, and climatic conditions prevailing in the area where the trees were obtained (Moya and Marín, 2011). The lower value of wood color in the present study may have been caused by many factors. Furthermore, as stated before, the L*, a*, and b* parameters showed a lightness axis, red-green axis, and yellow-blue axis, respectively; a significant difference in the axial or radial direction will result in significant contrast in appearance. These results have a positive value for the final use of wood and potentially influence customer perceptions.
In comparing the different groups of teak, there was no significant difference in heartwood percentage in the axial variation, as shown in Table 1. The heartwood percentage of the wood analyzed in the present study was higher than that of 10-yr-old clonal teak (Hidayati et al., 2015) and 9-yr-old clonal teak (Basri and Wahyudi, 2013); however, the heartwood percentage we report here was lower than that of a more recent sampling of 10-yr-old clonal teak as well as 10-yr-old conventional teak (Hidayati et al., 2022). The heartwood percentage in the present study was lower than that of 35-yr-old conventional teak (Sumarni and Muslich, 2008). Nonetheless, the mean diameter of clonal teaks in this study was higher than that reported in other studies. Although the heartwood percentage tended to be lower, a greater diameter will provide more stock of heartwood to customers. When the results of the heartwood percentage are considered together with the greater diameter, the overall result is beneficial from a commercial standpoint.
The statistical analysis results of the physical property parameters showed that most were not significantly different based on the axial and radial variation. The results of the physical property analysis are listed in Table 2.
The mean value of MC was 15.45%; this value is within the range according to Prawirohatmodjo (2012) that the MC varies between 15%–20% because the relative humidity value in tropical countries like Indonesia is generally high, between 80% and 90%. There were no significant differences in MC in the axial and radial variations; in other words, MC was balanced across all parts of the stem. Tiemann (1906) stated that water displacement is followed by an increase in strength. As can be seen, the MC spreads equally among the axial and radial variations, which also means that the strength increment is balanced through the axial and radial variations.
Mean BD was 525.67 kg/m3 and showed a significant difference only in the radial direction. The lowest value of radial direction was 480.00 kg/m3 in the near pith, and the highest value was 556.67 kg/m3 in the near bark. The BD measurements are plotted in Fig. 2.
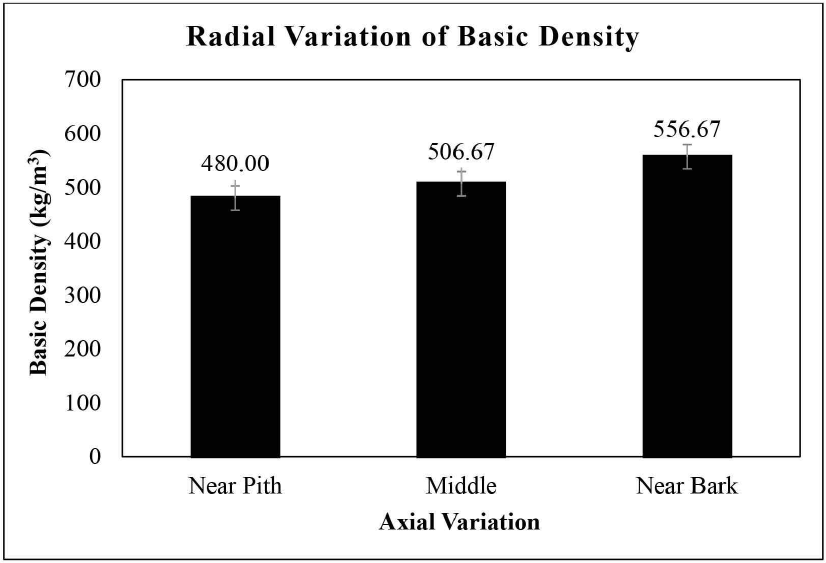
The BD in the present study was higher compared to that of 10-yr-old clonal teak (Hidayati et al., 2015), 15-yr-old clonal teak (Hidayati et al., 2022), and 15-yr-old clonal teak (Seta et al., 2023), but was lower compared to the corresponding values in 40-yr-old conventional teak (Darmawan et al., 2015), indicating that the clonal teak in the present study was probably still in the juvenile phase. Bhat et al. (2001) stated that teak trees begin to mature at approximately 15–25 years. Therefore, the BD of teak in the present study still has the potential to increase. In the National Standard of Indonesia (SNI) 01-0608-1989, wood intended for furniture making should have a BD above 400.00 kg/m3, and the BD values of the clonal teak in the present study met this requirement.
