1. INTRODUCTION
Persimmon (Diospyros kaki, family Ebenaceae) is a seasonal fruit-producing tree native to China, which later spread to Korea, Japan, and other parts of the world as a traditional crop and exotic fruit (Choudhary et al., 2022; Nazir et al., 2013; Yuniastuti et al., 2021). Persimmon tree has 15–17 m height unpruned and should not surpass 5–6 m height for cropping purposes (Baswarsiati et al., 2006; Intrigliolo et al., 2018). In Indonesia, persimmon trees are commonly found in several regions, including North Sumatra (Berastagi and Toba), West Java (Garut and Ciloto), Magetan, Malang, and East Java (Batu; Baswarsiati et al., 2006).
Persimmon wood is suitable for high-quality furniture, sculptures, and musical instruments because of its unique color and physical characteristics (Kiaei and Bakhshi, 2014). The heartwood of D. kaki rarely forms black streaks called “kurogaki” in Japan, which grows very slowly and has high density, excellent durability, and ornamental value. This blackened portion of D. kaki wood is used in tea ceremony goods (e.g., alcove posts in Japanese tea rooms), boxes, and other miscellaneous articles in Japan (Iwami et al., 2020; Noda et al., 2002; Ogata et al., 2008; Tazaki et al., 2017). In Indonesia, this species is usually planted for fruit production and is categorized as a slow-growing species (Mayasari et al., 2012). It is important to understand the potential wood utilization after the fruit-producing period ends or when the fruit is no longer productive. Because of the decreasing supply of wood as a raw material for the timber industry from natural forests, species diversification, especially from lesser-used species, is required as a raw material substitution (Augustina et al., 2020).
Wood utilization requires specific characteristics of the wood depending on its purpose. To develop efficient wood utilization, variations in wood characteristics and properties within trees, among trees, and among species must be understood for proper wood utilization (Fadwati et al., 2023; Jang et al., 2019; Zobel and van Buitjenen, 1989). Several wood characteristics such as wood cell proportion, wood cell dimensions, and the presence of juvenile wood are important parameters for determining wood quality (Nugroho et al., 2012; Savero et al., 2020).
Juvenile wood is formed when the cambium is younger, and over time, the cambium forms mature wood (Wang et al., 2021). The beginning of mature wood formation differs among species (Shmulsky and Jones, 2011). Palermo et al. (2015) reported that mature wood formation in Eucalyptus grandis occurs between the age of 8 and 13 year-old, whereas Zanuncio et al. (2022) reported that mature wood formed after 20-year-old cambium age in Pinus caribaea. In addition, Juvenile and mature woods have different anatomical characteristics. Juvenile wood has smaller and shorter fibers, thinner fiber walls, larger microfibril angles, higher lignin content, lower density, and lower strength than mature wood (Darmawan et al., 2013; Lu et al., 2021; Nugroho et al., 2012; Rahayu et al., 2021). Juvenile wood is quite undesirable because of its disadvantageous properties that limit its potential applications in veneer and solid wood products (Darmawan et al., 2013; Dirna et al., 2020; Hadi et al., 2019; Nawrot et al., 2014; Nugroho et al., 2012). The proportion of juvenile wood in the axial direction also varies; it can be cylindrical (Gatto et al., 2013; Zobel and Buijtenen, 1989) or conical in the core of the stem (Yang et al., 1986).
There is limited information on the juvenile and mature wood of D. kaki, such as their boundaries and anatomical properties in the axial direction. This study aimed to provide complete information about the boundary between the juvenile and mature wood of D. kaki along with information on the wood characteristics in the axial and radial directions, so that the utilization of wood can be more efficient.
2. MATERIALS and METHODS
The D. kaki trees in this study were obtained from a smallholder plantation located in Merdeka Village, Berastagi District, Karo Regency, North Sumatra Province, Indonesia (3°11'46.5"N 98°27'19.1"E, 1,375 m asl). Sampling was carried out in September 2019, when the temperature in Berastagi ranged from 19°C to 26°C, humidity was 79%, and rainfall was 2,100–3,200 mm/year.
Moreover, this study uses three 30-year-old selected D. kaki trees, with an average diameter at breast height of 152.8 mm and average height of 6.30 m. Three 30-year-old D. kaki were predicted to have formed mature wood. Bhat et al. (2001) and Nugroho et al. (2012) reported that some species, such as Acacia mangium and teak wood, have already formed mature wood at 23 and 20 years, respectively.
