1. INTRODUCTION
Many studies have been conducted on shear walls in timber construction (Jung et al., 2020; Lee and Jang, 2023). However, studies on roof and floor systems are not as numerous as those on shear walls. In timber construction, the deflection and creep of the floor and roof systems as well as the shear wall are important. For joists used in floor and roof systems, 2″ × 6″ or larger structural lumber should be used. When using structural lumber as a joist, there are restrictions on long spans. Owing to these problems, engineered wood such as glulam or CLT is mainly used and studied instead of structural lumber over long spans (Choi et al., 2020; Fujimoto et al., 2021; Yang et al., 2021). Galih et al. (2020) studied the mechanical properties of tropical hybrid cross-laminated timber using a bamboo-laminated board as the core layer. Park et al. (2020a) evaluated the bending creep properties of cross-laminated wood panels composed of tropical hardwoods and domestic temperate wood. Park et al. (2020b) tested the bending creep of glulam and bolted glulam under varying relative humidity. Choi et al. (2021) evaluated the flexural performance of ply lam CLT according to the plywood bonding method. Hwang et al. (2022) monitored the moisture and dimensional behavior of a nail-laminated timber concrete slab exposed to an outdoor environment. In addition to glulam and CLT, truss is a bending member that can be used for long spans. A truss is a structure in which the members are connected and arranged such that they are primarily subjected to axial loading. A truss has the advantage that it can be used for a longer span because the structure distributes the applied force to its members well, and the load is transmitted only in their axial direction. Trusses manufactured using timber have more advantages than those made of other materials. The advantages of timber trusses include the following: 1) Timber trusses are usually more cost-effective than metal trusses; 2) Wood trusses offer excellent thermal properties, particularly compared to steel; 3) Timber trusses are more flexible and versatile than those manufactured using other materials. Owing to these advantages, numerous studies on trusses have been conducted worldwide. Šilih et al. (2005) designed plane timber trusses by considering joint flexibility. Komatsu et al. (2018) experimentally and numerically analyzed the nonlinear behavior of wooden parallel chord trusses composed of self-tapping screws. However, only a few studies have been conducted on timber trusses in South Korea. Cha (1992) studied metal and plywood gusset plate connections for light-frame wood truss tension joints. Kim et al. (2014) studied the strength and application of OSB gusset trusses in field assemblies.
A truss in which the chord is made of timber and web members are made of steel is easier to manufacture than a truss in which both the chord and web members consist of timber. Moreover, it has the advantages of being cheaper, lighter to lift, and faster to install. Trusses consisting of timber chords and steel-web members have also been developed in other countries. Isopescu and Gavriloaia (2015) determined the model damping ratio of a hybrid floor system composed of timber chord and metal web members. Lennon et al. (2010) conducted a natural fire test on three engineered timber floor systems, including a timber truss incorporating upper and lower solid-timber chord members, and a pressed steel-web member.
In this study, a parallel chord truss was manufactured using steel-web members of a domestic company, S Co., and foreign company, P Co., for roof and floor systems. The bending properties of the two parallel-chord trusses were evaluated and compared.
2. MATERIALS and METHODS
In this study, to manufacture truss specimens, northern SPF No. 2 2 × 4 inch lumbers were used for the upper and lower chords. For all truss specimens, the upper and lower chord members were made of one piece of lumber without a lengthwise connection.
Concerning the steel-web members, two different types of steel-web materials, produced domestically and abroad, were used to compare their performance. The shapes and dimensions of the steel-web members used in this study are shown in Fig. 1 and listed in Table 1. Fig. 1(a) depicts a steel-web member manufactured by S Co., a domestic company. Fig. 1(b) shows the steel- web manufactured by P Co., a foreign company.
