1. INTRODUCTION
Lignin is a major component of wood and is the second most abundant biomass source on Earth (Bang et al., 2022; Fatriasari et al., 2020; Iswanto et al., 2021; Lee et al., 2021; Watumlawar and Park, 2023). The lignin is mostly burned as an energy source in paper and pulping industries (Hwang et al., 2021). However, the unique aromatic structure of lignin and the existence of various functional groups, including hydroxyl groups, suggest the possibility of using lignin as a raw material for polymeric materials (Park et al., 2020). In addition, interest in the use of lignin in the field of organic materials is increasing, as the functional properties of lignin, such as antioxidant, antibacterial, and UV properties, have been discovered recently.
There are two strategies for utilizing lignin in the materials industry. First, lignin can be used as an additive by exploiting the functionality of raw lignin materials (Cahyani et al., 2023; Hwang and Oh, 2023; Min and Um, 2017; Pawale et al., 2022). However, this method has the disadvantage that only a small amount of lignin can be used. Second, there is a method of utilizing a large amount of lignin by performing pretreatment and chemical modification to control its nonuniform physicochemical properties of lignin (Chen et al., 2021b; Sangian et al., 2018). In this regard, the value of lignin as a polymer material can be increased by implementing methods such as the organosolve process, controlling the molecular weight and functional groups of lignin through solvent fractionation, and imparting thermoplasticity through polymer grafting.
It has been known that lignin has excellent adsorption capacity for various harmful substances because it has an aromatic backbone capable of π–π bonding, aliphatic functional groups capable of hydrogen bonding, and oxygen-containing functional groups including phenolic hydroxyl groups (Ma et al., 2020). Kim et al. (2022) developed a lignin-based fibrous adsorbent for removing ionic dyes and confirmed that lignin exhibited excellent adsorption capacity for cationic dyes. However, because lignin mainly has anionic functional groups, such as carboxyl and hydroxyl groups, on its surface, it has a poor adsorption capacity for contaminants with negative charges, such as anionic dyes, chromium, and palladium compounds. In addition, most lignin-based adsorbents introduced thus far are composed of powdered lignin materials; therefore, it is difficult to apply them in batch or column processes for water treatment (Behboudi et al., 2021; Fu et al., 2019). In addition, because the use of adsorbents in the powder state requires an additional separation process for the adsorbents from contaminated water, conversion to bulky adsorbents, such as beads, fibers, and hydrogels, is essential.
In this study, the surface of lignin was chemically modified to maximize its adsorption capacity for anionic contaminants. Changes in the physicochemical properties of lignin due to cationization were confirmed through Fourier transform infrared spectroscopy (FTIR), elemental analysis, X-ray photoelectron spectroscopy (XPS) analysis, and lignin elution experiments in a water environment. In addition, a bead-type adsorbent with improved stability in a moist environment was prepared by incorporating cationized lignin into an alginate (Alg) matrix for utilization in a batch-type water treatment process and easy separation of the lignin-based adsorption material from contaminated water. Isothermal adsorption and adsorption kinetics analyses were performed to evaluate the hexavalent chromium [Cr(VI)] removal performance of cationized lignin-containing Alg beads and elucidate the Cr(VI) removal mechanism. Finally, the possibility of sustainable use of cationized lignin-containing Alg beads was evaluated through a reuse experiment.
2. MATERIALS and METHODS
Kraft lignin (KL) was kindly provided by Moorim P&P (Ulsan, Korea). Formaldehyde (37 wt%), polyethyleneimine (PEI, Mw ~800, branched), 1,5-diphenylcarbazide (≤ 98.0%), potassium dichromate (99.5%), sulfuric acid (95%–97%), calcium chloride (≤ 93.0%), and sodium Alg were purchased from Sigma-Aldrich (Yongin, Korea). Sodium hydroxide was purchased from Junsei Chemical (Tokyo, Japan).
KL (5 g) and formaldehyde (1 g) were added to a 50 mL solution of 4 wt% PEI. The pH of the solution was adjusted to 10.0, and the mixture was reacted at 50°C for 5 hours. After the reaction, the pH was lowered to 3.5 to precipitate the PKL. The obtained PKL was washed with distilled water (DW) until the washed water became neutral and then dried in an oven.
