1. INTRODUCTION
Wooden cultural heritages (WCHs) are directly exposed to meteorological events (e.g., torrential rains, heat waves, and heavy snow) at different times of the year as they are located outdoors (Chung et al., 2015; Kim and Chung, 2015). Accordingly, the woody members of these cultural heritages and various organic cultural heritage items stored inside the WCHs are exposed to various sources of degradation during different seasons (Choi et al., 2022; Lee and Bae, 2021). The main deterioration factors encompass meteorological factors (e.g., sunlight and rainfall), biological factors (e.g., plants, animals, and microorganisms), and natural phenomena (e.g., natural disasters). Among them, temperature (T) and relative humidity (RH) are highly related to meteorological conditions, greatly affecting the germination and growth of various airborne fungi inside and outside WCHs. In particular, in the Korean Peninsula with four distinct seasons, when high internal T and RH conditions are created in summer, and simultaneously the air quality inside the space is stagnant, the germination and growth of rot fungi and imperfect fungi, which gain nutrition from organic cultural heritages, cause biological damage to the materials of the heritage structures (Ham et al., 2021; Hong et al., 2018, 2019; Lee et al., 2022; Nandika et al., 2023; Priadi et al., 2021, 2023; Yun et al., 2021). For example, in the 1990s, Seosagan-Jang, one of the storage places for Tripitaka in Haeinsa Temple, had poor ventilation, causing high RH during the rainy season every year and continuous mildew growth on the surface of several printing wood blocks stored inside (Park, 2011). In an environment of continuous rainfall and exposure to ultraviolet rays and soil, wooden structural members are at high risk of biological degradation by microorganisms, regardless of whether they are open to visitors (Kim and Park, 2013). Under conditions where the germination and growth of such airborne fungi inside heritage structures repeatedly occurs at specific periods over a long time, physicochemical weakening and aesthetic discoloration of the materials of the cultural heritages would occur, as well as a higher risk of secondary damage from pests, e.g., termites (Im and Han, 2021; Lee et al., 2015). Therefore, environments in which movable cultural heritages are located should first be considered for their conservation, with environmental improvements aimed at enhancing the long-term preservation of their materials (Hong et al., 2015).
In this regard, cultural heritage-related institutions in Korea, such as the National Research Institute of Cultural Heritage (NRICH) and the Research Institute of Buddhist Cultural Heritage (RIBCH), have targeted WCHs of Buddhist temples that store cultural heritages made of organic materials, such as statues of the Buddha, hanging paintings, and woodblocks. These institutions have investigated the conservation of these environments, such as year-round T and RH control of indoor and outdoor spaces and monitoring airborne microbes, since 2010 (NRICH, 2017, 2019, 2021, 2023). However, the research on the T and RH of WCHs has only been conducted on data collected over a year, leading to difficulties in deriving meaningful results regarding managing internal environments. In addition, the basic plan and research projects related to climate change by the Cultural Heritage Administration (CHA) have only indicated the establishment of a management system for disaster prevention (e.g., fire) facilities inside and outside WCHs, and there has never been a study on conservation environments (CHA, 2012, 2017, 2020; NRICH, 2010). Although NRICH researched the distribution and diversity of airborne fungi inside WCHs in the late 2010s (Hong et al., 2015, 2018, 2019), their studies were only an introduction to their current status; there has been no study evaluating the risks of damage from environmental factors (i.e., temperature and relative humidity).
Conversely, European countries have implemented climate change impact assessments of cultural heritage structures since the early 2000s. This work focused on the prevention and reduction of damage to cultural heritages, the prediction of disasters that are highly likely to occur due to climate change impacts, selecting damage types, and strategically responding to the disasters and damages (Kim and Kim, 2021). Among these early studies, under the NOAH’S ARK project in which pan-European countries conducted research on cultural heritage and climate change from 2004 to 2007, there was a study on the biological damage of wood due to changes in RH, as an early research theme of WCH (Sabbioni et al., 2006). According to the recently revised UNESCO Climate Change Policy Document, it was strongly recommended that member countries should develop a risk assessment tool for cultural heritage structures against climate change and create a risk monitoring framework for climate change adaptation by 2030 (UNESCO, 2021). In Korea, efforts should be made to quantitatively identify the seasonal environmental suitability for conservation inside WCH structures and prepare countermeasures, where necessary. Furthermore, it is necessary to conduct studies assessing the relationship between environmental conditions and the germination and growth of airborne fungi mainly found inside heritage structures rather than limiting studies only to identifying airborne fungal species.
Therefore, this study attempted to identify seasonal patterns of T and RH inside WCHs as a foundational study for the conservation environment diagnosis to suggest a quantitative measure to diagnose their environmental suitability for conservation. Using a climograph, this study also identified the periods of highest risk for damage from airborne fungi inside WCHs.