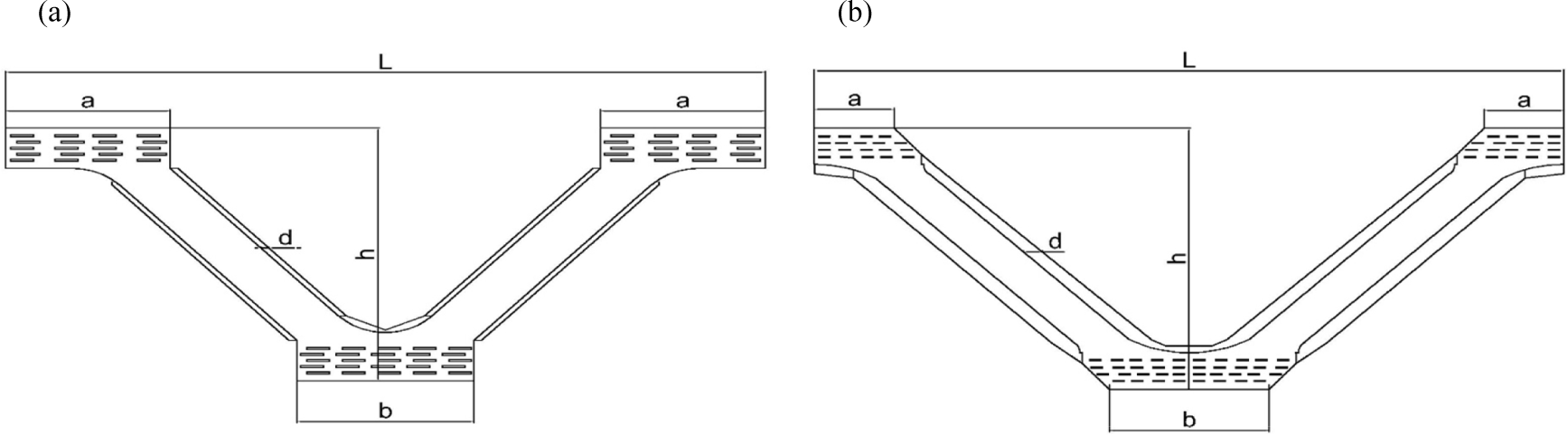
Steel web-member | Material | a (mm) | b (mm) | L (mm) | h (mm) | d (mm) |
---|---|---|---|---|---|---|
S Co. | Z275M280 | 100 | 135 | 600 |
200 250 300 |
7.34 |
P Co. | Z275M220 | 70 | 160 | 610 |
250 300 |
13 |
The parallel timber chord truss with steel-web (PCTSW) specimens used in this study were composed of timber chords and steel-web members, as depicted in Fig. 2, and are constructed as shown in Table 2. Note from this figure that the SAT specimen was manufactured by alternate installation of the steel-web members on both sides using those produced by the S company; SST and PST specimens were manufactured by continuous installation of the steel-web members on both sides using those produced by the S and P companies, respectively.
