1. INTRODUCTION
Environmentally-friendly building materials have gained global interest as a way of response to climate change. Wood has become popular as a building material because it releases 15%–20% less carbon than concrete and steel whose carbon emission and embodied energy are high, and as it absorbs and stores carbon dioxide (Chang et al., 2017). Recently, the use of laminated wood materials has increased as the main structural element in plyscrapers. Cross-laminated timber (CLT) is an engineered wood product manufactured through cross-lamination of at least three plies. Notably, using CLT in lieu of building materials with high carbon emission allows energy saving and carbon reduction (Liu et al., 2016). In Canada, where many ongoing studies are investigating CLT construction methods, the number of cases using CLT in high-rise buildings has increased to highlight CLT as an alternative environmentally-friendly building material for multi-story buildings (Choi et al., 2021). Using CLT, compared to ferroconcrete structures, the architectural cost and construction time can be reduced, and it is well-known that the CLT construction exhibits outstanding vertical - horizontal resistance. Ceccotti et al. (2013) reported on excellent seismic performance of timber frames constructed with CLT, and Muszyński et al. (2019) reported that CLT-based floor panels satisfied the criteria of 2-hour fire resistance performance in the ASTM E119 standard. Yoo and Lee (2019) explored the potential of low-grade timber in CLT production for more efficient use of their domestic wood. While heavy timber structures require the design to separate the beams, posts, and shear walls according to the design load, CLT can be used in walls, floors and roofs to necessitate the performance data on the applied load for each structural material based on the purpose of use. The factors that influence the strength properties of CLT include the tree species, grade, and percentage of knots in the lamina as well as the thickness ratio and fiber angle and the adhesion condition (Buck et al., 2016; Gong et al., 2019; He et al., 2021; Kim, 2020; Pang and Jeong, 2019; Sikora et al., 2016; Song and Hong, 2018; Song and Kim, 2022; Trisatya et al., 2023). To enhance the strength performance of CLT, numerous studies are testing the use of bamboo trees for lamina and examining the hybrid CLT using concrete or fiber-reinforced plastic (Galih et al., 2020; Jiang and Crocetti, 2019; Jung et al., 2020; Yang et al., 2021). There are also studies evaluating the strength performance by applying an engineered wood material rather than sawn wood for CLT laminae as an efficient alternative use of wood materials (Choi et al., 2018, 2020, 2021; Fujimoto et al., 2021). Various strength tests have also been conducted on the ironware such as screws that are used in construction as the method of connection has become important with the increased height and scale of wood buildings (Ahn et al., 2021; Lee et al., 2021, 2022). Currently, the quality criteria on CLT varies in each country according to the main tree species and their characteristics. The methods of specification and quality performance tests are also different. In the case of CLT production, the methods are divided into those based on the major-minor axes as in North America (ANSI/APA PRG 320, 2019) and those based on the external-internal layers as in Japan (JAS 3079, 2013). This causes variations across predicted values in the design of CLT. Additionally, each country has its own method of evaluating the bending performance in varying conditions such as the distribution of defects in CLT.
Thus, this study aimed to determine the effects of the lamina grade and composition conditions on the strength performance of the Larix kaempferi CLT (Larix CLT) and for this, bending and compression strength tests were performed. Through experiments, the differences in bending strength performance were compared according to the CLT lamina composition condition, and the applicability was verified through the prediction equation on the bending performance. Furthermore, the failure modes according to CLT composition in varying load conditions were compared.
2. MATERIALS and METHODS
The test materials used in this study were the L. kaempferi (Lamb.) Carrière [140 (B) × 30 (H) × 3,600 (L) mm] laminae purchased from a Timber Distribution Center in Yeoju-si, Gyeonggi-do, Korea. The average air-dry density and moisture content were 569 kg/m3 and 10.7%, respectively, for the L. kaempferi laminae used in this study. The laminae for CLT production were selected first through visual quality inspection, then a machine stress grading (MGFE-251, IIDA Kogyo, Komaki, Japan) was used to measure the modulus of elasticity (MOE) to define the grade based on the KS F 3020. For instance, the lamina grade E12 should have 12 GPa ≤ MOE < 13 GPa. The grade was set for 704 laminae in total. In the grade distribution result for L. kaempferi laminae (Fig. 1), E12 demonstrated the highest level in the distribution (16.5%). The grades E11 and below accounted for 28% of the total.