The chemical structures of lignin before and after the introduction of PEI were compared using FTIR spectroscopy (Nicolet Summit FTIR Spectrometer, Thermo Fisher Scientific, Waltham, MA, USA) and XPS (Axis Supra, Kratos Analytical, Manchester, UK). FTIR spectra were recorded in the spectral range of 4,000–700 cm–1 with 64 scans and a scan resolution of 4 cm–1 in attenuated total reflectance mode. The atomic ratios of KL and PKL were assessed using an elemental analyzer (Flash EA 1112, Thermo Electron, Waltham, MA, USA). Lignin was mixed with 0.1 M HCl, NaOH, and DW and stirred at 150 rpm for 1 hour to evaluate the water stability of lignin according to pH. The dissolution of lignin in each solution was measured using a UV-vis spectrometer (OPTIZENTM POP, KLAB, Daejeon, Korea) at an absorbance of 280 nm wavelength (Singh et al., 2022), and calculated using Equation (1):
where Aa represents the absorbance of the solution when all the lignin has dissolved, and At is the absorbance of the solution after t min.
Alg/PKL beads were prepared by the ionic gelation of Alg (Fig. 1). PKL was added to a 2 wt% sodium Alg solution to prepare various ratios (1:0, 1:0.5, 1:1.0, 1:1.5, and 1:2.0) of the Alg/PKL solutions. The resulting Alg/PKL solution was added dropwise to a 2 wt% CaCl2 solution using a syringe pump (KDS 100 Legacy, KD Scientific, Holliston, MA, USA). The distance between the surface of the CaCl2 solution and the needle tip was fixed at 5 cm. The sodium ions surrounding the Alg were replaced with calcium ions through a double replacement reaction, and the Alg solidified into beads. The cross-linked Alg/PKL beads were washed twice with DW. The morphological properties of Alg/PKL beads was analyzed using digital camera (Galaxy S10e, Samsung, Suwon, Korea) and field emission scanning electron microscopy (FE-SEM, SUPRA 55VP, Carl Zeiss, Baden-Württemberg, Germany).
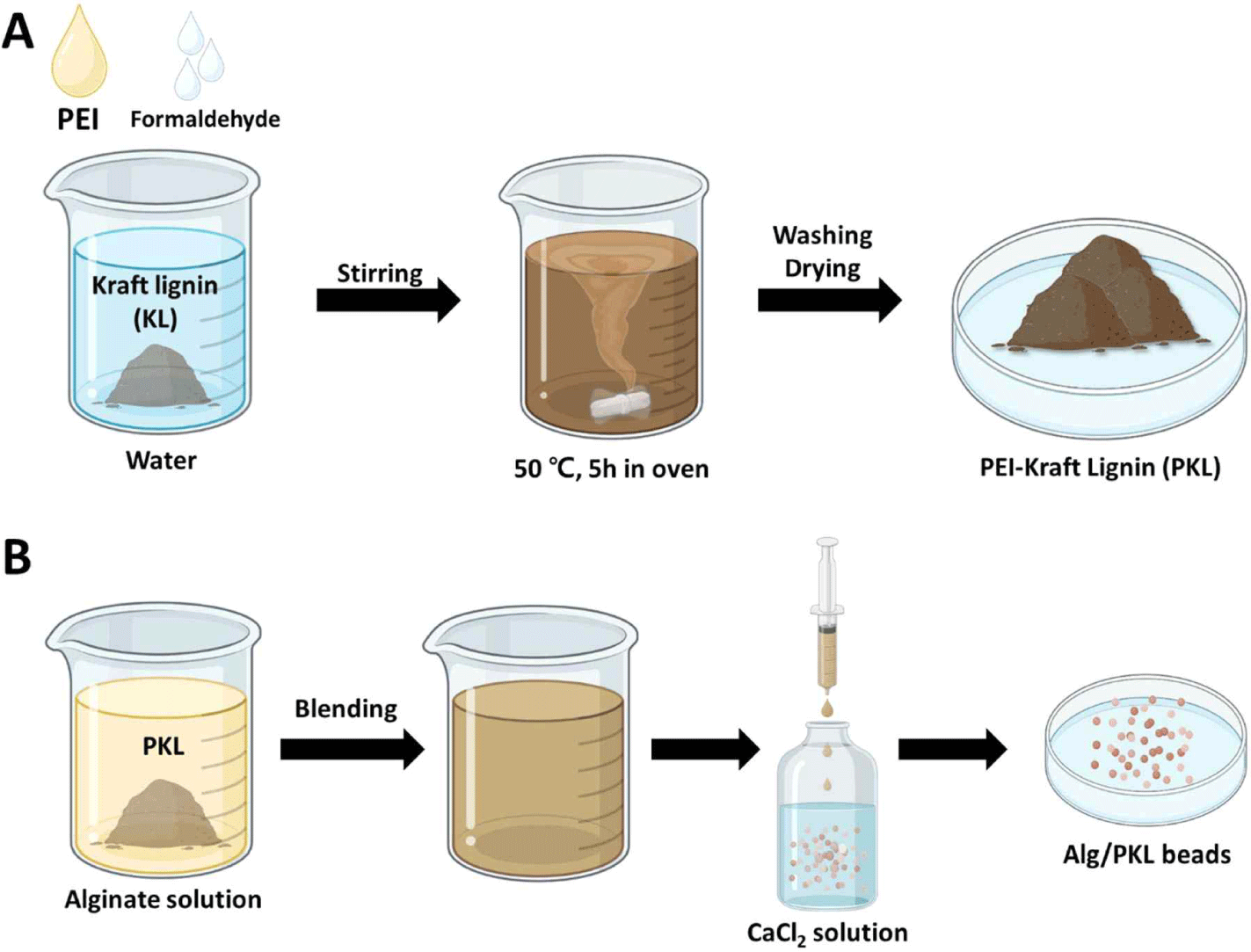
A 1,000 mg/L Cr(VI) solution was prepared using Cr2K2O7 and diluted to concentrations ranging from 10 to 550 mg/L. Alg/PKL beads (50 mg) were added to 50 mL of a Cr(VI) aqueous solution at various concentrations and stirred at 4 g during the adsorption experiment. After adsorption, the concentration of Cr(VI) remaining in the solution was evaluated using a colorimetric method using 1,5-diphenylcarbazide (Lace et al., 2019). For this evaluation, 45 mL of DW, 100 μL of Cr(VI) aqueous solution, 10% (v/v) sulfuric acid, and 1% (w/v) 1,5-diphenylcarbazide solution were mixed for 30 s. The absorbance of the mixed solution was measured using UV-vis spectrophotometry at a wavelength of 540 nm (Hasija et al., 2021). The Cr(VI)-adsorption capacity of the Alg/PKL beads was calculated using Equation (2):
where C0 and Ce are the initial and equilibrium concentrations of aqueous Cr(VI) solution (mg/L), respectively, V denotes the volume (L) of the Cr(VI) aqueous solution, and M is the mass (g) of the adsorbent.
The Cr(VI) adsorption performance of the Alg/PKL beads was evaluated under various adsorption parameters, including different concentrations and time conditions. The adsorption isotherms and kinetic analyses were conducted for each condition. For the adsorption isotherm analysis, the equilibrium concentrations of Cr(VI) in the solution at different initial concentrations were determined, and the data were fitted to various isotherm models, such as Langmuir or Freundlich, to understand the adsorption behavior and determine the maximum adsorption capacity. For the adsorption kinetics analysis, the change in Cr(VI) concentration over time was measured, and kinetic models, such as Pseudo-first-order or Pseudo-second-order kinetics, were applied to determine the rate of adsorption and the mechanisms involved.