Nine disk samples with a thickness of 50 mm were collected from the base, middle, and top of the stems of the trees at an interval distance of 200 cm (divided based on the branch-free height). The disk samples at the base were collected 30 cm above the ground, as shown in Fig. 1. The disk samples were then sprayed with 30% alcohol and wrapped in aluminum foil for preservation. The samples were observed at the Faculty of Forestry, Universitas Gadjah Mada, Yogyakarta, Indonesia.
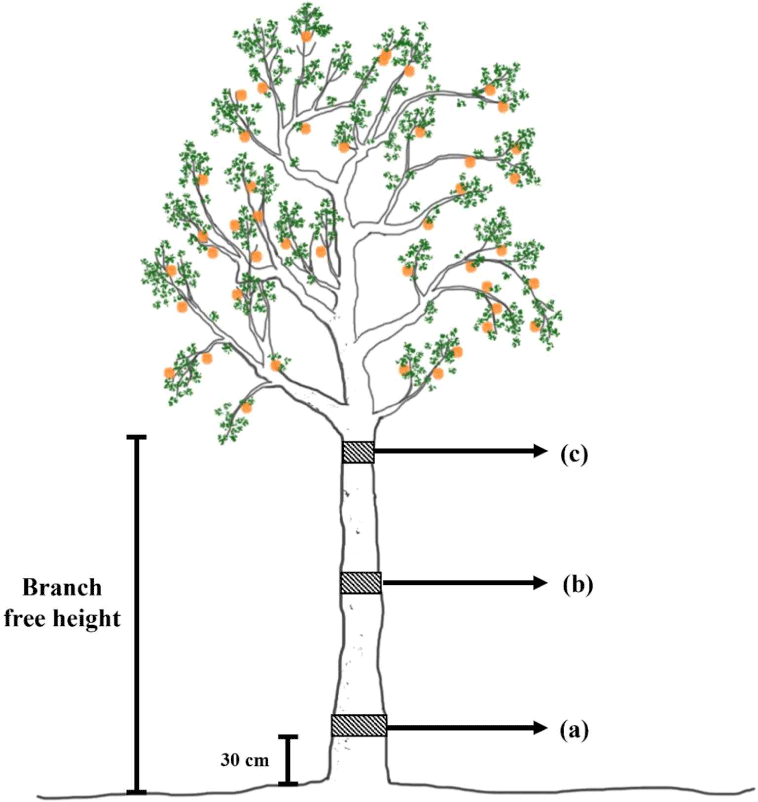
Maceration samples were prepared by cutting the disk (at 0–50 mm from the pith at 5 mm intervals; > 50 mm from the pith at 10 mm intervals) into small pieces in the form of sticks (approximately 1 × 1 × 20 mm3), as illustrated in Fig. 2. The stick samples were macerated with Franklin solution [a mixture of 100% glacial acetic acid (CH3COOH) and 50% hydrogen peroxide (H2O2) in a 1:10 ratio] until they disintegrated into fibers. The macerated fibers and vessel elements were cleaned using distilled water, placed on an object glass, stained with 1% safranin (WAKO Pure Chemical Industries, Richmond, VA, USA), cleared with xylol solution, mounted with resin (Entellan, Merk, Darmstadt, Germany), and covered with a cover glass. Furthermore, the fibers and vessel elements were observed using an Olympus BX51 series light microscope and images were captured using a digital camera (DP70, Olympus, Tokyo, Japan). Fifty cells (fibers and vessel elements) were analyzed using Image-Pro Plus ver. 4.