The CLT made of L.kaempferi was produced through cross-lamination of five plies. According to the CLT grade and composition, two CLT types were generated: 1) The major-minor type (A type) through cross-lamination that combined three laminae of layers in major direction and two laminae of layers in minor direction; 2) The external-internal type (B type) through cross-lamination that combined two laminae of external layers and three laminae of internal layers that apply to all layers excluding the external ones. Based on the grade distribution of laminae, E12 and E16 were used for the layer in major direction and the external layer, and E9 and E10 were used for the layer in minor direction and the internal layer. In reference to Table 1, Larix CLTs were produced based on two types according to composition condition and two types according to grade configuration to generate four types in total. For the adhesive, Phenol-Resorcinol-Formaldehyde was used, and the physical properties of the adhesive are given in Table 2. The spread rate was 200 g/m2 and the press pressure was 1 MPa for 24-hour press. Curing was performed for a week in a constant-temperature constant-humidity chamber with temperature at 20℃ and relative humidity at 65%. The same temperature and relative humidity were applied in performing the bending strength test.
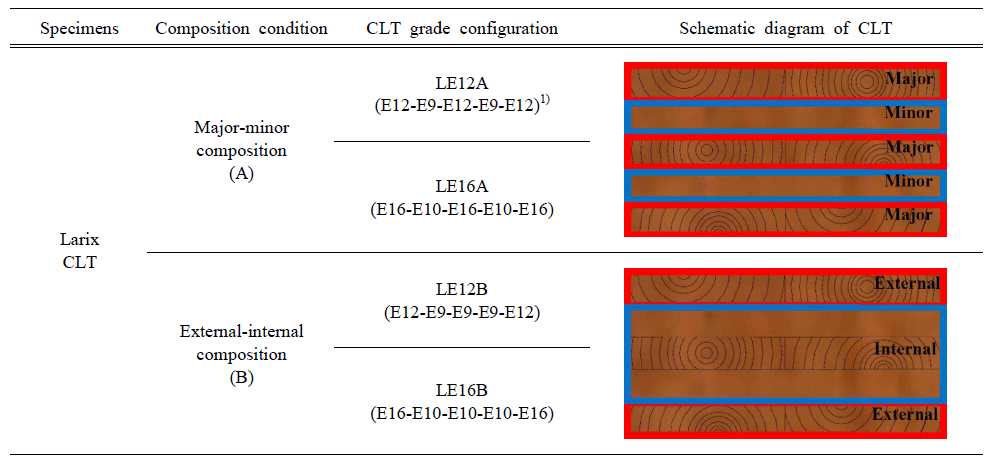
Product name | Viscosity (Pa∙s) | pH | Specific gravity | Odor | Solubility | Curing temperature (℃) | Color |
---|---|---|---|---|---|---|---|
Phenol-resorcinol-formaldehyde | 0.50 | 8.4 | 1.14 | Pungent odor | Easily soluble in water | 0–35 | Red brown |
The bending strength performance of Larix CLT was compared according to the lamina grade and composition. The surface of a bending specimen was 280 mm in width (w) and 150 mm in height (h). The specimen length was 3,300 mm (22 h). The bending strength test on the CLT was performed in reference to the methods of KS F 2081 (Cross-laminated Timber; KSA, 2021). A total of 16 specimens (four per composition) were prepared for the bending strength test. The span length was 21 h and the load length was 7 h. In Nocetti et al. (2013), two methods were applied to measure the MOE for CLT. They used the total bending deflection in the range of total length to calculate the global MOE (MOEG) which indicates the deformation caused by bending and shear stress, and used the bending deflection of the load length to calculate the local MOE (MOEL) which indicates the deformation caused purely by bending stress without shear stress. In this study, as shown in Fig. 2, the MOEG and MOEL were obtained by measuring the rate of deformation across span lengths and the rate of deformation across the load lengths with a yoke attached to the load length (6 h) on the anterior side of the CLT specimen and the span length (21 h) on the posterior side, in reference to EN16351 (EN, 2014). The resulting MOEG and MOEL were compared. A 4-point loading test was performed on the specimens at 12 mm/min load velocity using a universal compression tester (MTS cn/e45, maximum capacity 300 kN). Additionally, the Modulus of Rupture (MOR) was estimated using Equation (1), while the MOEG and MOEL were estimated using Equation (2) and Equation (3).