The reusability of the Alg/PKL beads was assessed using a 100 mg/L Cr(VI) solution. The Alg/PKL beads, which had adsorbed Cr(VI), were desorbed using a 0.1 M NaOH solution to remove Cr(VI), and then they were added back into a fresh 100 mg/L Cr(VI) solution. The adsorption–desorption cycle was repeated five times to evaluate the reusability of the Alg/PKL beads.
3. RESULTS and DISCUSSION
PEI was introduced to impart a cationic functional group to the lignin surface, and phenol-formaldehyde was used as a crosslinking agent. FTIR analysis was performed to observe the changes in the chemical properties of lignin after cationization. As shown in Fig. 2(A), in the case of PKL, amide bonds at 1,650 cm-1 and C-N bonds at 1,125 cm-1 were newly created, through which PEI was covalently introduced into the lignin surface and contained a large number of amine groups (Wei et al., 2021). Cationization by the introduction of PEI was easily confirmed by a change in composition [Fig. 2(B)]. KL was composed of 67% carbon, 31.9% oxygen, and 1.1% trace nitrogen. In contrast, the nitrogen content increased to 7.4% for cationized lignin, which was attributed to the presence of PEI on the surface. This increase in nitrogen content was also observed in the XPS spectrum, and the introduced amine functional group was confirmed through the high-resolution N 1s spectrum [Fig. 2(C) and (D)]. Nitrogen peaks were not observed in KL, while N- at 398.2 eV and –NH2 functional group peaks at 401.2 eV were observed for cationized lignin (Zhu et al., 2014). Stability in various pH environments is essential in water treatment adsorbents for sustainable utilization through excellent removal efficiency and repeated reuse. In the case of KL, chemical and physical crosslinking processes are required because lignin elution occurs easily via dissolution in an alkaline environment. Elution experiments were conducted under acidic, DW, and basic conditions to examine the effects of the introduction of PEI and the crosslinking process using phenol-formaldehyde on the water stability of PKL [Fig. 2(E)]. In the case of KL, the dissolution rate was less than 30% under acidic and DW conditions, but showed a high dissolution rate of 83% under basic conditions owing to the solubility of KL. However, in the case of PKL, a crosslinking process involving covalent bonding with phenol-formaldehyde was performed, and the elution rate in the base decreased to 26.5%. In addition, the dissolution characteristics of lignin could be effectively controlled by incorporating an additional Alg matrix into the prepared PKL. An adsorption experiment in a 100 mg/L Cr(VI) solution was conducted to examine the effect of cationization on the Cr(VI) removal characteristics of lignin [Fig. 2(F)]. KL showed a Cr(VI) removal efficiency close to 20%, while PKL showed a Cr(VI) removal efficiency close to 100%. This is because the lignin surface exhibits cationization properties through the introduction of a large number of amine groups, thereby maximizing the electrostatic attraction with the chromate anion (Li et al., 2018).
