1. INTRODUCTION
Indonesia has abundant natural forest resources, and many wood species have the potential to be used as raw materials in the pulp and paper industries. Mangrove plantations grow along Indonesian coastlines. Although these plantations are typically used for conservation purposes, it is feasible to develop industrial mangrove plantation forests for industrial use (Dahdouh-Guebas et al., 2000; Istomo, 2020; Yudha, 2021).
Understanding wood characteristics is necessary because the properties and characteristics of wood species differ significantly from one another, and these characteristics determine the quality of the final product (Park et al., 2024). Wood fibers are used as materials for making home industry products, such as threads, bags, mats, knots, and baskets (Jain et al., 2022). The specific gravity and dimensions of wood fibers are among the key variables in producing pulp and paper materials.
The selection of wood species for carpentry and housing is well understood. However, choosing wood species to manufacture pulp and paper involves different criteria and must meet specific raw material requirements. Pulp and paper are essential for the printing, packaging, and office support industries. Indonesia’s pulp production has reached 6.9 million tons per year, and paper is at 11.5 million tons per year, surpassing plantation-producing countries such as Brazil, the United States, and China (Anonymous, 2019). Despite this, the pulp and paper industry faces limited availability of raw materials, which are predominantly sourced from wood. Another significant issue is the negative publicity associated with forest and land fire disasters, which result from clearing forest areas for Industrial Plantation Forests (HTI).
Generally, the pulping process uses wood from fast-growing tree species originating from HTI. Many fast-growing wood species are cultivated to meet the raw material demands of the wood industry in Indonesia (Laksono et al., 2023; Nawawi et al., 2023). Consequently, HTI has become a priority for the Indonesian government to increase the supply of raw materials for the wood pulp industry (Fadwati et al., 2023). Common wood species used for pulp and paper raw material production are Acacia (Acacia mangium), Jabon (Anthocephalus cadamba), Mahang (Macaranga sp.), Leda/ Eucalyptus (Eucalyptus sp.), Tusam/Pinus (Pinus sp.), and others like Gerunggang (Cratoxylon sp.), Binuang (Duabanga sp. and Octomeles sp.), and Jelutung (Dyera sp.). According to Marbun et al. (2019), Benuang (Octomelessumatrana), Duabanga (Duabanga moluccana), Pisang merah (Horsfieldia hellwigi), and Terap (Artocarpus odoratissimus) are also suitable raw materials for pulp and paper manufacturing. Efforts to identify additional potential raw materials for the pulping industry should focus on well-known wood species and other species compatible with pulp making. There is a need for information on wood species from non-HTI sources that are suitable for use as raw materials of pulp and paper.
As a country with a vast coastline consisting of thousands of islands and 65% water territory (Hadi et al., 2016), Indonesia has extensive mangrove forests that warrant exploration. The mangrove forest vegetation in Indonesia has high species diversity, with a total of 202 recorded species, consisting of 89 tree species. However, only approximately 47 specific plant species are exclusive to mangrove forests (Matan et al., 2010). Globally, mangrove forests cover approximately 15 million hectares (Hoff et al., 2014), with Indonesia’s mangrove forests estimated to span 3 million hectares, or about 20% of the world’s mangrove forest area (Rahadian et al., 2019; Wanma et al., 2019). Indonesia’s mangrove forests extend along the east coast of Sumatra, the north coast of Java, the west and east coasts of Kalimantan, the bays of Sulawesi, the small Maluku islands, and the Papua's southern coast. According to Sreelekshmi et al. (2018), mangrove forests consist of distinct zones: the seaward/ fringing zone (Rhizophora sp., Avicennia sp., Sonneratia sp.), the intermediate/mid zone (Excoecaria sp., Sonneratia sp., Kandelia sp., Acanthus sp.), and the landward zone (Bruguiera sp., Acrostichum sp., and Avicennia sp.). The zonation pattern is established with discrete groupings of mangrove species. The growth of each plant species adapts to the surrounding environment, leading to morphology variations from one place to another (Hadi et al., 2016). Some mangrove species in Indonesia grow in the landward zone and are highly productive, making them suitable for sustainable exploitation.
Mangrove products can be obtained directly from the forests (wood) and their derivatives. Mangrove wood is most commonly used as a fuel source, either as charcoal or firewood, and as the primary material for the construction of boats, houses, furniture, and more (Hoff et al., 2014). Moreover, mangrove trees contain various compounds, such as alkaloids, phenolics, saponins, flavonoids, steroids, and terpenoids (Gouda et al., 2015). Besides wood for fuel and construction, mangrove forest trees are widely valued for their bark (used in tanning and dyes) and wood fiber (used to make rayon and paper; Walters et al., 2008). According to Chibudike and Udohitinah (2009), Rhizophora racemose is utilized for the production of pulp, paper, and related products. Given Indonesia’s extensive coastline, rich in mangrove plant species, it is necessary to explore the potential of using mangrove wood as an alternative material for pulp and papermaking (Emerhi, 2012).