MOR is the modulus of rupture (MPa) and MOEG and MOEL are the global and local modulus of elasticity (GPa). Pmax is the maximum load (kN), a is the difference between the load length and the span length, b is the CLT width, and h is the CLT thickness. l is the length of the specimen; i.e., span length, and l1 is the gauge length for the determination of MOE. ∆Global and ∆Local each indicate the proportional limit of the global and local sections.
The compression specimen was 280 mm in width and 150 mm in thickness. To prevent the rupture by buckling in the horizontal direction, the specimens were prepared using a column of the slenderness ratio ≤ 30 which prevents buckling (ASTM D198, 2015). The specimen length was 270 mm for the slenderness ratio = 6.9. A total of 48 specimens (12 per composition) were prepared, and the compression strength test was performed at 2 mm/ min velocity using a compression tester. In reference to EN408, the compression strength and MOE were estimated using Equation (4) and Equation (5). The compression MOE was estimated based on the total deformation of specimens.
A is the cross-sectional area (mm2) and lc is gauge length for the determination of modulus of elasticity, ∆P is the load difference (N) of the linear domain on the load deflection curve, which is (P2–P1) on the graph in Fig. 3. ∆ω is (ω2–ω1) on the graph to indicate the difference in deflection (mm) that corresponds to (P2–P1).
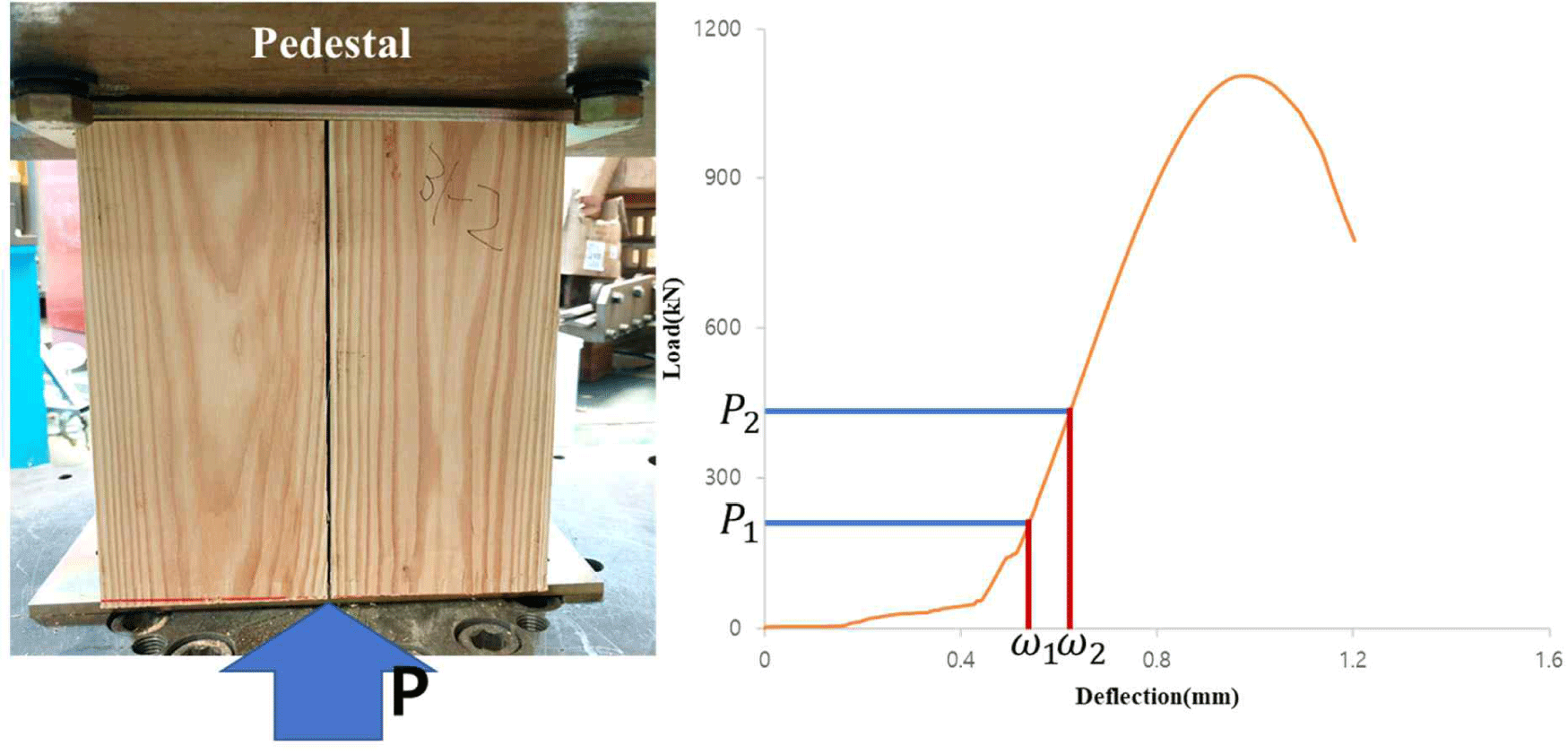
The bending characteristics were comparatively analyzed for CLT using those of laminae based on the prediction equations suggested in the CLT Handbook. The bending strength was predicted using the bending moment, and the MOE was predicted using the bending strength. To predict the MOE, two methods were tested: Shear-analogy Method by Equation (8) as a method incorporating the shear deformation in major and minor directions; and Gamma Method by Equation (9) as a method that does not incorporate the shear deformation