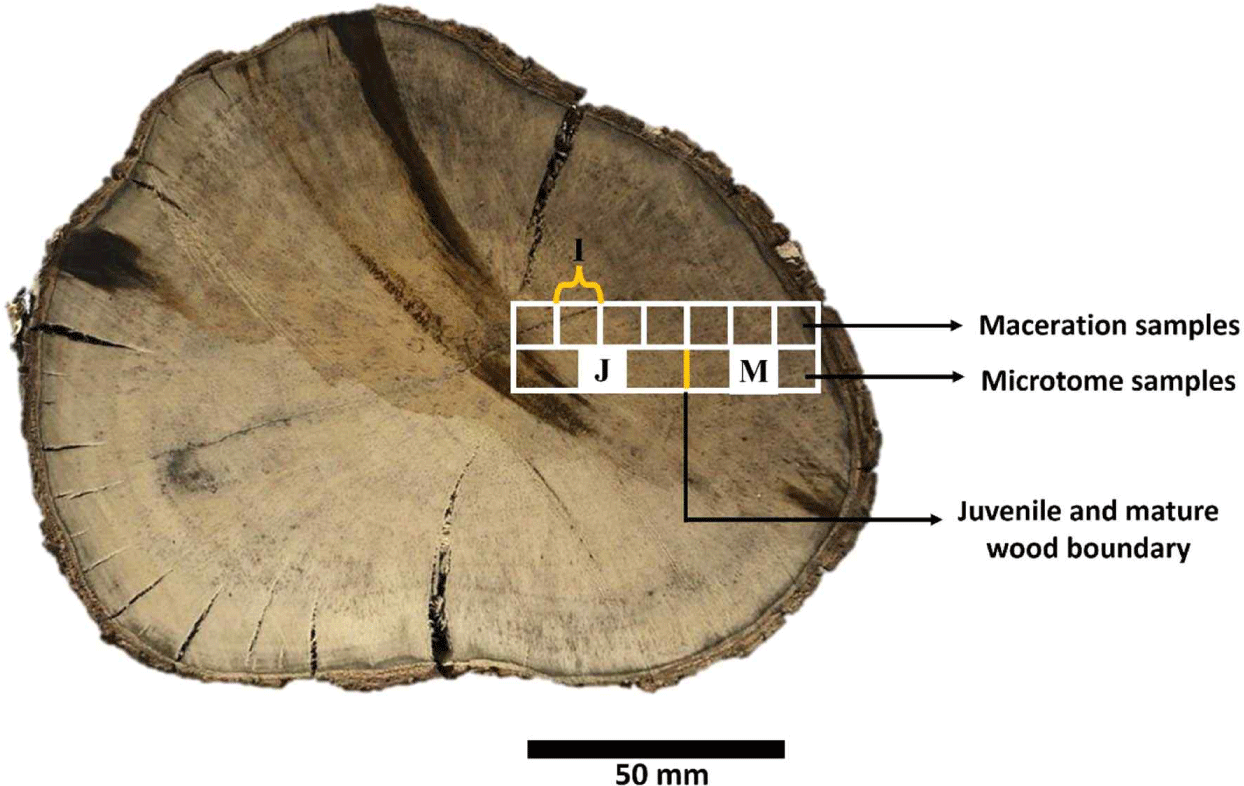
The boundary between juvenile and mature wood was determined by the variation in fiber length (Y) and vessel element length (V) from near the pith to near the bark position (Nugroho et al., 2012), according to the specified interval in this study. Fiber length in the juvenile wood zone rapidly increases and eventually reaches a constant value toward the bark in the mature wood zone (Fujiwara and Yang, 2000; Nugroho et al., 2012). The present study used the vessel element to calculate fiber elongation (Y') because it has a length similar to that of fusiform cambial cells (Kitin et al., 1999). The fiber elongation (Y') was calculated from the difference between fiber and vessel element length. The trend line of the variation in fiber elongation from the pith to the bark position was used to calculate the logarithmic curve of fiber elongation. Furthermore, the increase in wood fiber length (△D) was calculated from the logarithmic curve. The boundary of juvenile and mature wood was measured using a value of 0.3% (Nugroho et al., 2012).
After the boundaries of the juvenile and mature wood were determined, small block samples (1 × 1 × 1 cm3) were taken from each juvenile and mature part to observe differences in their anatomical characteristics. Samples were taken from the middle area of the juvenile and mature wood, as illustrated in Fig. 2. Samples were sliced using a microtome (Yamatokohki, Saitama, Japan) with 20 μm thickness in the transverse section and 15 μm in the radial and tangential sections. The sliced samples were cleaned with distilled water and placed on object glass. The slices were stained with 1% safranin (WAKO Pure Chemical Industries), cleared with xylol solution, mounted with resin (Entellan, Merk), and covered with a cover glass. Samples were observed using an Olympus BX 51 series microscope and images were captured using an Olympus DP-70 connected to the microscope. The parameters (cell diameter, cell lumen diameter, cell wall thickness, cell height, frequency, and proportion) were measured using Image-Pro Plus ver. 4, based on the IAWA List of Microscopic Features for Hardwood Identification (Wheeler et al., 1989). An analysis of variance (ANOVA) was performed using SPSS Statistics ver. 25 to determine the significant differences in fiber length in persimmon wood, the boundary between juvenile and mature wood and its variation in the axial direction, and the anatomical properties of wood in the radial (juvenile–mature) and axial directions.