2. MATERIALS and METHODS
We divided Korea into the northern, central, and southern regions to examine internal conservation environments seasonally and identify risk periods for damage from airborne fungi based on latitude. We conducted T and RH surveys while targeting the WCHs of five temples selected based on latitude: Cheonglyeonsa Temple (northern region) in Yangju; Ansimsa Temple in Cheongju and Jikjisa Temple in Gimcheon (central region); and Muwisa Temple in Gangjin and Heungguksa Temple in Yeosu (southern region). Most climate change-related studies have evaluated year-round climate change patterns by dividing a year into more convenient traditional three-month intervals, i.e., Spring (March–May), Summer (June–August), Autumn (September–November), and Winter (December–February). However, since the latter half of the 20th century, rapid urbanization and global warming have led to earlier starting dates for spring and summer and later starting dates for autumn and winter (Choi et al., 2006). In addition, the climate of the Korean Peninsula for approximately 100 years (from 1912 to 2020) showed an apparent increase in the annual minimum temperature (NIMS, 2021). Accordingly, we classified the data of the monthly average T and RH inside the five WCHs into Winter (January–March), Spring (April–June), Summer (July–September), and Autumn (October–December) based on recent changes to the climate of the Korean Peninsula. The survey was conducted for approximately 18 months, from July 2021 to January 2023.
We utilized Real-Time Monitoring (RTM; HSC-RTM, HSC, Cheongju, Korea) system, an integrated museum storage conservation environment monitoring system of the Heritage Science Center, Chungbuk National University, to identify seasonal trends of changing T and RH inside WCHs. The system was designed to collect environmental factor data inside WCHs located in forests by establishing a Wi-Fi Independent Network in the module itself with only a 220V power supply (Im et al., 2021). The real-time T and RH sensors installed in the Wi-Fi zone, where security settings were implemented, were designed to send measured values to the data server every 10 minutes. Then, the measured data stored in the data server was configured to be textualized and visualized in real-time by the staff in charge, using the internet via PC and smartphone applications. The T and RH sensors were installed to measure four points inside the WCHs in each cardinal direction. We calculated the monthly average T and RH based on the average of the 10-minute measurement values at each point.
As for the internal environmental suitability for conservation created per period, we used the Performance Index (PI) to quantify the data within the range of appropriate T and RH per period, among the total measured data, as a percentage. Considering the wood constituting the WCHs and the organic artifacts stored inside, we calculated the T and RH range based on the standards (T: 20 ± 4°C, RH: 50 ± 10%) for organic materials recommended by the National Museum of Korea. This method is frequently used to evaluate the conservation risk levels of artifacts/collections in museums, libraries and the performance of environmental controls in buildings (Corgnati and Filippi, 2010; Corgnati et al., 2009; Lee et al., 2013).
Previous studies showed that the primary airborne fungi inside WCHs in Korea were Aspergillus sp. and Penicillium sp. (Hong and Jung, 2009; Hong et al., 2011, 2018, 2019). Such fungi are known to be capable of germination and growth even at relatively low humidity levels (Pitt, 1975) and are called xerophilic fungi. The species with the highest desiccation resistance were known to record a value of 0.60–0.65. Most xerophilic fungi can survive and grow without dying in external environments by accumulating intrasolutes that allow cells to maintain high osmotic pressure in a dry state (Irwin, 2020). To estimate the risk period of damage from xerophilic fungi, identified as the main persistent fungi inside WCHs throughout the year, we put the monthly average T and RH measurement values inside the five WCHs into a climograph. The potential area of growth and germination of xerophilic fungus per T and RH, as defined by Lee (2016), as indicated in the graph to identify high-risk periods during the year.
3. RESULTS and DISCUSSION
The average monthly T inside the WCHs decreased to an annual low during January, then increased continuously from March to July, the warmest month. After that, it gradually decreased from August to December of the following year, following seasonal changes. Even though there is a slight difference in the T inside the assets throughout the year, we found a trend that the same patterns were repeated per period according to seasonal changes (Fig. 1).