in major direction but incorporates only the shear deformation in minor direction by applying the concept of effective strength. The Gamma method uses Equation (10), which is a Connection Efficiency Factor, to describe the shear deformation in minor direction. The MOE was estimated by dividing the bending strength by the secondary moment.
(fbS)eff is the effective bending moment for CLT (N․mm) and Fb,CLT is the allowable bending stress (MPa) for CLT. (EI)eff,S and (EI)eff,G each indicate the effective bending strength (N․mm2) for CLT in Shear Analogy and Gamma methods. ECLT is the MOE (MPa) for CLT and Ei is the MOE (MPa) of the ith lamina. by is the width of the lamina in the major direction, Ai is the area of the ith lamina, n is the number of CLT laminae, hi is the thickness of the ith lamina (mm), and Iyi is the cross-sectional secondary moment (mm4). Ai is the CLT surface area (mm2), zi is the length between the ith lamina and the central axis of the CLT (mm) and GR is the rolling shear modulus.
3. RESULTS and DISCUSSION
In a strength performance test, the bending performance was compared across Larix CLTs consisting of the major-minor composition (A type) and the external-internal composition (B type). Table 3 shows that LE12A had 15% higher bending strength than LE12B, whereas the MOEG and MOEL tended to decrease. LE16A had 7% lower bending strength than LE16B, and the MOEG and MOEL decreased by 6% and 5%, respectively. As can be seen in the failure mode, the bending strength of LE16 specimens was measured based on the rolling shear failure prior to rupture in bending at the tensile zone in the case of using high-grade laminae. In terms of the composition type, the B type exhibited a higher MOE than the A type. The MOE according to lamina grade indicated higher strength performance for CLTs using high-grade laminae. Furthermore, in agreement with Ravenshorst and van de Kuilen (2009) and Nocetti et al. (2013), the mean MOEL was generally higher than the mean MOEG.