Furthermore, the mean values of radial, tangential, and longitudinal shrinkage were 0.65%, 1.25%, and 0.31%, respectively. These values were lower than those of conventional teak from several places in Gunungkidul, Indonesia (Marsoem et al., 2014) and mature to aged (50- to 70-yr-old) conventional teak from East Timor (Miranda et al., 2011). Among the shrinkage rates recorded in the present study, the only significant difference among the samples was radial shrinkage in the axial direction. The highest value of radial shrinkage was 1.57% at the top part of the stem, and the lowest was 0.46% at the bottom part of the tree. A plot of the radial shrinkage measurements in the axial direction is shown in Fig. 3.
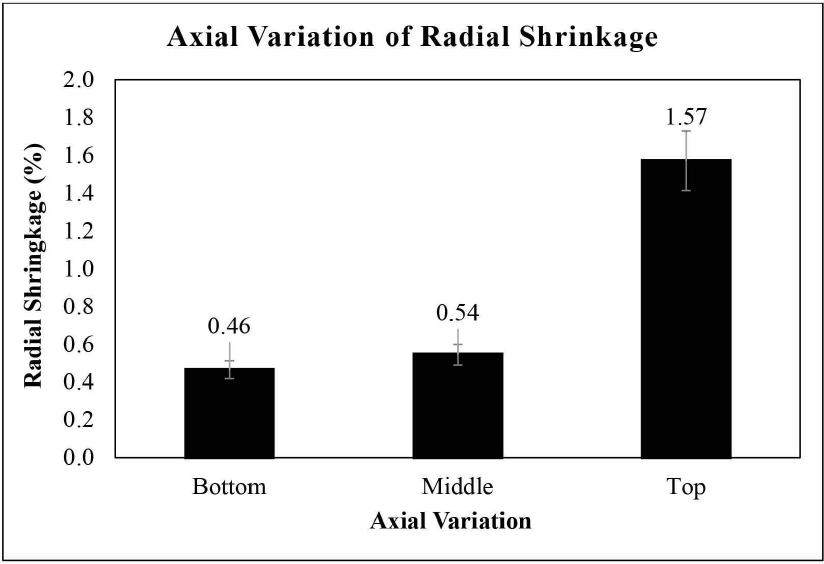
The shrinkages in the present study were also lower compared of 15-yr-old clonal teak (Hidayati et al., 2022), 15-yr-old clonal teak (Seta et al., 2023), 11-yr-old clonal teak and 14-yr-old seed source teak (Hidayati et al., 2015). The shrinkage in the present study showed promising results in general, even when compared to results from several other studies. The clonal teak we analyzed had greater dimensional stability and was more suitable for commercial purposes. However, the T/R ratio was lower than the corresponding value of other teak samples in previous studies, as mentioned earlier. This also supports the finding that the clonal teak in this study had more dimensional stability. The shrinkage in general and T/R ratio did not show any significant difference, which means that most of the parts of the stems have good dimensional stability, which provides added value as it indicates that most parts of the stem have high potential for use in any products that require high dimensional stability.
The statistical results of the mechanical property analyses are presented in Table 3. The average value of MOE was 9.35 GPa, which is lower compared to mature-aged teak from East Timor (Miranda et al., 2011), 65-yr-old mature-aged teak from India (Bhat and Priya, 2004), and 15-yr-old clonal teak (Hidayati et al., 2022). In contrast, the results we report here were similar to those of 10-34-yr-old teak planted in Panama (Posch et al., 2004), and 21-yr-old teak from India (Bhat and Priya, 2004). The MOE exhibited a significant difference in both the axial and radial directions. The highest axial and radial direction values were 10.10 GPa in the middle part of the stem and 10.21 GPa in the near bark, as shown in Fig. 4.