Notably, before utilizing certain wood species, it is essential to understand their properties. The suitability of wood species for paper sheet processing is strongly influenced by the specific gravity and dimensions of the fiber and its derivatives, as well as the content of its chemical components. Chemical components in wood affect the consumption and penetration of cooking ingredients, while specific gravity greatly influences the suitability of the cooking process. Additionally, fiber morphology affects the quality of the bonds between the fibers (Fatriasari and Hermiati, 2008). To elucidate the morphological properties of mangrove wood species suitable for pulp and paper raw material production, we studied the fiber dimensions and their derivatives of seven mangrove wood species.
2. MATERIALS and METHODS
Wood samples of seven mangrove tree species were obtained from plantation forests in Desa Pabean Ilir-Pasekan, Indramayu Regency, West Java Province (Fig. 1). The seven species of mangroves analyzed included Avicennia alba, Bruguiera gymnorhiza, Bruguiera cylindrica, Hibiscus tiliaceus, Sonneratia ovata, Sonneratia caseolaris, and Excoecaria agallocha (Table 1). The samples were collected from the base of the tree. For each wood species, a disc was taken from a mature tree (10–25 years old) and divided into three parts for maceration (Fig. 2): one part near the bark and the others in the middle and near the pith.
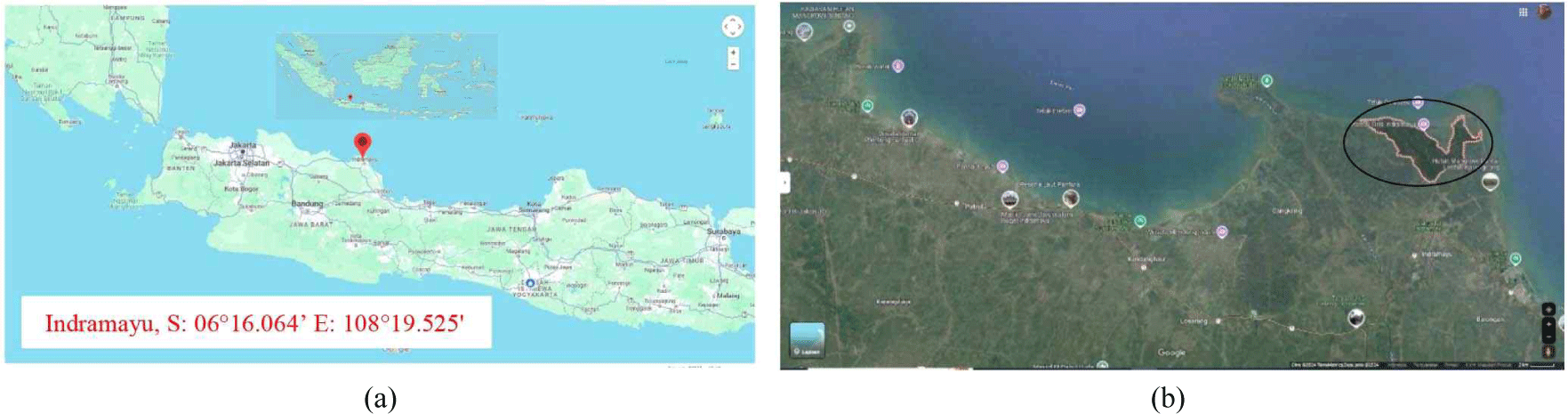
Maceration preparations were performed according to Tesoro (1989) to observe the fiber dimensions. Matchstick-sized pieces of wood samples were heated slowly in a test tube containing a 1:1 solution of hydrogen peroxide with glacial acetic acid. The separated fibers were washed thoroughly with tap water and stained with safranin. Subsequently, the colored fiber was placed on a glass slide, dripped with glycerin, spread evenly, and covered with a cover glass at which point the samples were ready for the measurements. The fiber length, fiber diameter, and fiber lumen diameter were measured using a microscope. The cell dimension data (vessels and fibers) obtained were quantitatively analyzed using the MINITAB 14 program. The values obtained were expressed as confidence intervals for the mean value, namely x ± t (0.025, db = n – 1) x mean SE, where x is the average value, t is the value of the t-student distribution at the significance level α = 5%, and SE is the standard error of the mean (Mattjik and Made Sumertajaya, 2002).