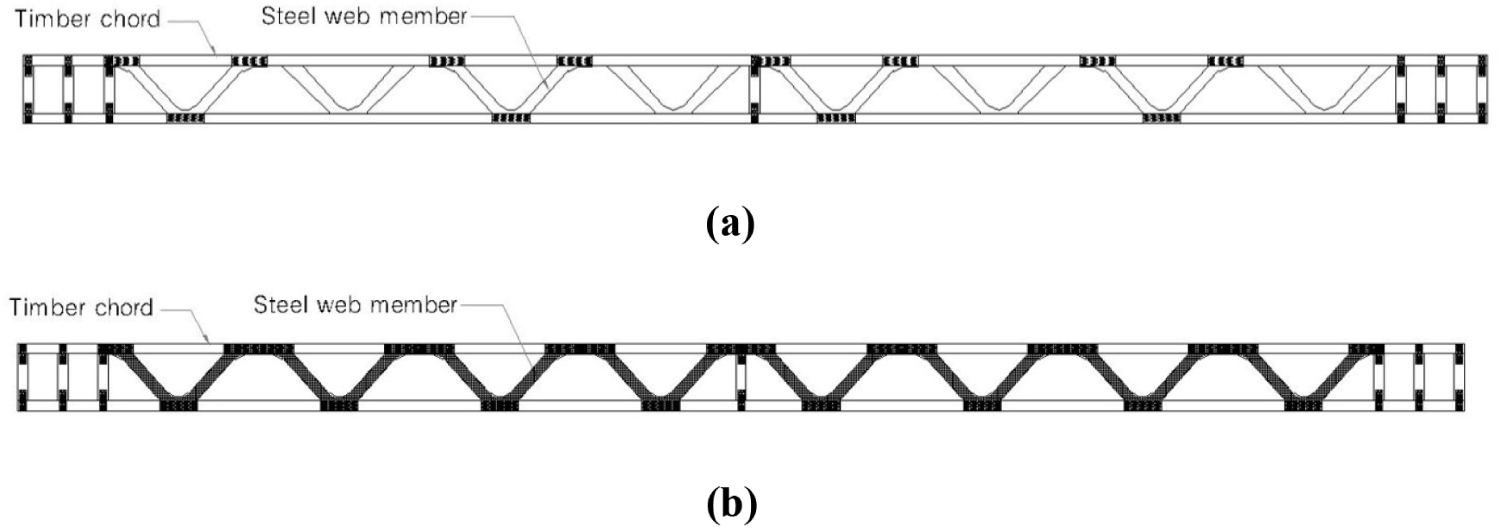
As listed in Table 2, the SAT specimens were manufactured using domestic S Co.; these specimens featured five different lengths and three different heights. The SST specimens were manufactured using domestic S Co.; they presented three different heights and lengths. The PST specimens were manufactured using imported P Co. and had two different heights and lengths.
The bending tests were performed in accordance with KS F 2150 to evaluate the bending properties of the truss specimens, as shown in Fig. 3 (Korea Standard Association, 2020). Note that the loading points were adjusted such that the load acted through the joints located at approximately 1/3 of the span. During the test, the deflection was measured using two linear variable differential transformers (LVDTs) attached to both sides at the middle of the specimen, and the average of two LVDTs measurements was recorded to determine the deflection of the specimen.
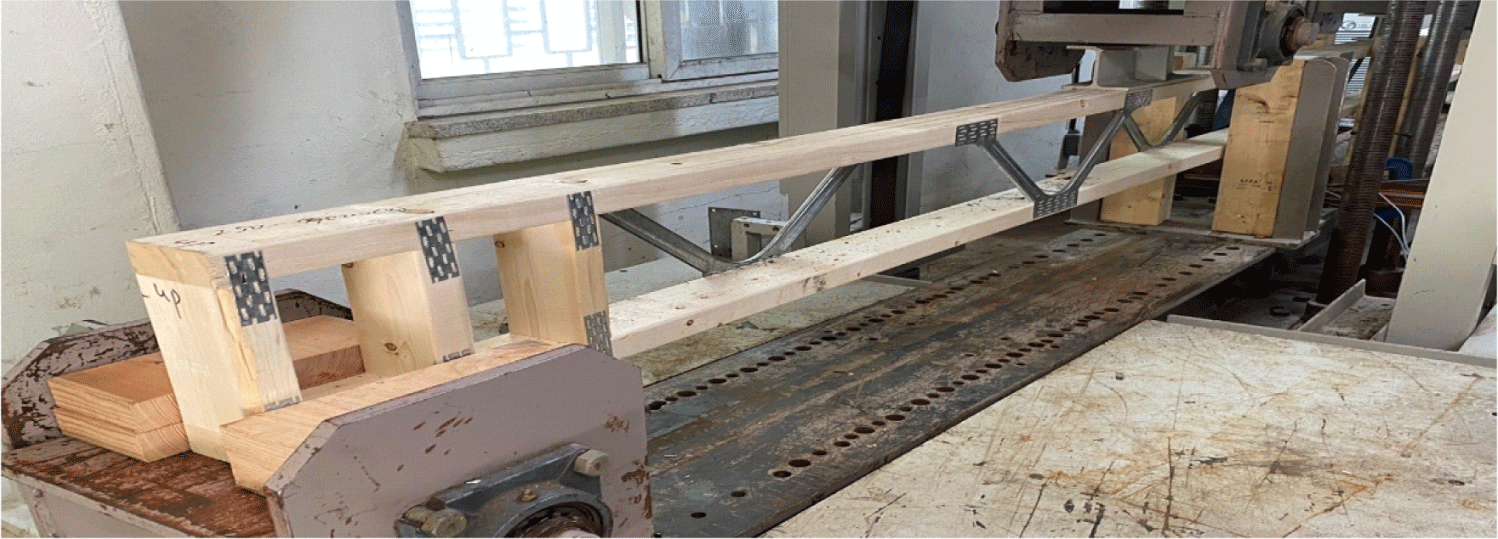
Load–deflection diagrams similar to the graph shown in Fig. 4 were obtained from the bending tests of the PCTSW specimens.