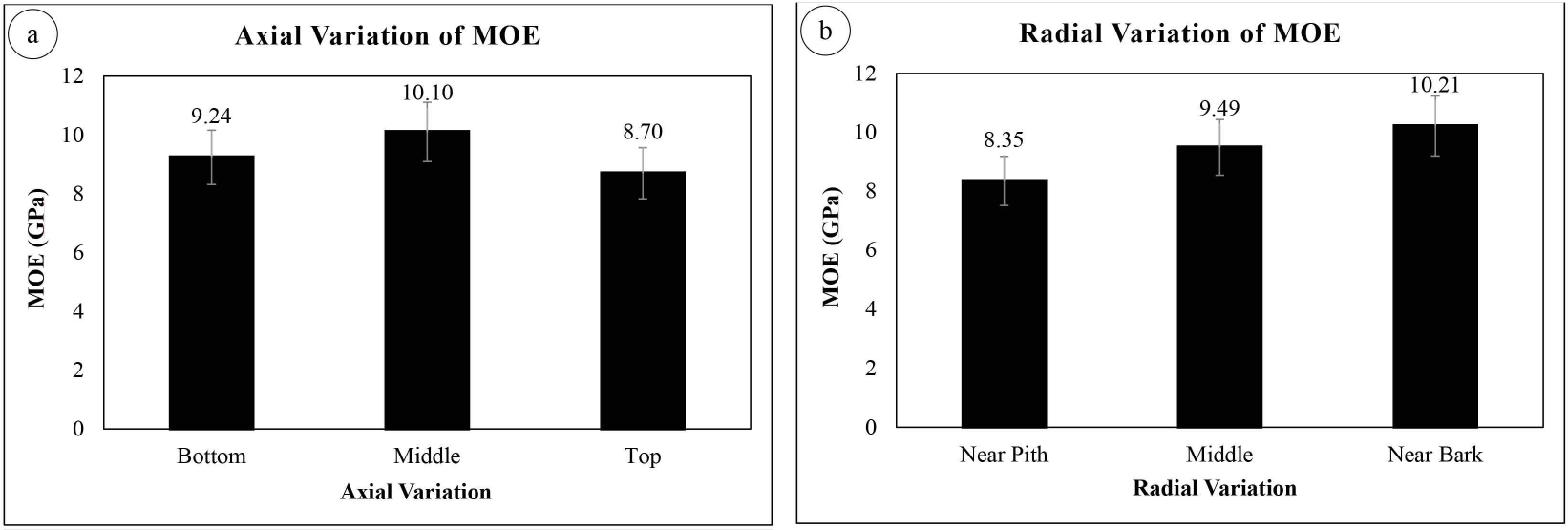
The mean MOR was 72.00 MPa. This value is lower than that of mature teak from East Timor (Miranda et al., 2011), variously aged teak from Panama (Posch et al., 2004), 21-yr-old teak from India (Bhat and Priya, 2004), and 40-yr-old conventional teak (Darmawan et al., 2015). MOR showed a significant difference only in the radial direction. The value increased from near the pith to near the bark, with the highest value of 80.25 MPa, as shown in Fig. 5.