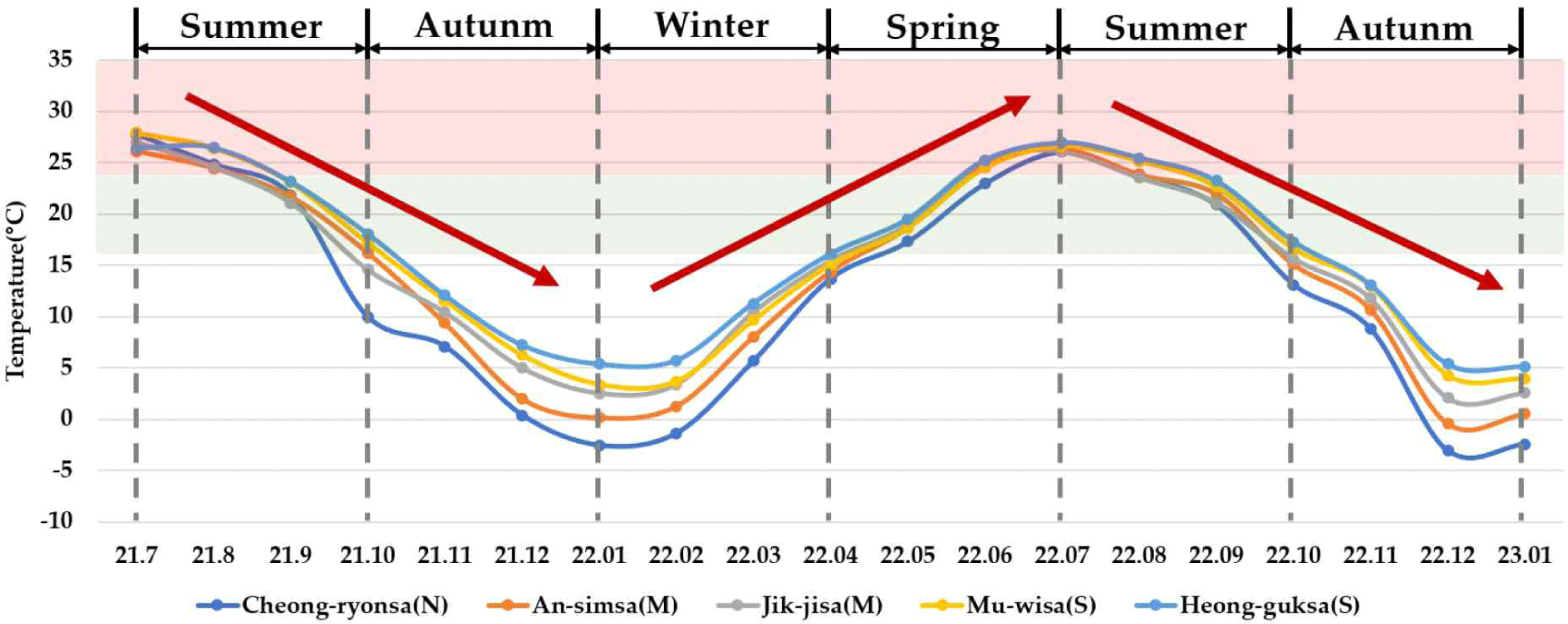
The variation of the average monthly T was confirmed to differ according to the latitudinal location of the WCHs. Cheonglyeonsa Temple in the northern region was located at a relatively higher latitude than Heungguksa Temple and Muwisa Temple in the southern region, and the former showed the largest decrease in internal T during winter. In January, the coldest month, the northern and southern regions showed the largest T difference (approximately 5°C or higher). The internal T of Ansimsa Temple and Jikjisa Temple, located in the central region, had values intermediate of the northern and southern sites throughout the year. On the other hand, July, the warmest month, showed the smallest T difference between all regions (less than 1°C–2°C). As a result, Cheonglyeonsa Temple in the northern region showed the largest T range of 30°C (–3°C–27°C) over the course of the year, whereas Heungguksa Temple in the southern region had a T difference of approximately 20°C (7°C–27°C) over the course of the year, indicating greater annual variation in average monthly T at higher latitudes.
The monthly average RH inside the WCHs rose from a low in February to 50%–65% in March to May, and 75%–80% from July to August, regardless of latitude. After that, from October, all regions gradually decreased until February of the following year, regardless of latitude, and again reached the lowest point of the year. We confirmed that the year-round RH inside the WCHs increased and decreased equally, regardless of latitude, according to seasonal changes, within 40%–80% (Fig. 2).