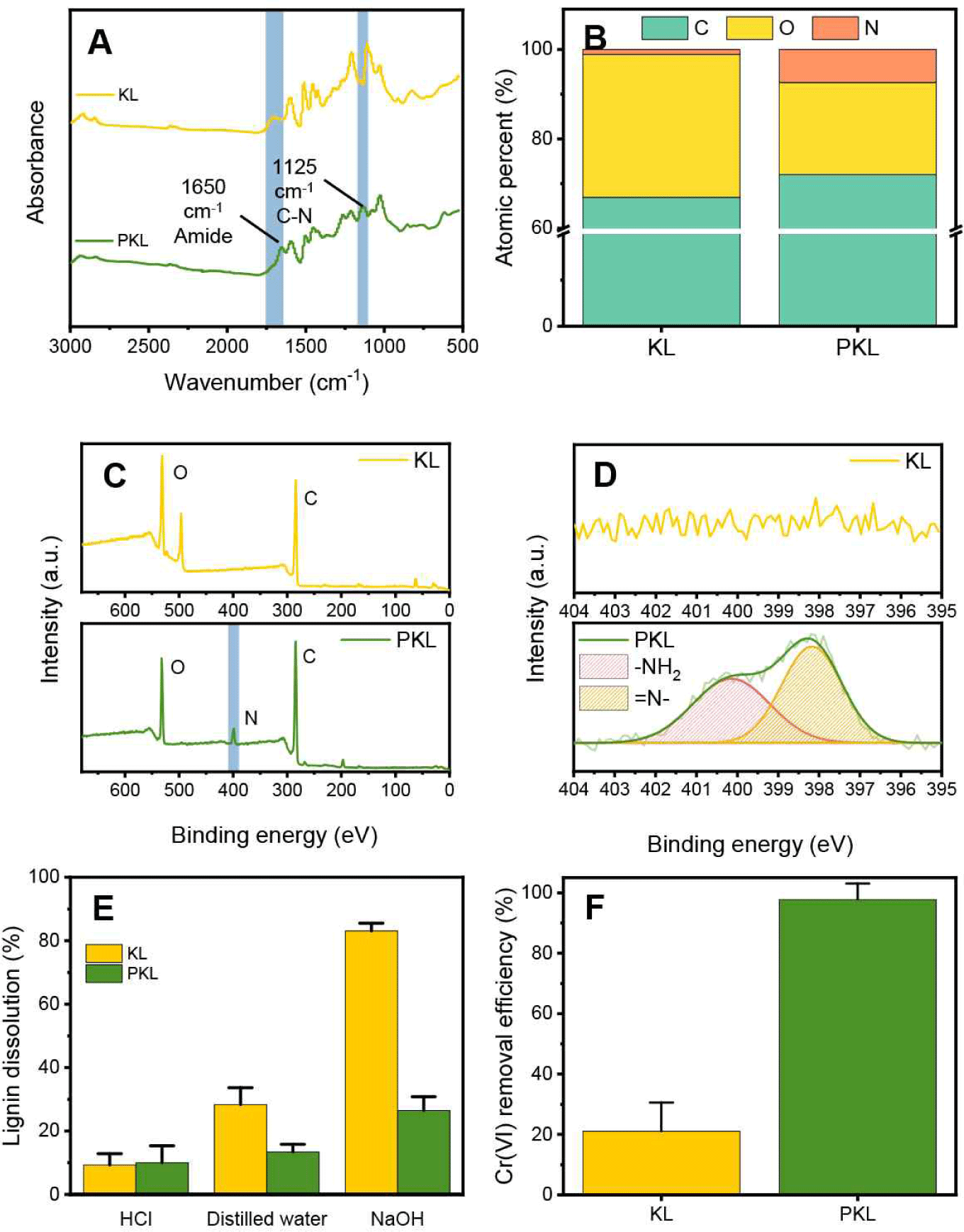
Generally, Alg gelation by adding polyvalent ions, including divalent ions, is a simple process. Calcium-induced gelation is the most popular gelation method for Alg, and it has been shown that an “egg box” structure appears due to a specific and strong interaction between the G block (α-L-guluronic acid) of Alg and Ca2+ (Alnaief et al., 2011). Beads of various Alg/PKL ratios were prepared to select the Alg/PKL ratio capable of maximizing the adsorption capacity of PKL via cationization and minimizing the elution of lignin. In the Fig. 3(A), it was found that Alg/lignin beads were formed up to a 1:2.0 (Alg:lignin) ratio, even with the addition of PKL, indicating that PKL was well incorporated into the Alg three-dimensional matrix. The presence or absence of lignin elution was examined under batch-type adsorption conditions according to the amount of lignin added, and the results are shown in Fig. 3(B). Even when 150% lignin was added compared to Alg, no lignin elution was observed; however, when 200% lignin was added, destruction of beads and elution of lignin were observed in a physically stirred environment. The Cr(VI) removal capacity of the adsorption material was evaluated based on the amount of PKL added. As shown in Fig. 3(C), the Cr(VI) removal capacity increased as the addition amount of PKL increased, and in the case of Alg/PKL beads with 150% addition, an excellent Cr(VI) removal capacity of 537.6 mg/g was observed. However, when 200% lignin was added, no noticeable