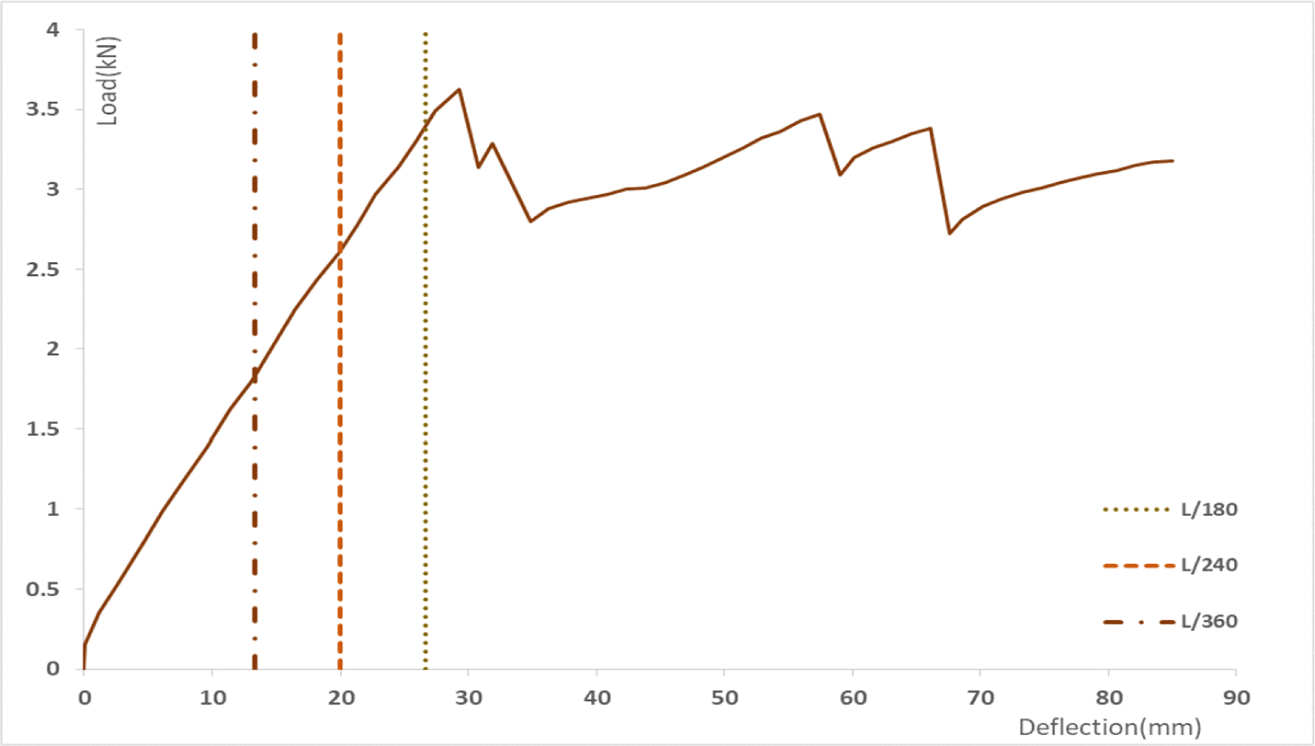
In Fig. 4, the dotted lines indicate the deflection limits of 1/360, 1/240, and 1/180 of the span, which can be applied to the design according to the loading conditions. The deflection limits obtained from KDS 41 50 20 (Ministry of Land, Infrastructure and Transport, 2022a) and KDS 41 50 70 (Ministry of Land, Infrastructure and Transport, 2022b), which depend on the deflection limit under loading conditions, are listed in Table 3.