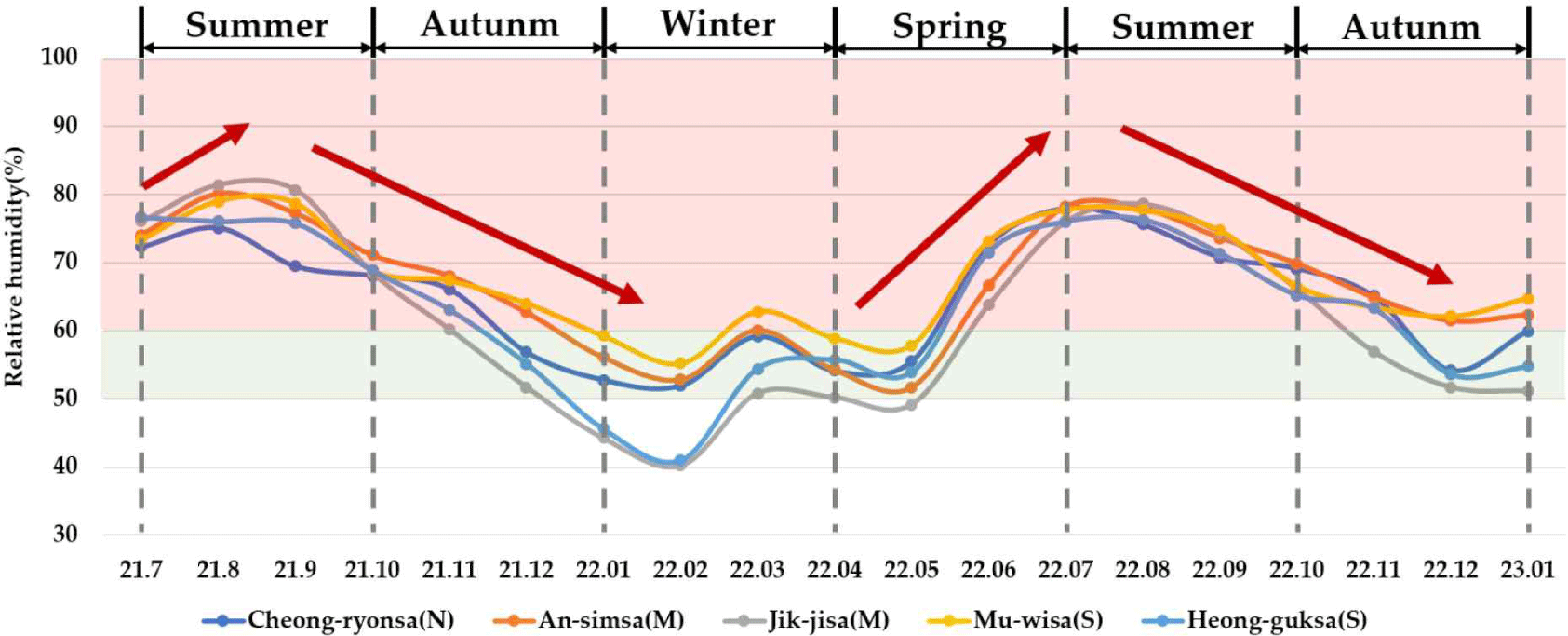
Jikjisa Temple in the central region and Heungguksa Temple in the southern region showed a relatively larger variation of year-round RH inside the WCHs during winter compared to the other three temples. However, Muwisa Temple in the southern region showed the smallest variation throughout the year due to the highest internal RH, even in winter. During the summer season, July and August, the RH inside five temples was approximately 75%–80%, showing the smallest inter-site difference throughout the year (Fig. 2). The RH in the central region (Jikjisa Temple and Ansimsa Temple) was higher than that in the southern region (Heungguksa Temple) in summer (August–September). Furthermore, in winter (November–December), the RH inside the temples in the northern region (Cheonglyeonsa Temple) was higher than those in the central (Jikjisa Temple) and southern regions (Heungguksa Temple). The difference in RH per site was also confirmed even in the same region. In February, Muwisa Temple in the southern region recorded an average internal RH of approximately 55%, the highest among the five WCHs. However, Heungguksa Temple, located in the southern region, recorded 40%, showing a difference of 15% or higher compared to Muwisa Temple; Heungguksa Temple recorded the lowest RH, along with Jikjisa Temple. It was also confirmed that Ansimsa Temple and Jikjisa Temple in the central region had different RH values (10% or higher), indicating that the RH in winter can differ significantly, even at the same latitude. Considering this, unlike T, we could not find any consistent RH patterns by latitude.
The PI value (the value of environmental suitability for conservation) of the average monthly T inside the temples was 58.3 ± 4.1% in spring (April–June), creating the most stable environment throughout the year. In summer, when the T increases to the highest levels of the year, the value was 40.2 ± 10.9% in 2021 and 46.9 ± 7.8% in 2022, confirming that an appropriate conservation environment was maintained during the 30%–55% period over the entire period. In summer, the SD of PI values per temple was highest, at 10.9% in 2021 and 7.8% in 2022, compared to other seasons. We confirmed that the PI value for the internal T decreased by approximately 1% or less in winter (January–March), as the external air T declined as the season changed from summer to autumn and winter. The internal T gradually decreased from spring (the season in which the highest value was recorded) to winter, then rose sharply again in the following spring (the lowest value recorded in this season). Therefore, it was possible to clearly distinguish the difference in PI values for the T inside the WCHs per period, confirming that the appropriate conservation environments were created in the order of spring > summer > autumn > winter (Fig. 3).