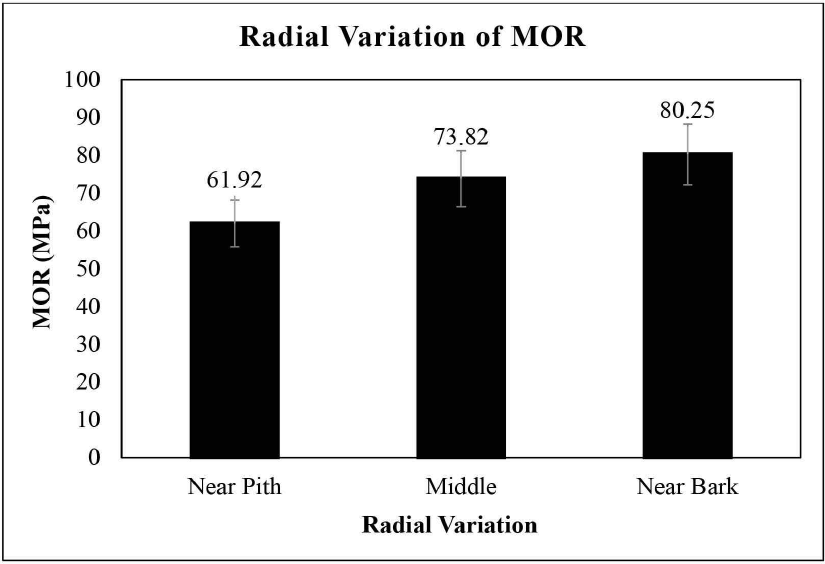
The mean values of CS parallel and perpendicular to grain were 37.93 and 13.45 MPa, respectively. These values were lower than those reported in several other studies, such as mature teaks from East Timor (Miranda et al., 2011), variously aged teaks from Panama (Posch et al., 2004), and 15-yr-old clonal teak (Hidayati et al., 2022). The CS parallel to grain showed a significant difference in the axial and radial directions, with the highest values of 39.40 and 41.07 MPa, respectively, as illustrated in Fig. 6. Furthermore, the CS perpendicular to the grain showed a significant difference in both the axial and radial directions, with maximum values of 15.11 and 14.96 MPa, respectively, as