3. RESULTS and DISCUSSION
The fiber length of D. kaki in the radial direction tended to increase rapidly from near the pith to approximately 40 mm from the pith and then became constant toward the bark, as shown in Fig. 3. This result is in line with those of Marbun et al. (2019), Palermo et al. (2015), and Shmulsky and Jones (2011), where the length of wood fiber increased from the juvenile area near the pith toward the mature area near the bark. Kiaei and Bakhshi (2014) also stated that the fiber length in Diospyros lotus increased from the pith toward the bark. Furthermore, the fiber of D. kaki in this study has an average length of 1.33 ± 0.01 mm, categorized as medium fiber according to Hosseini and Naghdi (2004). D. kaki has a longer fiber compared to other Diospyros species, namely D. lotus with 1.13 mm, D. celebica with 1.04 mm, D. blancoi with 1.19 mm, and D. mespiliformis with 0.98 mm (Asdar, 2017; Kiaei and Bakhshi, 2014; Krisdianto and Abdurachman, 2005). The long fibers of D. kaki could be beneficial because long cells sometimes affect the strength and stability of boards when combined with steep microfibril angles (Zobel and Buijtenen, 1989). In addition, although hardwood fibers are rarely used in pulp and paper manufacturing, long wood fibers are suitable for pulp processing because they affect the weaving power (Lempang, 2019; Zobel and Buijtenen, 1989). Moreover, the axial variation in D. kaki fiber length did not differ significantly among the base, middle, or top of the tree (p = 0.724), as shown in Table 1. The same result was reported by Tavares et al. (2011), Taylor (1973), and Zobel and Buijtenen (1989), where the fiber length was essentially the same at all heights and was not influenced by height. Sseremba et al. (2016) reported that fiber length decreased from the base to the top of the tree.
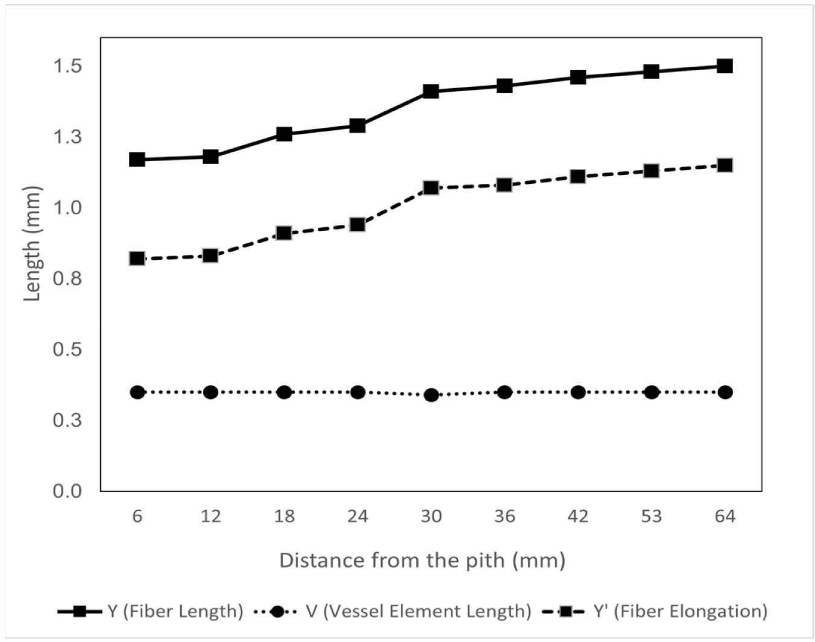
The length of the vessel element was relatively constant and formed a linear pattern with an average of 0.35 ± 0.02 mm in the radial direction (Fig. 3). In addition, the axial variation of the vessel element length was slightly increased from the base to the top of the tree (p = 0.173), ranged from 0.33–0.36 mm, as shown in Table 1. Vessel elements are constant (slightly elongated after differentiation) radially and across the stem, similar to the pattern observed in fusiform cambial cells (Kitin et al., 1999).