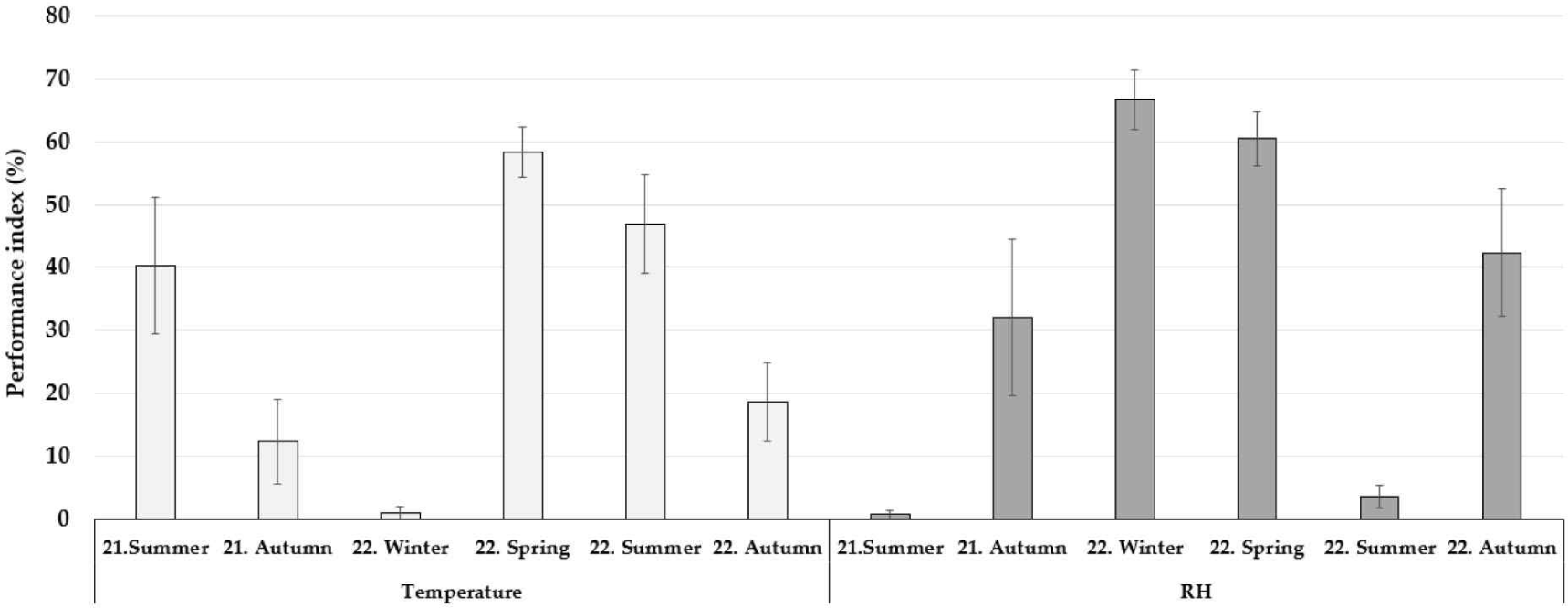
The PI values of the monthly average RH per season were 66.7 ± 4.8% in winter (January–March) and 60.5 ± 4.3% in spring (April–June); those two seasons showed PI values of 60% or higher. In addition, the deviation of the PI values among the five WCHs was within 5%, indicating that the latitudinal differences were small. Autumn (October–December) showed the highest PI value deviation per target site, ranging between approximately 20%–50% (i.e., 32.1 ± 12.4% in 2021 and 42.4 ± 10.1% in 2022). On the other hand, summer (July–September) recorded the lowest PI values throughout the year (0.6 ± 0.6% in 2021 and 3.5 ± 1.8% in 2022), indicating that the appropriate RH environment was not created inside the assets at that time. The PI values of internal RH decreased rapidly as the season changed from spring to summer and rapidly increased along with the seasonal changes from summer to autumn. As a result, the PI values of the RH inside the assets confirmed that appropriate conservation environments were created in the order of winter > spring > autumn > summer (Fig. 3).