shown in Fig. 7. Based on the MOE, MOR, and CS values, the clonal teak in the present study was within strength classes II-III–according to Seng (1990).
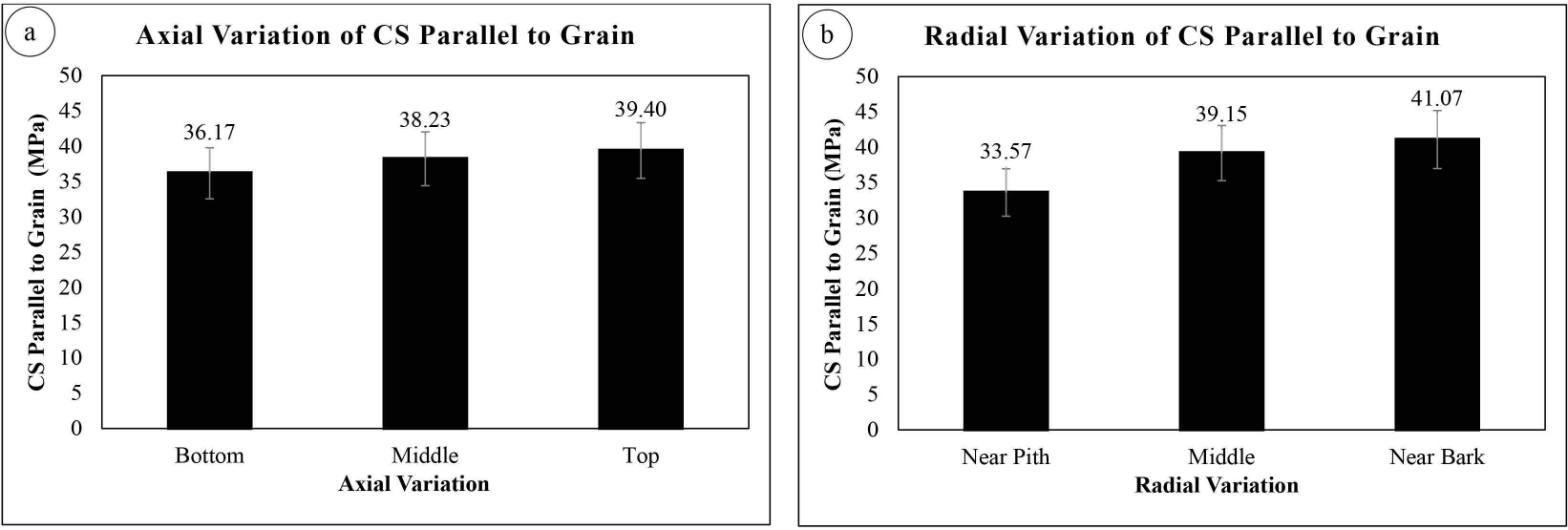
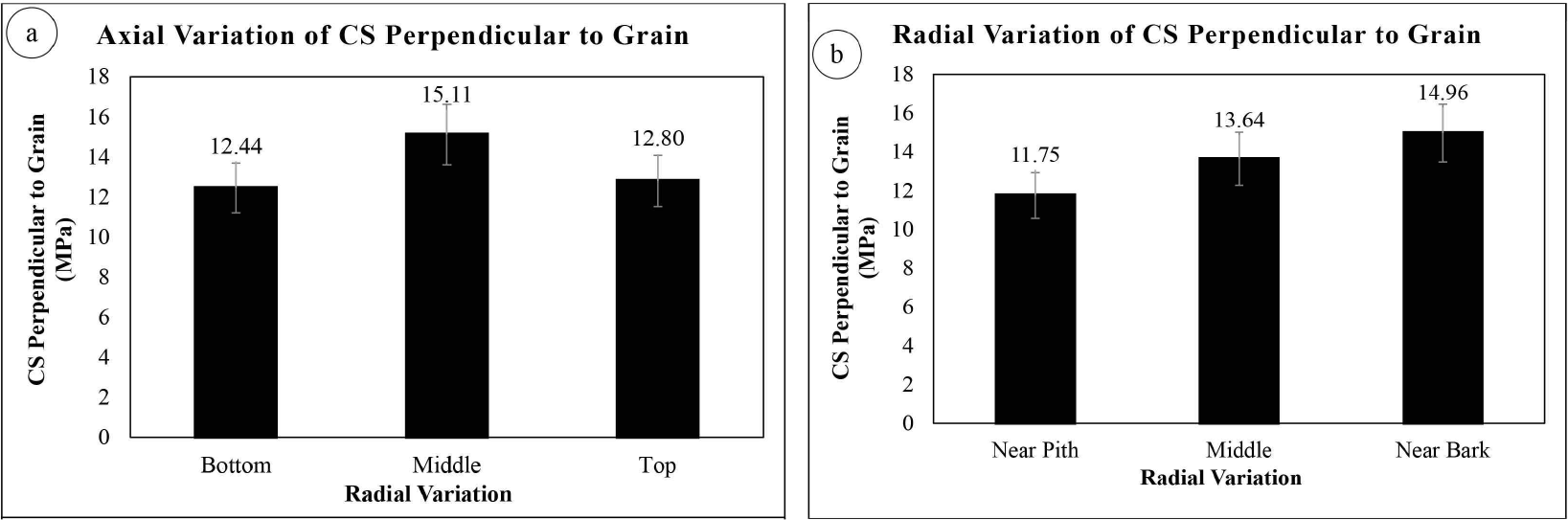
The mean values of hardness and cleavage strength were 19.29 MPa and 554.50 kg/m, respectively; these values were lower than the corresponding values reported for 15-yr-old teak planted in Ghana (Amoah and Inyong, 2019), and 5-yr-old fast-growing teak (Wahyudi et al., 2014). Both hardness and cleavage strength showed significant differences in the radial direction. The highest value was 20.59 MPa in the middle and 638.28 kg/m in the near bark, respectively, as presented in Fig. 8.