Fig. 4 shows the failure modes after the 4-point bending strength test on Larix CLTs. Two failure modes; tensile failure mode and shear failure mode, were detected. The failure modes varied according to lamina grade and not to lamina composition for CLTs. The first failure mode is the mode with shear failure observed after rupture in bending, which mostly occurred in LE12A and LE12B comprising E12. As shown in Fig. 4(a), the most frequent rupture in bending involved the presence of a knot or tensile failure caused by bending stress. The knot in bottom layer, rather than that in top layer, generally appeared to be the direct cause of failure as the external lamina is under the strongest influence of bending stress. For LE12A, tensile failure was found on the external lamina, which did not transfer to the lamina in major direction in the middle layer, where shear failure occurred along the adhesive layer. It was also apparent that, following the maximum load measurement, ≥ 50% residual strength was maintained before failure ultimately occurred. For this, failure tended to be suppressed in the middle layer after the failure on the lamina at the tensile zone in the case of using lamina in major direction (Fig. 5). The second failure mode, as shown in Fig. 4(b), is shear failure observed mostly in E16 bending specimens using high-grade laminae. This is presumed to be due to the difference in lamina grade according to the arrangement, which led to rolling shear failure on the lamina in minor direction prior to rupture in bending. Pangh et al. (2019) and Ettelaei et al. (2022) reported that, in a bending strength test on CLTs using high-grade laminae ≥ E13 at 18 h span length, rolling shear failure was observed in most cases. In Pang and Jeong (2019), differences in the failure mode for CLT were observed according to the span to thickness ratio. Likewise, Hematabadi et al. (2021) reported that the maximum moment increased as the span to thickness ratio increased with reduced maximum shear force. This implies that, in the case of using laminae ≥ E16, the span to thickness ratio should be taken into account in determining the span length in a bending strength test for CLT.
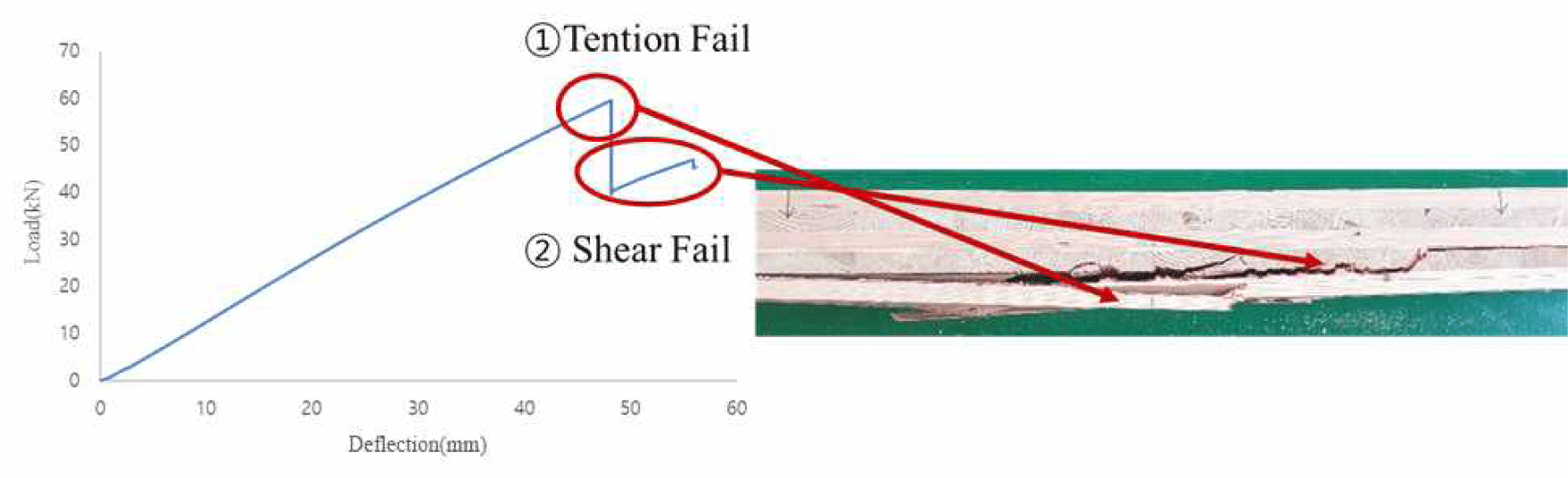
For Larix CLTs consisting of the A and B types, two prediction equations suggested in the CLT Handbook were applied in comparing the predicted and experimental values of MOE and the bending strength. For CLTs using laminae ≥ E12 grade, the ratio of the experimental value of bending strength as calculated by Equation (1) based on the predicted value of bending strength as calculated by Equation (7) (Experiment/Prediction) was 1.07–1.22. For CLTs using laminae ≥ E16 grade, the Experiment/Prediction ratio on bending strength was 0.90–0.98, which indicated a slightly lower level of strength than the predicted value.