The average internal RH in winter was considered appropriate by confirming the potential period of germination and growth of xerophilic fungi based on the monthly average T and RH values inside five WCHs per latitude. Still, since the T was low in winter, the season located farthest from the possible growth zone of xerophilic fungi (Fig. 4). From March, compared to February, the internal T rose sharply to approximately 6°C–7°C and RH to approximately 6%–10%. During autumn (from October to December), the internal RH was maintained within the range of 60%–70%, but from October, compared to the summer period (September), the internal T decreased sharply by approximately 7°C, and then it moved to the range outside the potential growth zone of xerophilic fungi. Summer was located in the risky growth zone of xerophilic fungi, as the internal T was 20°C or higher, and the RH was higher than 70% from July to September in both 2021 and 2022. Furthermore, we found that the average values from July to September in summer were clustered relatively precisely compared to the other seasons. We also found the possibility of damage caused by the growth of xerophilic fungi, as the T was approximately 25°C and RH was 70% in June during the spring season (April–June). Therefore, we found an environment appropriate for the germination and growth conditions of xerophilic fungi that were created inside the assets for four months (June to September; Fig. 4).
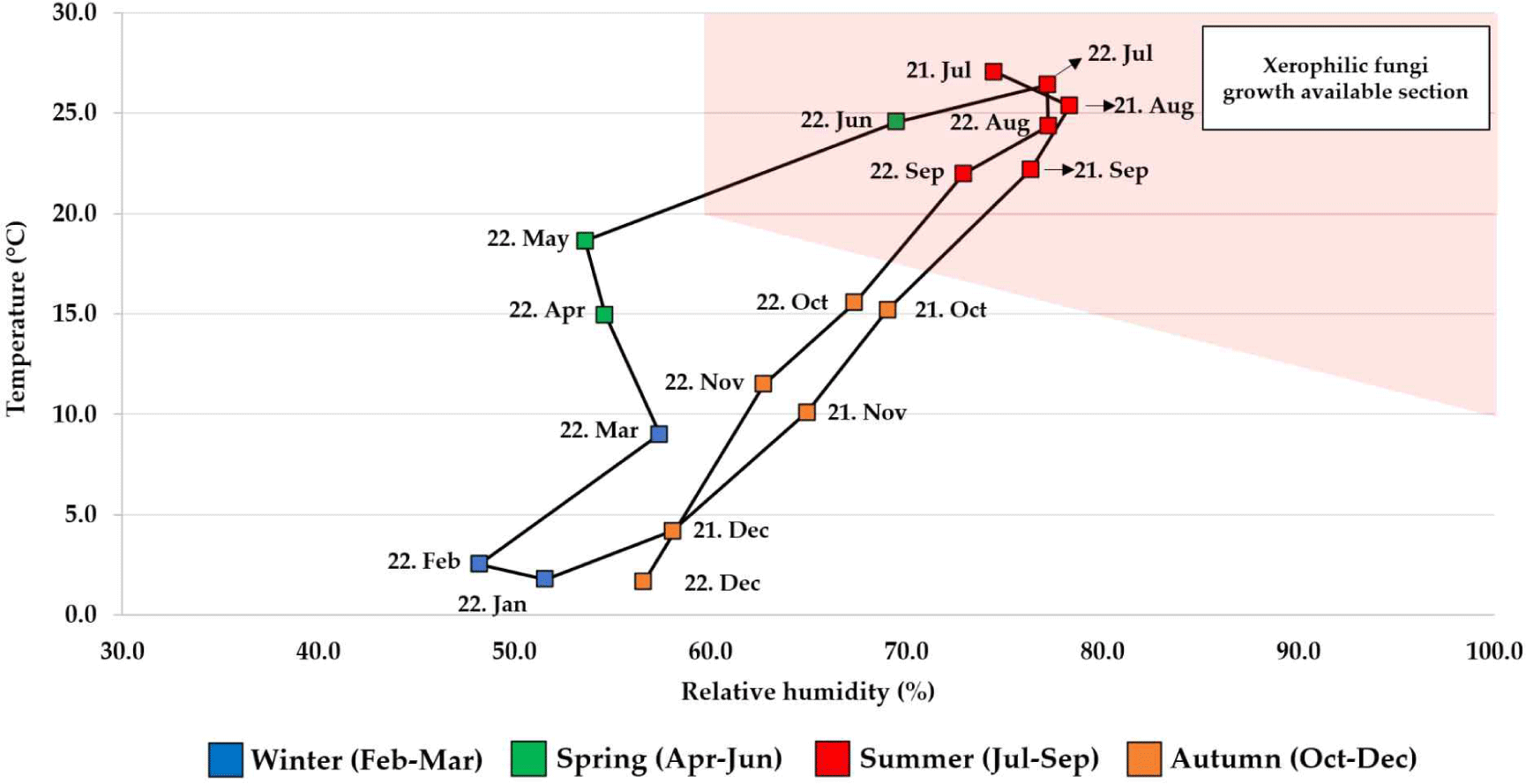
4. CONCLUSIONS
Like Im et al. (2021), this study used a real-time IoT (Internet of Things) environment monitoring system for cultural heritage, which was applied in spaces with easy access to data communication networks (e.g., museum storage) while targeting WCHs in suburbs and forests with difficult accessibility. This approach enabled us to automate the graphs displaying the seasonal T and RH change trends inside the heritage structures and seasonal PI values. More diverse conservation environment surveys will be possible in the future by adding additional sensors to the T and RH sensors used here.