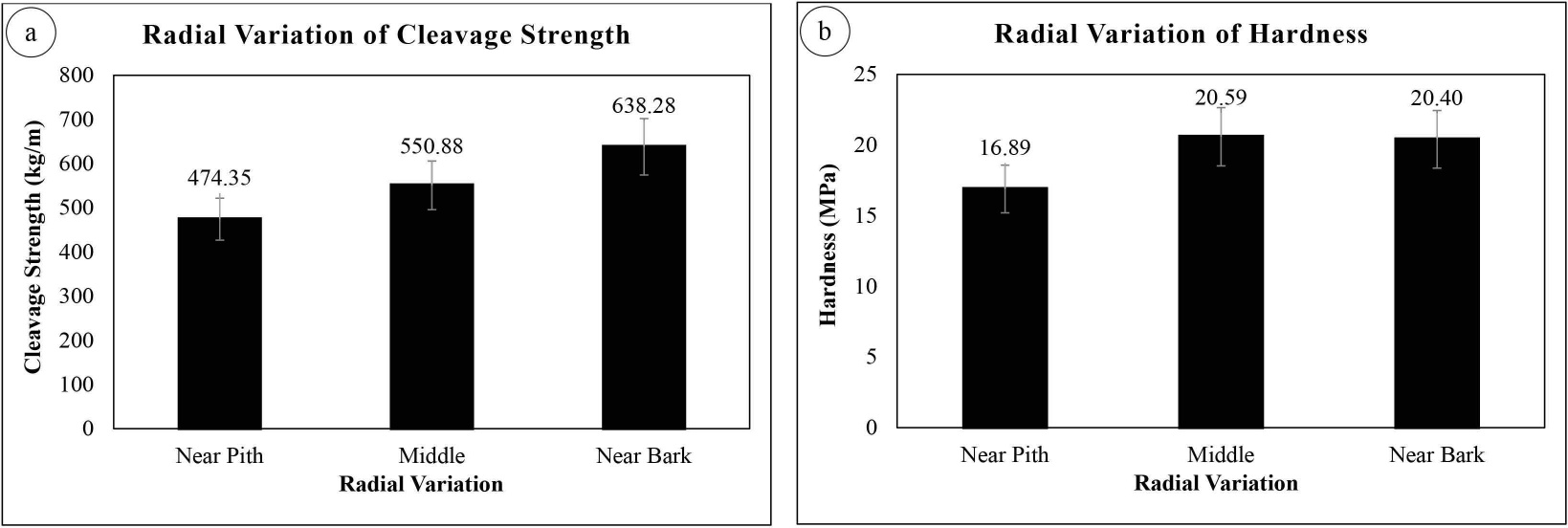
There is limited information available on the hardness and cleavage strength of teak. In line with other mechanical characteristics, the hardness and cleavage strength also tended to be lower than those of teak reported in other studies. Compared with several other studies, the mechanical properties were mostly lower but still within the range of teak mechanical properties in general. This indicated that the clonal teak in the present study was of sufficient quality. Accompanied by other properties, clonal teak is profitable and promising, allowing it to open the gates for future silvicultural treatment and land management.
4. CONCLUSIONS
The clonal teaks in this study generally showed good characteristics. The clonal teak was within strength classes II-III. According to this class, this clonal teak has considerable potential for use, although it still cannot be concluded that it reaches the quality of general teak at its prime age level. However, it should be noted as the important point that the clonal teak trees in this study were relatively young and had a high potential for enhancement. Clonal teak wood is suitable for light construction, carpentry, packaging, crafts, household furniture, decorative veneers, musical instruments, and other uses.
Moreover, the physical conditions show good potential for future use. The wood color was slightly different to that of younger clonal and mature conventional teak. The wood color looks a bit darker, greener, and bluer. The heartwood percentage in the present study was lower than that of mature conventional teak. Furthermore, the heartwood percentage is higher than some younger clonal and seed source teaks, on the other side, the value is lower than some younger clonal and conventional teaks from previous research. However, it should be noted that the diameters of the clonal teaks in this study were extraordinarily large. A high heartwood percentage accompanied by a high diameter will provide an enormous amount of teak wood stocks of good quality, and those supported by aesthetic wood color will improve the value.
Furthermore, the physical properties were similar to those of teak reported previously. The BD of the clonal teak was higher than that of some younger clonal and seed source teaks from previous studies, but the value was slightly lower than that of mature conventional teak. The shrinkage and T/R ratio of this clonal teak were lower than those of younger and mature-aged teaks from previous research, which means that this teak has better dimensional stability. Furthermore, the mechanical properties of this clonal teak tended to be similar to those of younger clonal and seed source teaks; however, the values were lower than those of mature teaks from previous research. Improvements are needed to increase the value, because the results were in the lower range of teak mechanical scores. However, it can be concluded that the breeding program with these clonal teaks succeeded in producing teak plantations with shorter cycles and a higher quantity of timber and improved some indicators of wood quality.