The Experiment/Prediction ratio on MOE was 1.00–1.09 in the Shear-analogy method and 1.14–1.25 in the Gamma method. The comparison revealed that the values were closer to one another in the Shear-analogy method that simultaneously reflects the shear deformation in minor and major directions compared to the Gamma method that reflects solely the shear deformation in minor direction. Crovella et al. (2019) reported that the difference between predicted and experimental values was 5% in the case of softwood species. Baño et al. (2018) also reported that the accuracy was higher in the Shear-analogy method that reflects the rolling shear failure in minor direction and the vertical shear failure in major direction.
In a strength performance test, the compression performance was compared across Larix CLTs consisting of the A and B types according to grade and composition. The specimens were compared by type and mean values. For five plies, the compression strength performance increased by 2% and 9% in the A type CLT using E12 and E16, respectively, compared to the B type. The compression MOE showed an increase by 4% in both types (Table 4). The cross-sectional area with the load is critical in compression strength in the longitudinal direction for CLT. In the case of timber, approximately 3.4–4.4 times stronger compression load is supported in the longitudinal direction than in the transverse direction according to the section on long-term allowable stress for softwood machine graded lumber in Appendix A of the KS F 3020 (Softwood Structural lumber; KSA, 2018). As cross-lamination involves the longitudinal direction in the production method for CLT, differences arise in the rate of lamina in major direction. Hence, the compression strength performance of CLT is conjectured to be under a greater influence by lamina composition than lamina grade.
For compression strength, the failure modes were distinguished between those on the surface and those on the side of the external layer. Table 4 shows the surface failure mode ratio and the side failure mode ratio for each type of specimen. The New Wood Physics and Mechanics (Kang et al., 2008) was referred to regarding the surface failure modes on the external layer. As shown in Fig. 6, complex failure modes comprising two or more modes were frequently detected. The varying modes were generally compression failure, including Shearing (31.3%), Splitting (6.3%), Crushing (31.3%), Wedge splitting (25.0%) and Crushing and Splitting (6.3%). Even in an identical specimen, the onset of failure due to splitting on an external layer or a knot led to different failure modes on each anterior and posterior lamina. Thus, the failure mode on the part that displayed the first signs of failure was selected. The study by Tian et al. (2022) was referred to regarding the side failure modes, and the selected failure mode was the one on the part that displayed the first signs of failure. As shown in Fig. 7, the failure modes were divided into those on the external layer or layer in major direction, including Lumber Split, Knot Failure and Shear Failure, and those on the layer in minor direction or adhesion line, including Annual Ring Failure and Bond Line Failure. The failure was shown to be delayed in the A type for lamina composition and high-grade E16 for lamina grade to demonstrate a high percentage of Bond Line Failure or Annual Ring Failure compared to the failure modes on the external layer or layer in major direction. This is presumed to be due to the lamina in major direction in the middle layer suppressing the failure. Additionally, complex final modes were observed, such as Lumbar Split, Knot Failure and Shear Failure on the side of the external layer and Annual Ring Failure and Bond Line Failure on the internal layer.