We confirmed that the average T inside the five WCHs per period was lowest in the coldest month (January) and highest in the warmest month (July): the rise and fall were repeated around the same periods every year, regardless of latitude. The pattern of the annual internal T inside the WCHs was consistent with the trend of annual internal T changes in WCHs in several regions [e.g., Juhamnu Pavilion and Yeonghwadang of Changdeokgung Palace (the palace of the Joseon Dynasty), and Buddhist temples such as Beopjusa Temple or Seonamsa Temple; Kim et al., 2015, 2017]. This pattern of year-round T changes is because Korea belongs to the Eurasian continent and is affected by monsoons, such as northwesterly winds in winter and southeasterly winds in summer (Kim and Jhun, 1992). The year-round monthly T changes were consistent with the monthly average change trends in outdoor T throughout Korea, confirmed at 61 points across the country (Choi et al., 2006). In contrast, as for the internal average RH, the RH inside the WCHs increased to a maximum range of 75%–80% in July and August, regardless of latitude, starting from the lowest value in February. This trend of changes in year-round RH inside the assets is also consistent with the results of seasonal internal RH measurements of various WCHs across the country conducted by NRICH (2020, 2021, 2023). Regarding the outcome, Kim et al. (2015) compared internal RH impacted by the opening and closing of WCHs during the rainy season in summer. They reported that lowering RH via the opening was insignificant due to climatological reasons and the high humidity outside during this season. The summer RH shows an increasing tendency because the Korean Peninsula has four distinct seasons and belongs to the monsoon climate zone, where 70% of the annual average precipitation is concentrated from June to September (Hwang et al., 2022; Lee et al., 2011; Song and Kim, 2022). After summer, the internal RH gradually decreased from October to February of the following year, regardless of the region. This seems to be attributable to the decrease in external RH, due to the monsoon of the dry Siberian air mass. As the average winter T on the Korean Peninsula has also risen over the past 100 years due to climate change, we assume that the trend of decreasing average RH outdoors in winter has also had an impact (Ko and Lee, 2013; NIMS, 2021). Therefore, the T and RH inside the WCHs were significantly affected by the climatological changes in the Korean Peninsula throughout the year. The regular operation of air conditioners and environmental monitoring should be carried out in summer and winter to reduce sharp changes repeated annually to a minimum level and to maintain the T and RH inside the assets at an appropriate and consistent level throughout the year.
The annual T variation inside the assets per latitude was approximately 20°C in the southern area (Heungguksa Temple, Muwisa Temple) and approximately 30°C in the northern area (Cheonglyeonsa Temple), indicating that the higher latitude showed greater variation. The year-round monthly T differences based on these latitudinal differences are consistent with the findings of previous studies comparing WCHs (i.e., Beopjusa Temple in the central region and Seonamsa Temple in the southern region; Kim et al., 2017). During the latter half of the 20th century, winter was shortened, and the starting dates of spring and summer advanced in the Korean Peninsula. Over the past 30 years, the range of external T increases in winter and spring has been the most prominent throughout the year (Choi et al., 2006; NIMS, 2021). Therefore, at higher latitudes, organic cultural heritage repeatedly experiences large T changes throughout the year, and the risk of physical and chemical degradation of the materials is presumably greater. However, considering that RH showed large seasonal differences, even within the same latitude, it is assumed that latitude did not have a meaningful impact on the humidity. In particular, the difference in internal RH was largest in winter, and there were cases where the internal RH differed by 10% to 15%, even in the same region. This is assumed to be due to heating systems operating for religious activities inside the heritage structures in winter.
Generally, at below-zero Ts in winter, the internal RH drops due to the decrease in the air’s ability to retain water vapor and the increase in the moisture absorption rate of organic materials such as wood. Under such circumstances, if the air is dried further by operating the heating systems, the internal RH is lowered further. Therefore, supplementation of water vapor is essential to compensate for the RH environment inside the dry heritage structures in winter. Air conditioning without considering such a point could potentially cause irreversible damage to the heritage structures by drying them further (Han and Lee, 1999). Therefore, the RH of WCHs should be managed, as well as examining climatic factors of the surrounding areas, and seasonal management plans for staff in charge of each asset should be developed and implemented.