Building code | Use classification | Live load | Total load (Live load + dead load) |
---|---|---|---|
KDS 41 50 70 | Roof beam | L/240 | L/180 |
Floor beam | L/360 | L/240 | |
KDS 41 50 20 | Roof and floor beam | L/360 | L/240 |
3. RESULTS and DISCUSSION
From the results of the bending tests for the PCTSW in this study, the loads at three deflection limits were obtained for each PCTSW specimen, as listed in Table 4.
Fig. 5 shows the failure modes in the bending tests of the PCTSW specimens. In all the test specimens, failure occurred because of the shear force rather than the bending moment. The steel-web member was bent by the shear force. Subsequently, the joint between the steel-web member and upper chord failed.
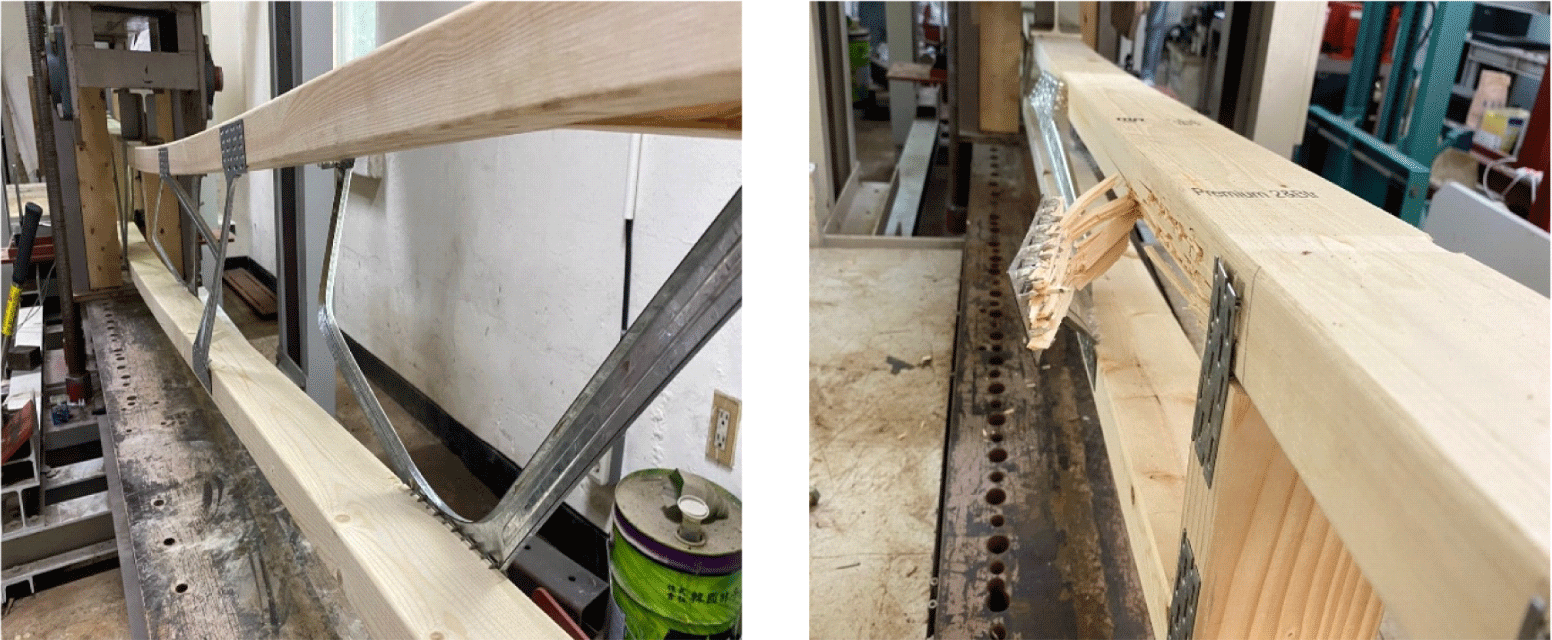
Fig. 6 depicts the change in load at three deflection limits for the SST 200 specimen featuring the same height but different lengths. This shows the effect of the length of PCTSW specimens. According to Fig. 6, the load at the deflection limit and the deflection limit itself increased from L/360 to L/180 regardless of the lengths of the specimen. Some trends were observed for all the specimens, as shown in Table 4.
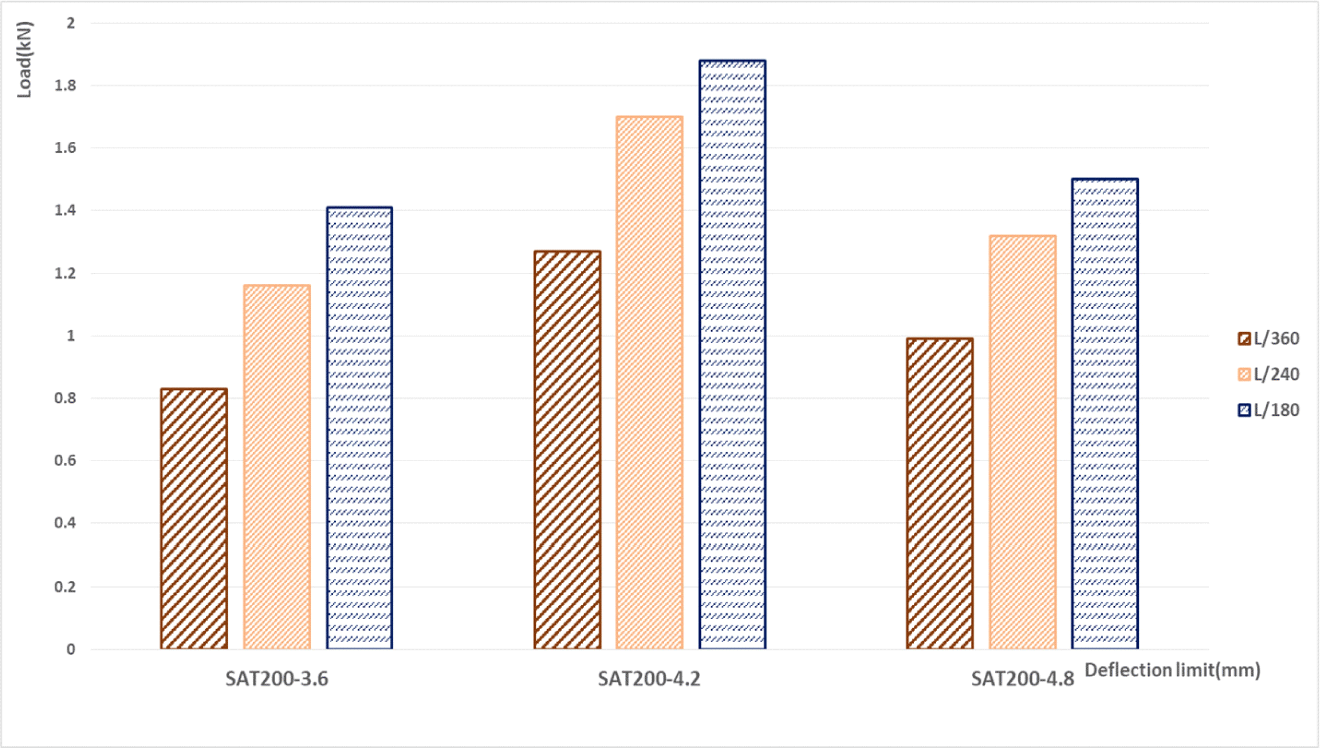
Fig. 7 shows the change in load at three deflection limits for SAT specimens. Load at the deflection limit increased as the height of PCTSW specimens increased from 200 to 300 mm.