The determination of the boundary between juvenile and mature wood was conducted by the calculation of the increase in length of wood fiber, increment rate of fiber length (△D), and logarithmic line of fiber length according to Nugroho et al. (2012), as shown in Fig. 4. The average value of the juvenile and mature wood boundaries in D. kaki was found at 44.11 mm from the pith, as the fiber length became stable with a 0.3% increase in fiber elongation (△D). This result was slightly different from Acacia mangium in Indonesia, where mature wood was formed at 45.8–52.0 mm from the pith (Nugroho et al., 2012). Juvenile and mature wood boundaries vary among species and genotypes and depend on their geographic origin (Boruszewski et al., 2017). These results align with Zobel and Buijtenen’s (1989) statement that the transitional age between juvenile and mature wood varies greatly among trees.
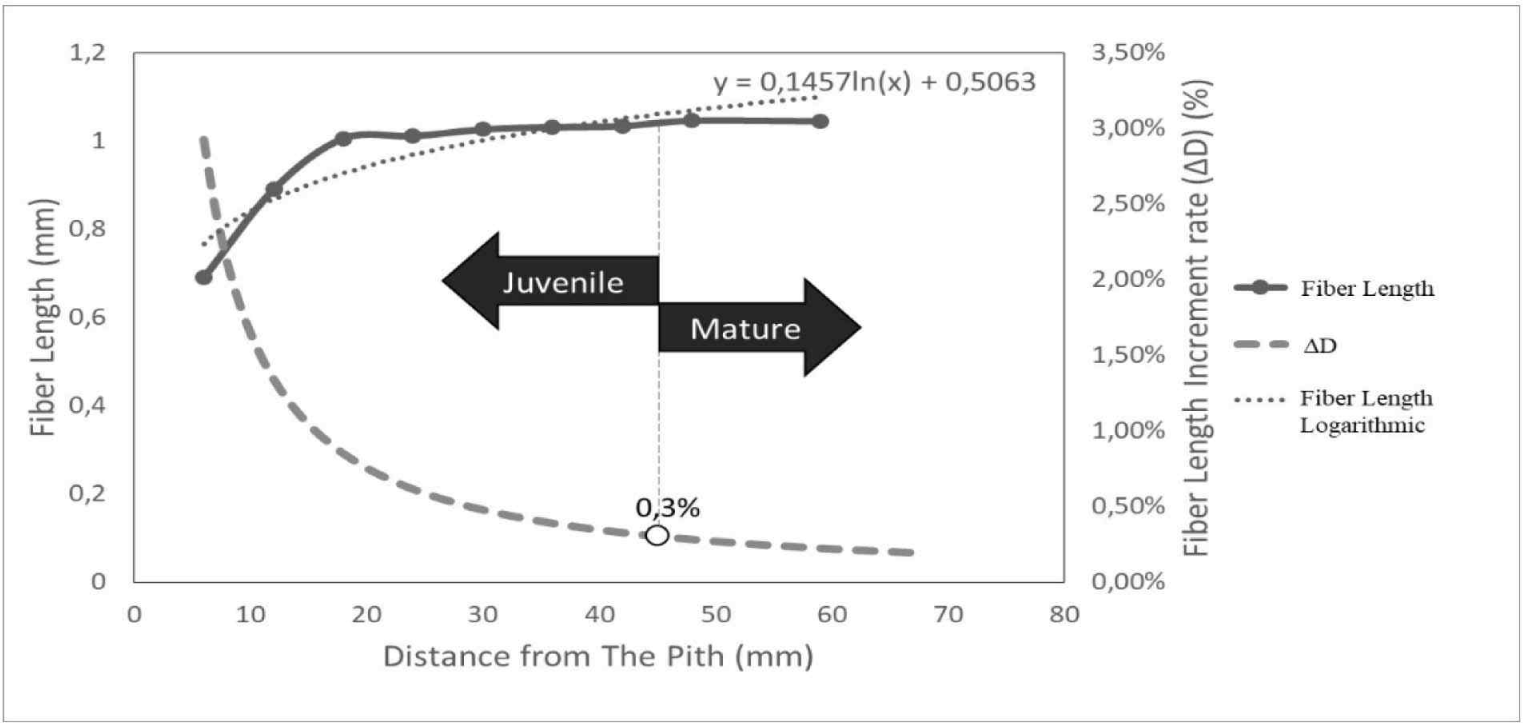
Moreover, the boundary between the juvenile and mature wood showed no significant difference in the axial direction (p = 0.996). The proportion of juvenile wood in the axial direction tended to be constant (44–44.33 mm), and its distribution showed a cylindrical shape with mature wood included at the top of the tree, as indicated by the proportion of juvenile wood that showed no significant difference in the axial direction (p = 0.212), as shown in Table 1. Alteyrac et al. (2006) reported the same results, in which the estimated proportion of juvenile Picea mariana wood along the stem remained constant. The results of the present study are in line with those of Gatto et al. (2013) and Zobel and Buijtenen (1989), in which juvenile wood had a cylindrical shape with a uniform diameter from the base to the top of the stem.