PI values of T and RH inside the assets throughout the year showed clear differences depending on seasons. In spring, the highest PI value was measured with a T of 58.3 ± 4.1% and RH of 60.5 ± 4.3%. On the other hand, in summer (July–September), the T was 40%–50%, and the RH was lower than 5% (Fig. 3). As shown in Figs. 1 and 2, it was assumed that the rainy season influenced the creation of high-T and high-RH environments inside the heritages. In autumn (October-December), the PI value of RH, which was low in summer, showed a tendency to rise to 60% or higher rapidly. This is assumed to be attributable to the internal RH falling within the appropriate environmental range for conservation, as the external air T and RH, which had risen in summer due to seasonal changes, decreased (Figs. 1 and 2). However, in winter (January–March), influenced by the dry and cold monsoons of the Siberian air mass, the internal T was maintained below 10°C throughout the entire period, resulting in an extreme drop in the PI value of the T to 5% or lower (Fig. 3). Therefore, despite the high PI value of RH, an appropriate environment for conservation could not be created due to the low PI value of T. The inside of WCHs was confirmed to be only a maximum of approximately 60% of the environmental suitability for conservation throughout the year. As a result, it was assumed that air conditioning should be installed to maintain appropriate T and RH levels in all seasons. Regarding this, Kim (1981) indicated that it would be challenging to conserve all cultural heritage items inside WCHs in an ideal conservation environment, due to the great number of materials and the heritage items and their contrasting importance. However, Kim (1981) mentioned that since damage to any item can happen during internal storage, it is necessary to prepare appropriate conservation facilities, and at the same time, to conduct science-based conservation inspections to check whether there are any changes to the items regularly.
Furthermore, T and RH measurements inside WCHs per latitude were converted to PI values and graphed. This approach enabled us to quantitatively identify the status of appropriate conservation environments inside WCHs instead of the previous approach in which the measured T and RH data were shown in a line or bar graph. However, it was impossible to clearly explain the reason for the increase or decrease of the internal environment’s suitability for conservation depending on seasonal changes, based on the only PI value-based graph for each season. Accordingly, we examined the current status of and diagnosed the conservation environments inside the WCHs using a line graph (Figs. 1 and 2) of the existing trend of T and RH changes and a bar graph showing the PI values (Fig. 3). The calculation and utilization of these two types of data to identify the status of WCHs and prepare management plans for creating appropriate year-round T and RH environments in various regions of this country.
As a result of confirming the possible period of germination and growth of xerophilic fungi, the average internal RH was considered to be at an appropriate level in autumn and winter, but the T was low, leading to a lower risk of damage from xerophilic fungi. On the other hand, in spring (June) and summer (July–September), the internal environments of WCHs were maintained with a T of 20°C or higher and RH of 70% or higher (Fig. 4). June satisfied the standard of summer defined by the NIMS (2021): ‘the first day when the daily mean T rises above 20°C and does not drop below this level again’. Accordingly, as June met the environmental conditions of summer (i.e., approximately 25°C in T and 70% in RH), the germination and growth of xerophilic fungi were confirmed to be possible. This is assumed to be attributable to the trend that the average T increase rate in spring over the past 109 years has risen to 0.26°C per decade due to climate change impacts such as global warming (NIMS, 2021). Fungi in cultural heritage structures grow at approximately 4°C–37°C, and their optimum growth happens around 20°C (Roe and Han, 1996). Such germination and growth of fungi require all three conditions (i.e., suitable nutrients, T, and RH). In this sense, the materials of cultural heritages are the nutrients; thermophiles, mesophiles, and psychrophiles exist according to different T ranges, considering that the RH within conservation environments becomes the largest factor determining microbial damage. Based on these standards, the inside of the WCHs in Korea, in which the environment with a T of 20°C or higher and an RH of 70% or higher is maintained for four consecutive months (June–September), is assumed to provide suitable conditions for xerophilic fungi, the airborne fungus, to germinate and grow while gaining nutrition from the materials of cultural heritage structures.
In particular, this study found that as for the internal T and RH during July–September, the five heritage structures at different latitudes were all found in high-T and high-RH environments (Figs. 1 and 2). Considering this, it is clear that four specific months of the year (June–September) were the periods with the highest risk of damage from xerophilic fungi in the country, regardless of latitude. Therefore, during the corresponding period, regular monitoring and alternatives should be needed to lower the high RH. There should also be more studies on the germination and growth characteristics of xerophilic fungi on the different main organic materials of cultural heritage structures, considering T and RH conditions, while targeting xerophilic fungi, which were identified in previous studies as being among the major airborne fungi inside WCHs. Results from studies identifying growth features for each species would enable proper damage prevention and countermeasures against xerophilic fungi according to internal environmental conditions.