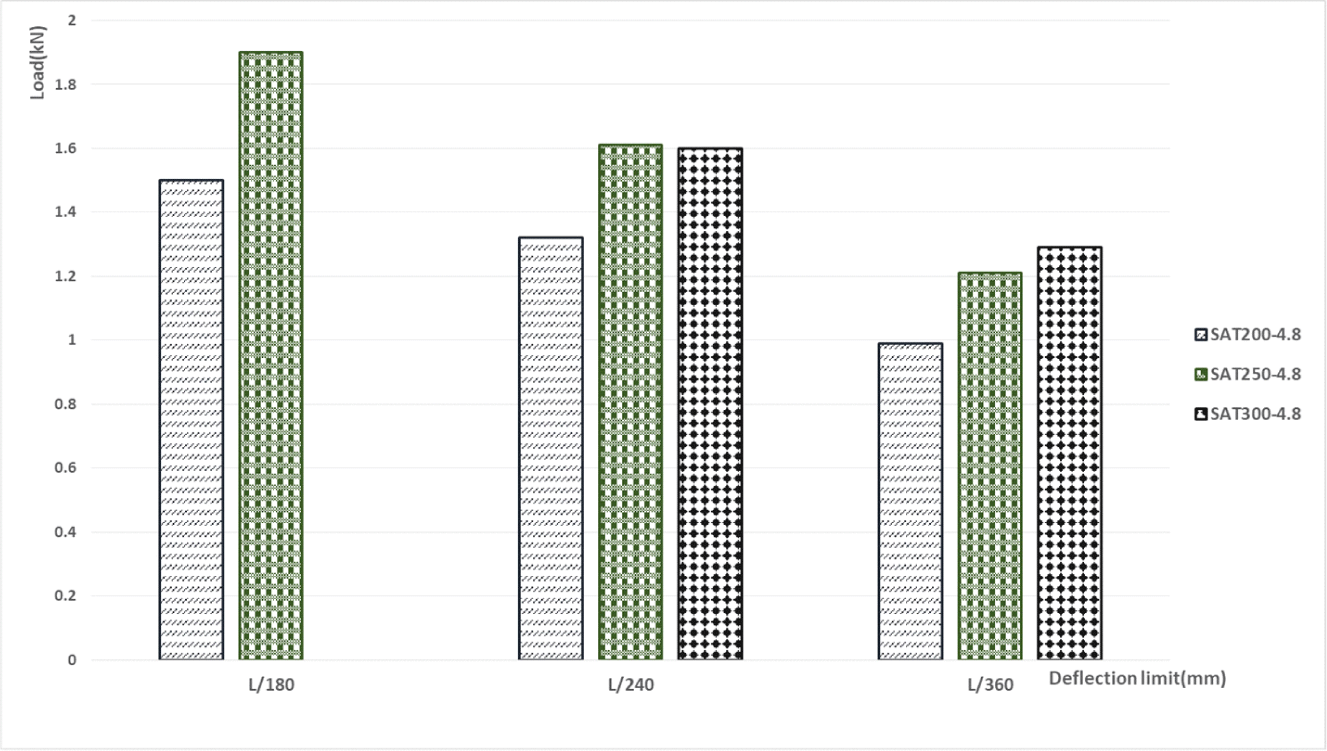
Fig. 8 shows the change in load at three deflection limits for SAT and SST specimens with the same height and length. For successive installation of the steel-web members (SST), approximately 1.4–1.7 times higher load was measured at each deflection limit than that of SAT specimens (alternate installation of the steel-web members).