increase in adsorption capacity was observed, which is thought to be the result of bead disintegration and lignin elution. To confirm the morphology of the Alg/lignin beads with cationized lignin, FE-SEM images of the beads lyophilized using liquid nitrogen were obtained, and the results are shown in Fig. 3(D). It showed the pure Alg beads having a diameter of 800 μm and a pore structure of 100–200 μm. In the case of Alg/lignin beads with 150% added, the size increased to an average diameter of 1,100 μm, which is due to the increase in viscosity according to the addition of lignin. Meanwhile, the pore size was also converted to a dense pore structure with a size of 50–100 μm, and PKL formed microparticles surrounding the Alg network structure. Overall, the amount of added lignin that could minimize lignin loss while maximizing the cationized lignin adsorption capacity was 150%. Therefore, an Alg/lignin ratio of 1:1.5 was selected as the optimal mixing condition and used for additional adsorption experiments.
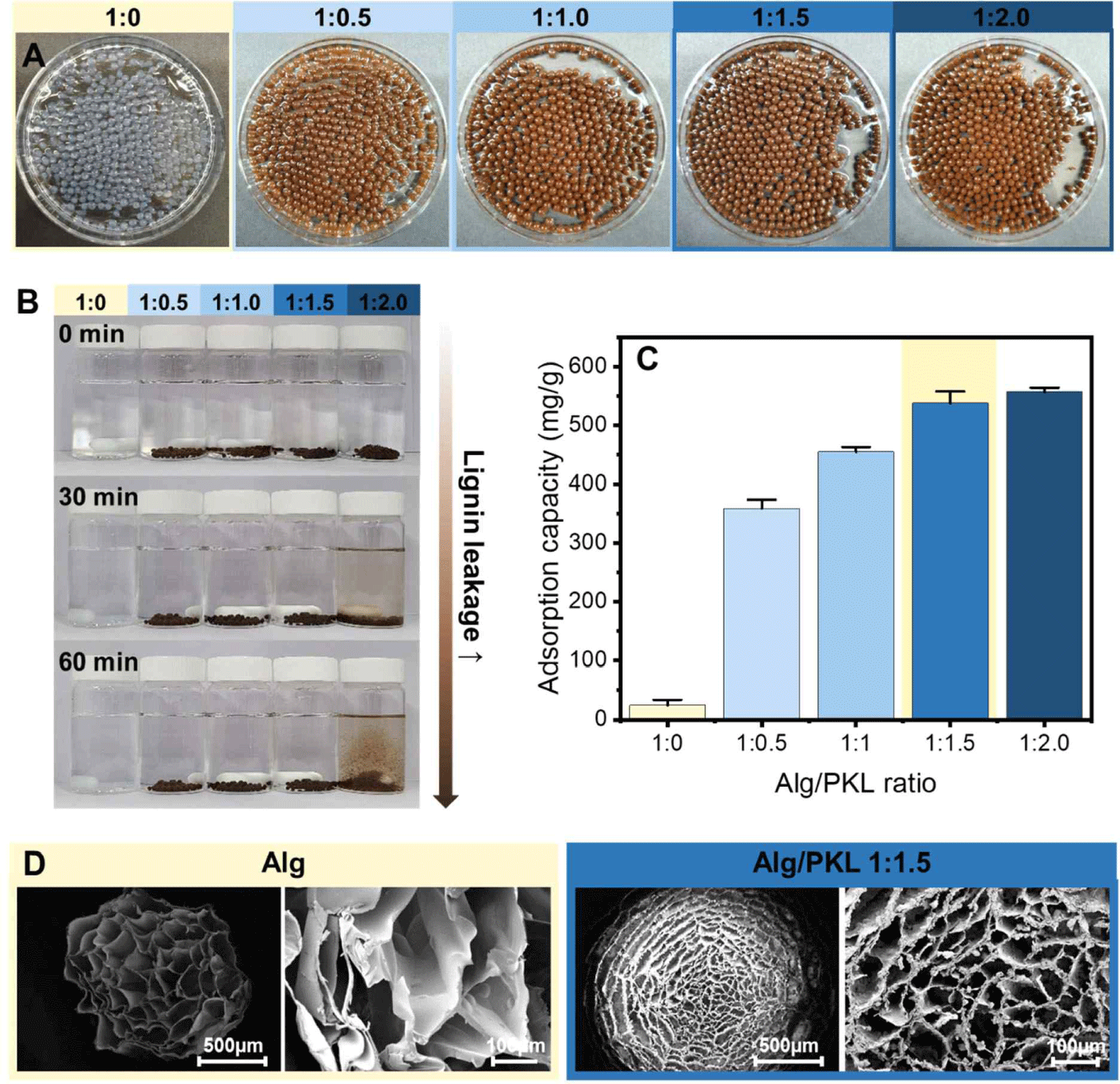
The change in adsorption capacity according to the initial Cr(VI) concentration was evaluated to determine the Cr(VI) removal performance of the prepared cationized lignin-containing Alg beads. As shown in the adsorption isotherms, the adsorption capacity of the lignin-containing Alg beads increased as the initial Cr(VI) concentration increased. This is because the interaction between the surface of the adsorbent and the adsorbate becomes more active as the adsorbate