Based on the boundary between the juvenile and mature wood of D. kaki in this study, the proportion of juvenile wood was higher than that of mature wood. The proportion of juvenile wood ranged from 64.18%–71.51%, as shown in Table 1. A high proportion of juvenile wood in trees can be utilized as the central layer of laminated products, such as glulam and cross-laminated timber, without a substantial negative impact on product performance if the drying distortion is not excessive. Furthermore, the impact of juvenile wood on reconstituted panel products, such as medium-density fiberboard and oriented strand board, is smaller than that on solid wood products, where the modulus of elasticity, modulus of rupture, and internal bond are similar to those of mature wood (Moore and Cown, 2017). The quality of wood with poor physical and mechanical properties, such as juvenile wood, must be improved. The properties of low-density wood can be improved through various treatments such as densification and chemical modification (Basri et al., 2023; Dirna et al., 2020; Hadi et al., 2019; Nawawi et al., 2023).
This study showed that juvenile wood of D. kaki has a larger fiber diameter (p = 0.047), higher vessel proportion (p = 0.009), and higher axial parenchyma proportion (p = 0.016) than mature wood, as shown in Table 1. The average fiber diameter in juvenile wood was 17.54 ± 0.21 μm and showed significant differences compared to mature wood, that has 16.25 ± 1.10 μm in the diameter of the fiber. A large fiber diameter is related to specific gravity, where both the fiber diameter and cell proportion affect the porosity of wood (Zobel and Buijtenen, 1989). Juvenile wood of D. kaki has a large fiber diameter and a high proportion of vessels and axial parenchyma, as illustrated in Fig. 5. A high proportion of vessel elements and axial parenchyma increases wood porosity and lowers specific gravity and strength, as they do not contribute to mechanical stability (Morris et al., 2018; Shmulsky and Jones, 2011). In contrast, the proportions of vessels and axial parenchyma decreased from juvenile to mature wood. Palermo et al. (2015) reported the same result in E. grandis, where the proportion of vessels decreased radially.