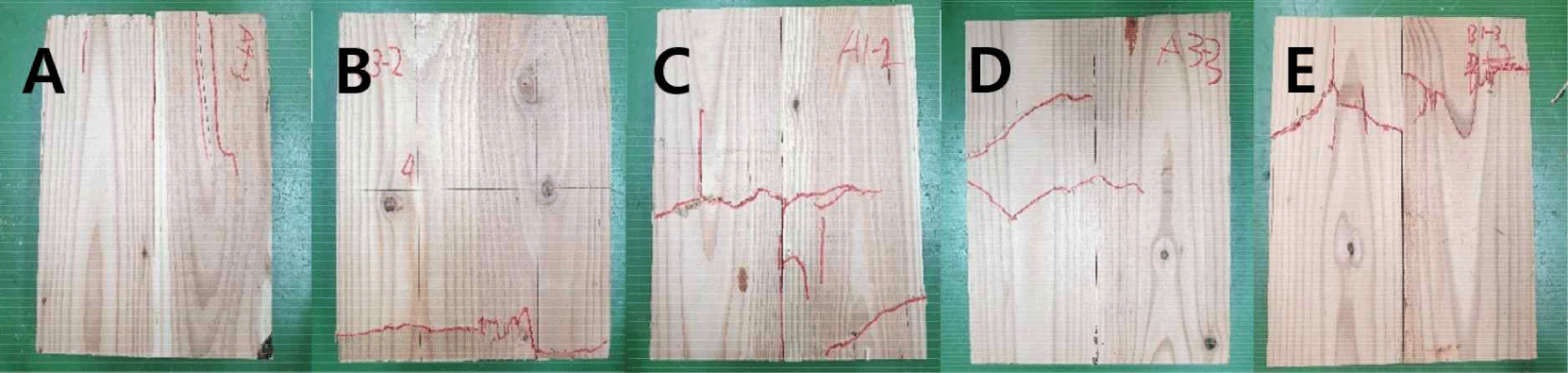
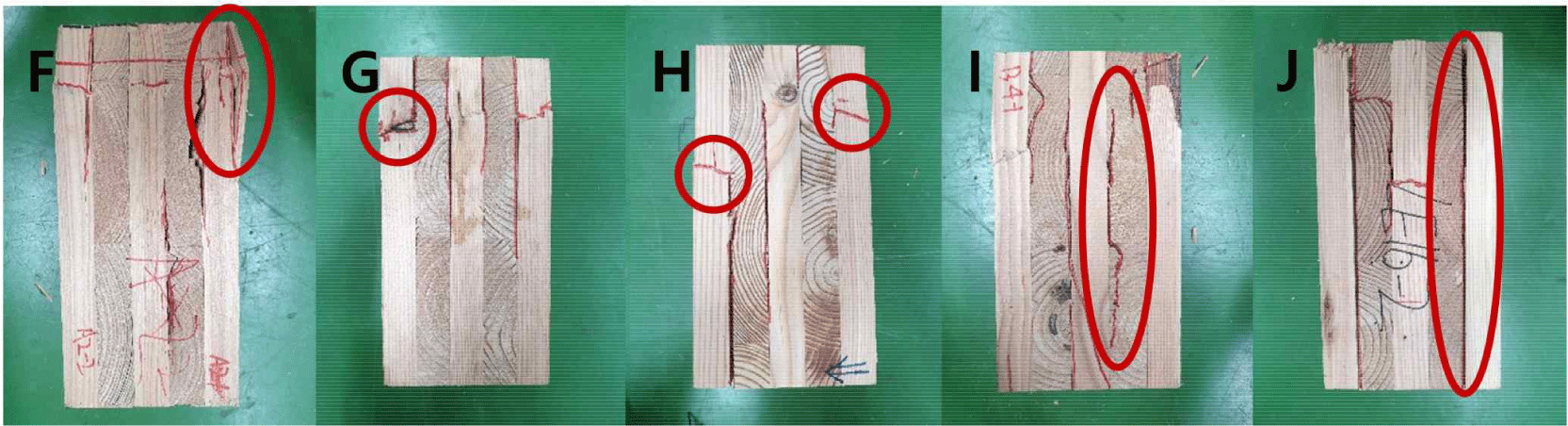
In Fig. 8, the area of the layer in major direction or external layer against the cross-sectional area is shown to decrease as the number of ply increases for CLT. In addition, the layer composition ratio for the A and B types remain identical up to three plies but the ratio varies as the number of ply increases. The percentage of low-grade lamina varies by 20% for five plies and by 29% and 33% for seven plies and nine plies, respectively. The margin of variation increases as the number of ply increases. Hence, the method of lamina composition for CLT should be reviewed to ensure efficient use of lamina.