concentration in the limited contaminated water increases. The obtained results were fitted by applying the Langmuir and Freundlich models (Duman et al., 2020; Kwak et al., 2015), which are representative isothermal adsorption models commonly used to describe the interactions between adsorbents and adsorbates. The adsorption isotherm data were fitted using the Langmuir and Freundlich linear models by applying the following Equations (3) and (4):
Equation (3) represents the linear equation for the Langmuir adsorption isotherm, and Equation (4) represents the linear equation for the Freundlich adsorption isotherm. In the equations, qe represents the adsorption capacity at equilibrium, KL is the Langmuir constant, qmax is the maximum adsorption capacity, Kf is the Freundlich constant, and 1/n is the Freundlich exponent of nonlinearity. The adsorption constants obtained from the Langmuir and Freundlich adsorption isotherm equations are presented in Table 1. As shown in Fig. 4(A) and (B), the Freundlich model showed better agreement than the Langmuir model, indicating that the removal behavior of Cr(VI) was heterogeneous. The maximum adsorption capacity of the Langmuir monolayer, obtained by applying the Langmuir model, is a representative performance comparison index for adsorbents. The qmax value of the Alg beads containing cationized lignin was 478.98 mg/g, which was the result of synergistic effects due to the cationic modification of lignin and its incorporation into the three-dimensional Alg network structure.