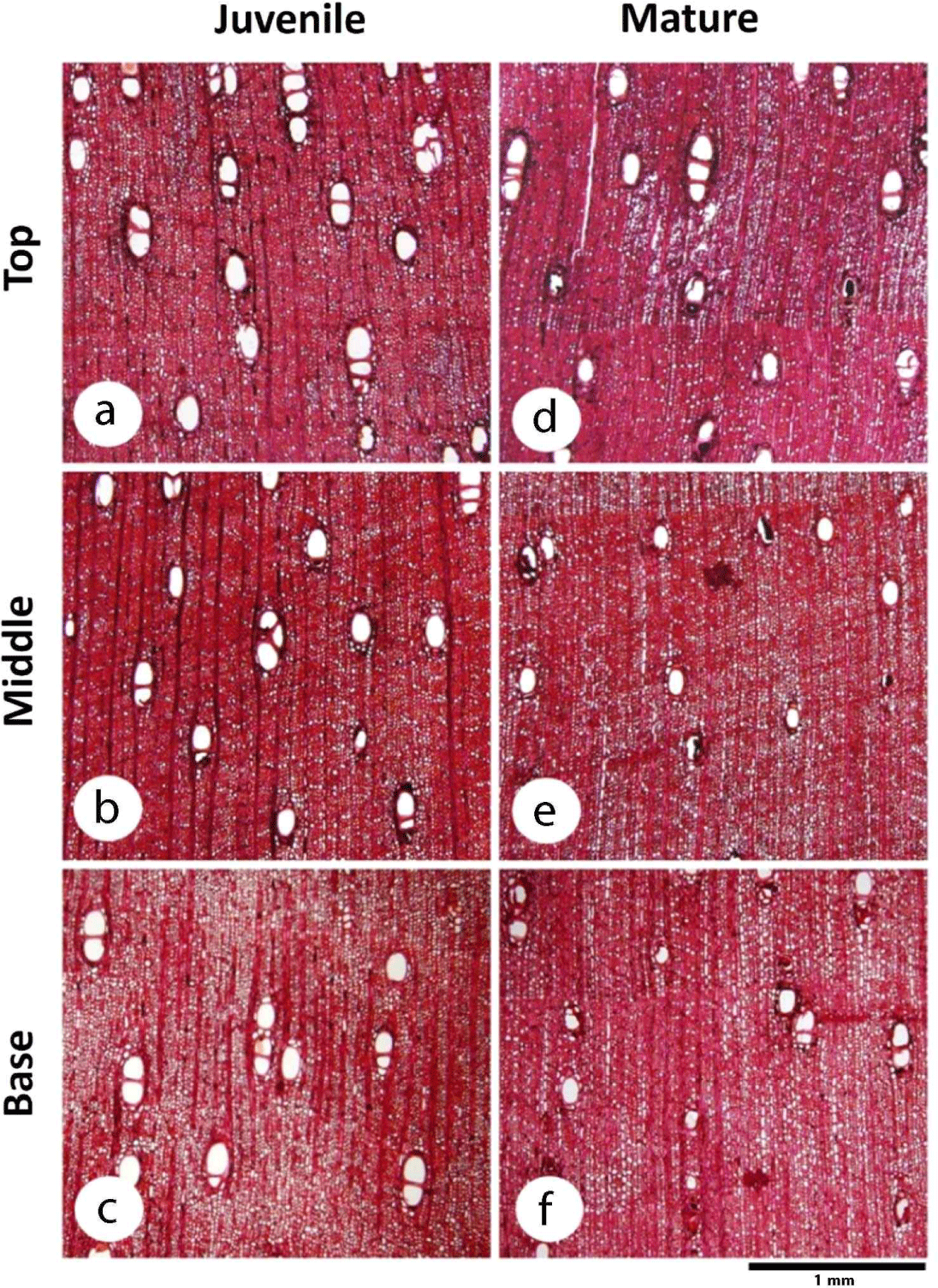
Furthermore, juvenile wood of D. kaki has no significant differences in fiber lumen diameter (p = 0.061), vessel frequency (p = 0.086), ray parenchyma height (p = 0.402), and ray parenchyma proportion (p = 0.074) compared with mature wood. The fiber lumen diameter on juvenile wood of D. kaki was 10.74 ± 0.43 μm and mature wood was 9.34 ± 1.09 μm. A previous study conducted by Asdar (2017) reported that D. celebica fiber lumen diameter decreased radially to the bark. In Shorea parvistipulata, the lumen diameter of the fibers decreases from the pith to the bark (Supartini and Kholik, 2010). In addition, a high vessel frequency and ray parenchyma proportion may increase the porosity of the wood.
The mature wood of D. kaki has a significantly higher fiber proportion (%), with average value of 67.62 ± 1.43 compared to juvenile wood (p = 0.005). Fiber wall thickness (p = 0.151), vessel diameter (p = 0.575), and ray parenchyma frequency (p = 0.307) in mature wood tended to be higher than in juvenile wood, although the differences were not statistically significant. The fiber wall thickness of D. kaki tends to be lower in juvenile wood (3.24 ± 0.15 μm) than mature wood (3.49 ± 0.17 μm), as shown in Table 1 and Fig. 6. The greater fiber wall thickness in the mature wood of D. kaki may have been caused by a thicker S2 layer (Borrega et al., 2015). Palermo et al. (2015) found that the fiber dimensions and tissue composition can predict specific wood properties. Furthermore, thin cell walls formed at the beginning of growth resulted in low-density wood, whereas mature wood with thicker cell walls had a higher density (Rahayu et al., 2021; Seta et al., 2023). High vessel diameter and ray parenchyma frequency in mature wood increase its porosity and considerably affect the quality of wood products. Wider cells often affect paper quality as they provide bulk to the paper. Abundant ray or parenchyma cells affect the quality of solid wood and pulp products, as thin-walled cells contribute little to the strength properties (Zobel and Buitjenen, 1989).