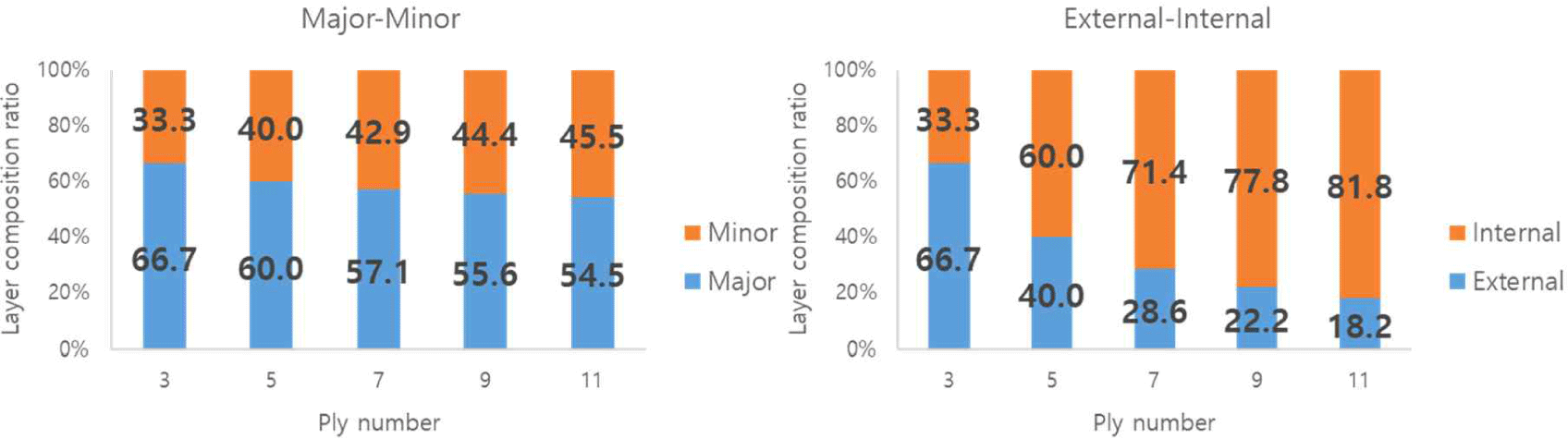
Considering the differences in strength as shown by previous test results as well as the layer composition ratio used in the A and B types, the lamina composition of the B type appears to be more outstanding with respect to the efficient use of lamina.
4. CONCLUSIONS
For Larix CLT produced in two different grades based on the lamina composition conditions that vary across countries between the major-minor and external-internal compositions, bending and compression strength tests for Larix CLT were performed in this study to identify not only the bending and compression strength performance but also the relation between the predicted and experimental values of bending strength. Furthermore, the failure modes for CLT after the tests were analyzed. The following conclusions were drawn.
-
The result of the bending performance test for CLT suggested that the MOE was under a greater influence by lamina grade than lamina composition. However, the use of lamina in major direction in the middle layer was shown to delay the progression of failure following maximum loading.
-
The predicted and experimental values of bending performance suggested that predicting the MOE and bending strength is adequately possible. For MOE, the Shear-analogy method with results that more closely approximated to experimental values seems to be a more efficient method to estimate accurate design values compared to the Gamma method. The Shear-analogy method is also a better method to predict the MOE to ensure the use that suits the lamina grade.
-
The result of the compression performance test suggested that the CLT compression strength was affected by the differences in grade for the lamina in major direction in the middle layer, which is presumably due to the differences in compression strength caused by the higher load on the lamina in major direction than on the lamina in minor direction. The A type was thus shown to be a better method of composition in terms of compression performance.
-
The most frequently detected failure modes in the compression strength test on CLT were Shearing and Crushing for the surface failure mode and Shear Failure for the side failure mode. In arranging the middle layer with high-grade laminae, rolling shear failure or bond line failure was observed in the lamina in minor direction to indicate continued compression loading in the middle layer.