The fiber dimensions were observed using an Olympus microscope CX 23LED. The fiber length was measured at 100 × magnification, and the fiber and lumen diameters were measured at 400 × magnification. The fiber length, wall thickness, and lumen diameter were determined using the maceration method developed by the Forest Product Laboratory (Rulliaty, 1994). Using a micrometer, measurements were conducted on 25 samples for fiber length and 15 samples for fiber diameter and lumen diameter. The average fiber dimensions were calculated using the MINITAB 14 program. The derivative value of the fiber dimension for the fiber quality classification was calculated according to Silitonga et al. (1972):
Where l is the fiber lumen diameter (μm), L is the fiber length (μm), d is the fiber diameter (μm), and w is the fiber wall thickness (μm).
The determination of the fiber quality class/grade for raw paper material followed the guidelines of Rachman and Siagian (1976; Table 2).
Data from Rachman and Siagian (1976).
3. RESULTS and DISCUSSION
The complete fiber length and thickness data are listed in Table 3, and the morphology of the mangrove wood fiber are depicted in Fig. 3. Cell wall thickness and fiber length influence the specific gravity of wood species. Notably, fibers of B. gymnorhiza and A. alba were observed to be longer and possess thicker cell walls.
Data of specific gravity from: Oey (1990).
The quality of wood fibers can be predicted using their dimension derivative value. The fiber dimension derivative values of the seven mangrove wood species analyzed are listed in Table 4.
Wood species with well-known properties can be used to produce high-quality products. Understanding the foundational properties of mangrove wood is essential for optimizing sustainable forest product utilization (Sushardi and Kodi, 2018). Wood fiber quality plays a crucial role in pulp and paper production, with fiber dimensions and derivative factors serving as fundamental predictors of pulp properties (Syafii and Siregar, 2006). Our analysis revealed that B. gymnorhiza exhibits the longest fibers (1,405.51 μm), whereas S. ovata has the shortest fibers (927.82 μm). B. gymnorhiza and B. cylindrica also exhibit the thickest cell walls (7.02 and 7.20 μm, respectively) compared to the other species. In contrast, H. tiliaceus shows the thinnest cell walls (4.09 μm), albeit thicker than H. cannabinus from India (3.6 μm; Sivan et al., 2021). Fibers of B. gymnorhiza from India are reported to be 2,330 μm long with a 12.5 μm thick wall (Sun and Suzuki, 2000). The cell wall thicknesses of mangrove wood species exceed those of kapok (Ceiba pentandra) and balsa (Ochroma pyramidale), which are reported to have 1.34 μm and 2.40 μm cell walls, respectively, according to Purnawati et al. (2018).
Wood species intended for pulp and paper raw materials typically have a specific gravity ranging from 0.35–0.8 (Brown et al., 1994; Syafii and Siregar, 2006). According to Oey (1990), the specific gravities of the mangrove tree species analyzed in this research are as follows: B. gymnorhiza and A. alba have relatively high specific gravities of 0.91 and 0.83, respectively, while H. tiliaceus, S. ovata, S. caseolaris, and E. agallocha have relatively low specific gravities of 0.54, 0.46, 0.49, and 0.41, respectively. According to Haroen (2016), wood species used as raw materials for pulp should have a specific gravity of less than 0.70. Hardwood, which has a low specific gravity of less than 0.70, produces better pulp quality in terms of high tear and tensile properties. A higher specific gravity also affects the pulping process, especially in the use of chemicals. Wood species with a high specific gravity generally have thick fiber walls, making them less suitable as raw materials. Wood species with thinner fiber walls soften more easily, creating a wider surface area of the pulp sheet to form bonds between fibers, resulting in high tensile, breaking, and folding strengths (Nurrahman and Silitonga, 1973).
The mangrove wood species analyzed in this study generally have thinner fiber walls than Bruguiera sp. According to Nurrahman and Silitonga (1973), thin-walled fibers can produce dense pulp and paper sheets with high burst strength. Thin-wall fibers can also easily soften and flatten, which causes a large surface area to form bonds between cells, resulting in high tensile, breaking, and folding strengths (Casey, 1979).