Parameter | Value | R2 |
---|---|---|
Langmuir isotherm | ||
qmax (mg/g) | 478.98 | 0.924 |
KL (L/mg) | 0.03348 | |
Freundlich isotherm | ||
Kf | 34.75 | 0.993 |
1/n | 0.398 |
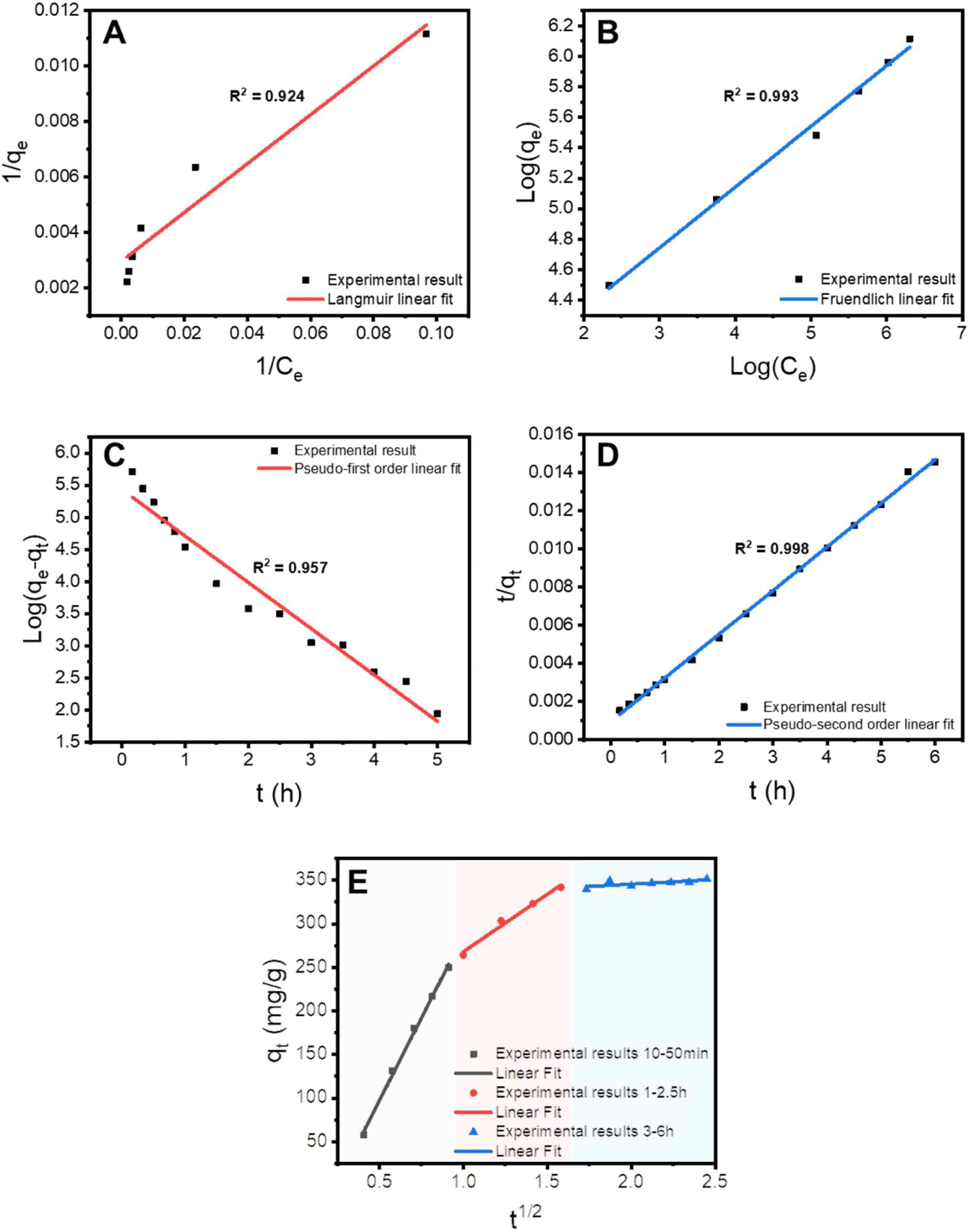
Representative kinetic models, pseudo-first and pseudo-second models, were applied to the results obtained to investigate the adsorption mechanism in more detail. The linear Equations (5) and (6) for the pseudo-first- and pseudo-second-order kinetics models are as follows:
where t is the adsorption time, k1 is the rate constant of the pseudo-first-order kinetics, and k2 is the rate constant of the pseudo-second-order kinetics. The adsorption kinetic constants obtained by fitting are listed in Table 2. As shown in Fig. 4(C) and (D) and Table 2, both the pseudo-first and pseudo-second models showed high agreement, indicating that both physical and chemical adsorption were involved in the removal process of Cr(VI). The R2 value for the pseudo-second-order model was 0.998, indicating an exceptionally good fit. Most cationically modified biomass-based adsorption materials are more suitable for the pseudo-second-order model (Kwak et al., 2020), indicating that the chemical-adsorption-based Cr(VI) removal mechanism is more strongly expressed. The adsorption kinetics results confirmed that a chemical bond was formed between the surface of the Alg beads containing cationized lignin and Cr(VI) contaminants (Chen et al., 2021a).