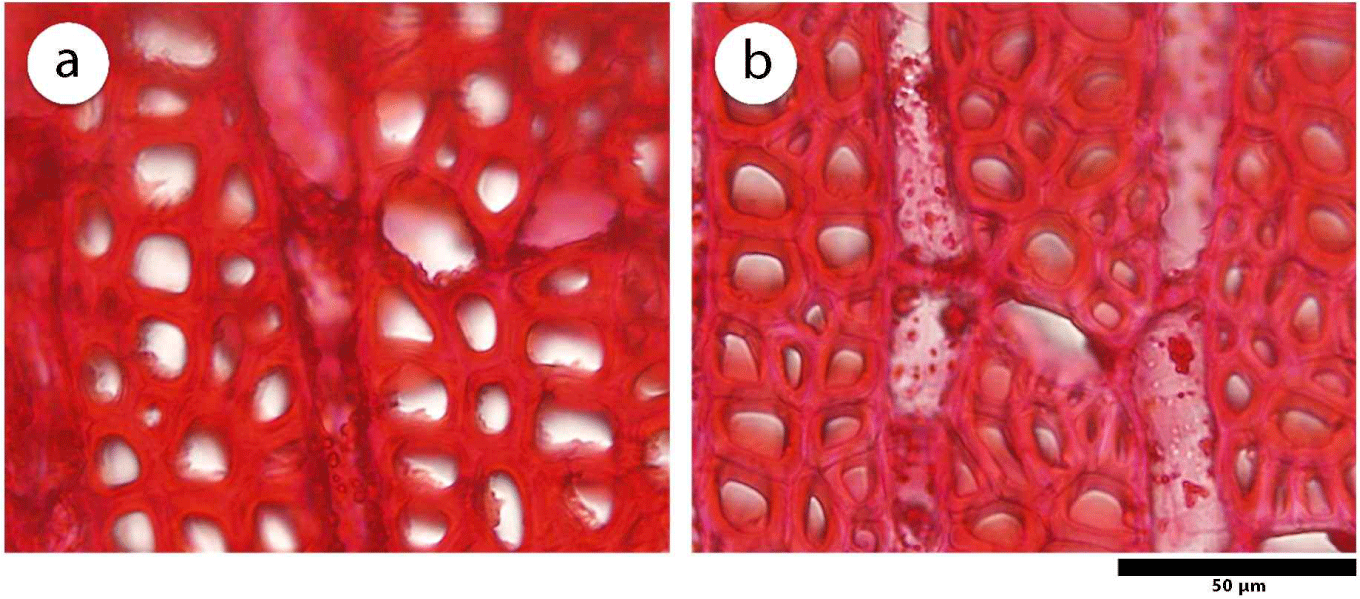
In terms of wood variation in the axial direction, the vessel diameter (p = 0.014), ray parenchyma frequency (p = 0.028), and ray parenchyma proportion (p = 0.047) were significantly different at the base, middle, and top of the tree. The vessel diameter of D. kaki increased from the base to the top in both the juvenile and mature wood. The same result was reported by Noah and Durojaiye (2019), where the vessel diameter increased in the axial direction of Boscia angustifolia. Ray parenchyma frequency increased from the base to the top in juvenile wood and decreased at the top of the tree in mature wood, as shown in Table 1, whereas the proportion of ray parenchyma decreased from the base to the middle and then increased to the top of the tree, both in the juvenile and mature wood of D. kaki. The same result was found in S. parvistipulata, where the proportion of parenchyma of the radius tended to decrease from the base to the middle of the stem and then increase toward the top (Supartini and Kholik, 2010). Wider cells with a high proportion of ray parenchyma cells affect the porosity of wood because they lower wood density. In this study, D. kaki tended to have a lower density at the top of the tree in both juvenile and mature wood.
4. CONCLUSIONS
The average value of juvenile and mature wood boundary of thirty-year-old D. kaki wood was found at a distance of 44.11 mm from the pith as the fiber length became stable, with 0.3% increase in fiber elongation (△D). In the axial direction, the boundary of juvenile and mature wood tended to have the same distance, and its distribution showed a cylindrical shape, with mature wood included at the top of the tree. Furthermore, the juvenile wood of D. kaki had a higher fiber diameter, vessel proportion, and axial parenchyma proportion, with a slightly higher fiber lumen diameter, vessel frequency, ray parenchyma height, and ray parenchyma proportion than mature wood. The mature wood of D. kaki had a higher fiber proportion and tended to have a higher fiber wall thickness, vessel diameter, and ray parenchyma frequency. In the axial direction, the vessel diameter, ray parenchyma frequency, and ray parenchyma proportion had different values at the base, middle, and top of the tree.