The fiber length of the mangrove wood species analyzed in this study ranged from 927.82 μm to 1,405.51 μm, categorizing them into the medium class (Nurrahman and Silitonga, 1973; Wheeler et al., 2008). This range is significantly shorter compared to Rhizophora sp. From mangrove forests in Nigeria, which reach 1,657–1,719 μm (Emerhi, 2012). However, Sushardi and Kodi (2018) report that R. mucronata falls under class III pulp quality. According to Kasmudjo (2010), the broadleaf wood species commonly exhibit a fiber length range of 800–1,500 μm. Notably, longer wood fibers produce pulp with high tear strength (Rizqiani et al., 2019; Syafii and Siregar, 2006; Walia, 2013).
The fiber quality grade shown in Table 4 indicates that H. tiliaceus, S. caseolaris, and E. Agallocha have a fairly low Runkel number (0.41–0.47), placing them within the class II criteria (Artistien and Mandang, 2002). Research on fiber quality in Hibiscus cannabinus indicated that this species also exhibits a low Runkel number, making it suitable for producing paper of various grades (Dutt et al., 2009). According to Setyayunita et al. (2022), H. cannabinus fibers are a natural fiber used as raw material in various industries such as fiberboard, pulp, and paper. Syafii and Siregar (2006) noted that a low Runkle ratio is a good match for pulping because it indicates that the fiber wall and lumen diameters are wide, easy to flatten, and have high tensile and bursting strengths. Fibers with a Runkel ratio of less than 1 are considered ideal for papermaking, as they are more flexible and foldable and produce paper sheets with improved fiber bonding (Nasser et al., 2015). In contrast, the Runkel ratio of the fibers of Laguncularia racemosa (Combretaceae) is 1.10, indicating regular quality for paper production (Yáñez-Espinosa et al., 2004).
The felting power of all mangrove wood species analyzed in this study was low (34.20–53.40). According to Syafii and Siregar (2006), a fiber with a high felting power has a better tearing strength and is more flexible than a fiber with a low felting power.
The Muhlsteph ratios of the mangrove wood species analyzed in this study was classified as class III (B. gymnorhiza, B. cylindrical, A. alba, S. ovata) and class II (H. tiliaceus, S. caseolaris, E. agallocha). Fibers with high Muhlsteph ratios exhibit lower pulp sheet densities and strengths (Syafii and Siregar, 2006).
The flexibility ratio value of mangrove wood fibers analyzed in this study were classified as class II (0.56–0.70), except B. gymnorhiza and B. cylindrica, which fell into class III (0.45–0.49). The flexibility ratio is the ratio of the lumen diameter to the fiber diameter. Fibers with thin walls can easily change shape if they have a high flexibility ratio. The ability of fibers to shape change can improve the contact between the surfaces of the fibers; thus, the bond between each fiber is stronger, producing pulp sheets of good strength quality (Syafii and Siregar, 2006). Conversely, fibers with a low flexibility ratio have a small lumen diameter, resulting in thicker pulp sheets with rough surfaces and lower strength (Husein, 2004).
The rigidity coefficient, which is the ratio between the wall thickness of the fiber and the diameter of the fiber, plays a crucial role in determining paper tensile strength. A lower rigidity coefficient indicates higher tensile strength of the paper, meeting the requirements for pulp and paper raw materials (Syafii and Siregar, 2006). In this study, the rigidity coefficient ranged from classes II–III. Wood species belonging to class II include H. tiliaceus and E. Agallocha, while those in class III include B. gymnorhiza, B. cylindrica, S. ovata, S. caseolaris, and A. alba. Although some mangrove wood species are included in quality class III, their rigidity coefficients are comparable to those of six species of bamboo fibers, which exceed 0.15 (Fatriasari and Hermiati, 2008).
The fiber dimension derivative calculations (Table 4) showed that the wood fibers of H. tiliaceus, A. alba, S. caseolaris, and E. agallocha met quality criterion II. Wood species with fiber quality III included B. gymnorhiza, B. cylindrica, and S. ovata. Sulastri and Rizalinda (2014) suggested that A. marina can be used as raw material for paper, whereas A. alba can be used as a low-quality building material. However, our study shows that A. alba falls into quality class II, indicating its suitability for raw paper materials. This discrepancy may arise because their assessment relied solely on fiber cell wall thickness without considering fiber dimension derivatives. As noted by Mandang (1993), fibers classified as quality class III are not suitable for raw pulp materials, whereas fibers classified as quality class II (medium) are recommended for raw pulp materials.
4. CONCLUSIONS
Based on the quality of wood fiber dimensions assessed in this study, only H. tiliaceus, A. alba, S. caseolaris, and E. agallocha are deemed appropriate for pulp and paper. However, selecting wood species that are appropriate for pulp and paper production requires additional criteria, particularly related to wood chemical properties and plant growth characteristics.