C0 (mg/L) | Pseudo-first order | Pseudo-second order | ||||
---|---|---|---|---|---|---|
k1 (h–1) | qe (mg/g) | R2 | k2 (g/mg/h) | qe (mg/g) | R2 | |
1,000 | 1.66 | 228.54 | 0.957 | 2.29×10–3 | 436.68 | 0.998 |
An adsorption experiment was conducted in a 1,000 mg/L Cr(VI) solution, and the change in adsorption capacity over time was measured to understand the principle of Cr(VI) removal behavior of the prepared lignin-based adsorbent. As shown in Fig. 4(E), the Cr(VI) adsorption behavior of Alg/PKL beads can be divided into three stages. In the first stage, the Cr(VI) ions diffuse from the bulk solution to the external surface of the adsorbent. Subsequently, the Cr(VI) ions that reached the external surface of the adsorbent underwent intraparticle diffusion and penetrated the interior of the particle. Finally, they reach the internal active sites of the adsorbent. The adsorption behavior of the Alg/PKL beads demonstrated the typical adsorption process of porous adsorbents. This observation was consistent with the FE-SEM image in Fig. 3(D).
Designing an optimal desorption process during adsorption to ensure continuous reusability is essential. The PKL-containing Alg beads adsorbed with Cr(VI) were immersed in 0.1 M NaOH to perform a desorption process, and repeated adsorption and desorption experiments were conducted to evaluate the reuse efficiency. In the initial one-time reuse environment, the reuse efficiency was close to 90%, as shown in Fig. 5. However, as the adsorption number increased, the continuous Cr(VI) adsorption efficiency decreased. After five reuse cycles, a low reuse efficiency of 46.6% was observed. This is because an alkaline environment, which is a desorption environment, can induce swelling of the Alg beads, and additional dissolution of Alg can also occur (Zhuang et al., 2016). For this reason, the desorption of Cr(VI) adsorbed in the basic environment and the elution of lignin occurs, and the adsorption efficiency decreases according to the number of reuses. Therefore, when an additional crosslinking process capable of minimizing the loss of Alg in a basic environment is proposed, it is believed that the reuse efficiency can also be improved.
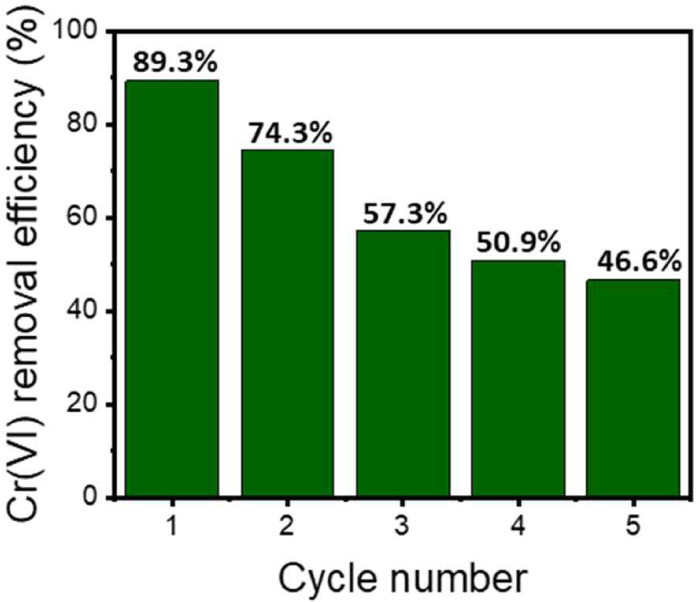
4. CONCLUSIONS
In this study, lignin, a component of lignocellulosic biomass, was cationically modified and processed into beads, which strengthened its interaction with anionic chromate ions, to develop an adsorption material to remove Cr(VI). Using cationic PEI as a functional material and phenol-formaldehyde as a crosslinking agent, a large number of amine groups could be introduced to the surface of lignin, and the water stability was also increased due to crosslinking. However, when lignin was incorporated into the Alg matrix, the amount of lignin required to maximize Cr(VI) removal while minimizing the loss of lignin was found to be 150% of the weight of Alg. The Alg beads containing the addition of cationized lignin showed an excellent Cr(VI) maximum removal capacity of 478.98 mg/g, owing to the cationic modification of lignin and its successful incorporation into the Alg network and pore structure. However, the efficiency decreased rapidly as the number of reuses increased in the reuse experiment, which was due to the structural instability of Alg under basic conditions, that is, the desorption environment of Cr(VI). Therefore, the potential use of lignin in the water treatment field will expand if a lignin incorporation technology with improved structural stability that guarantees stability under acid/base conditions, which is the adsorption/desorption condition of Cr(VI), is developed in the future.