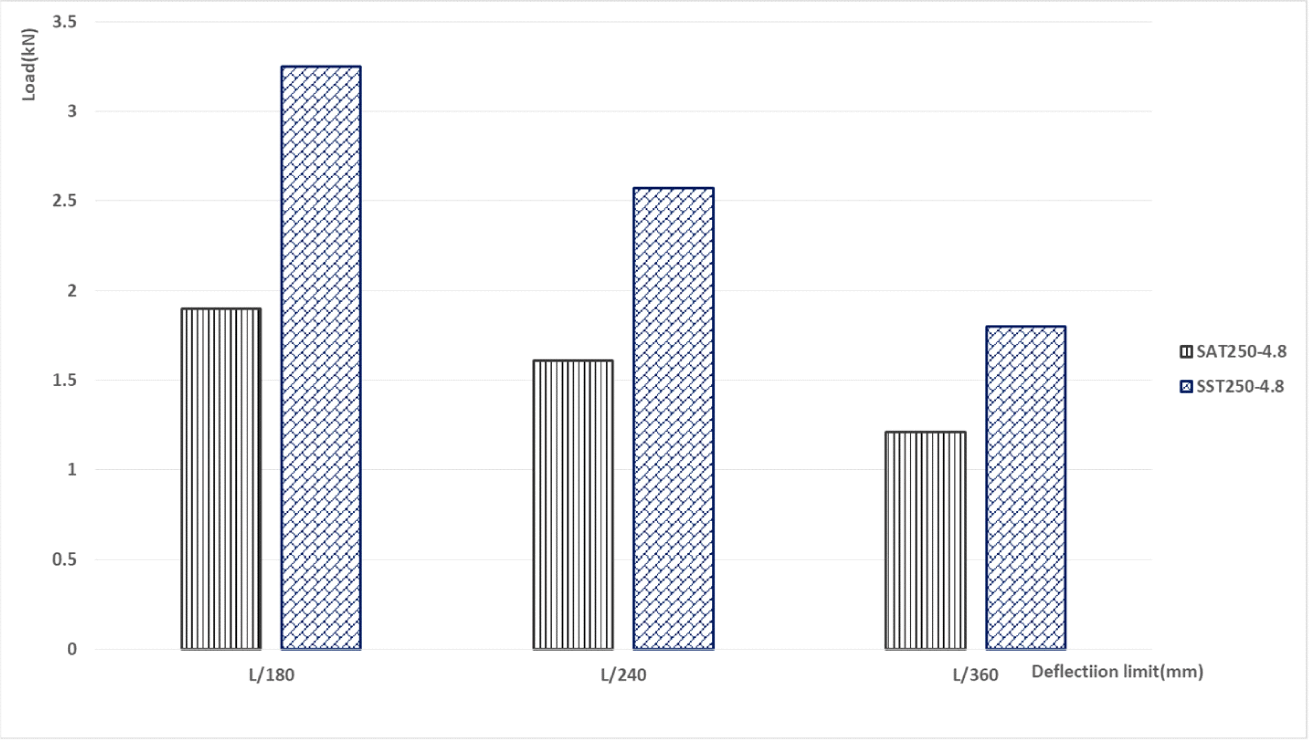
The changes in load at three deflection limits for different steel-web members is shown in Fig. 9. The load at each deflection limit for SST specimens (using steel-web members manufactured by S Co.). were higher than those of PST specimens (using steel-web members manufactured by P Co.). As described in Section 3.2., in all the test specimens, the steel-web members were bent by the shear force. Thus, this difference, which depends on the manufacturer of the steel-web member, is attributed to the material.
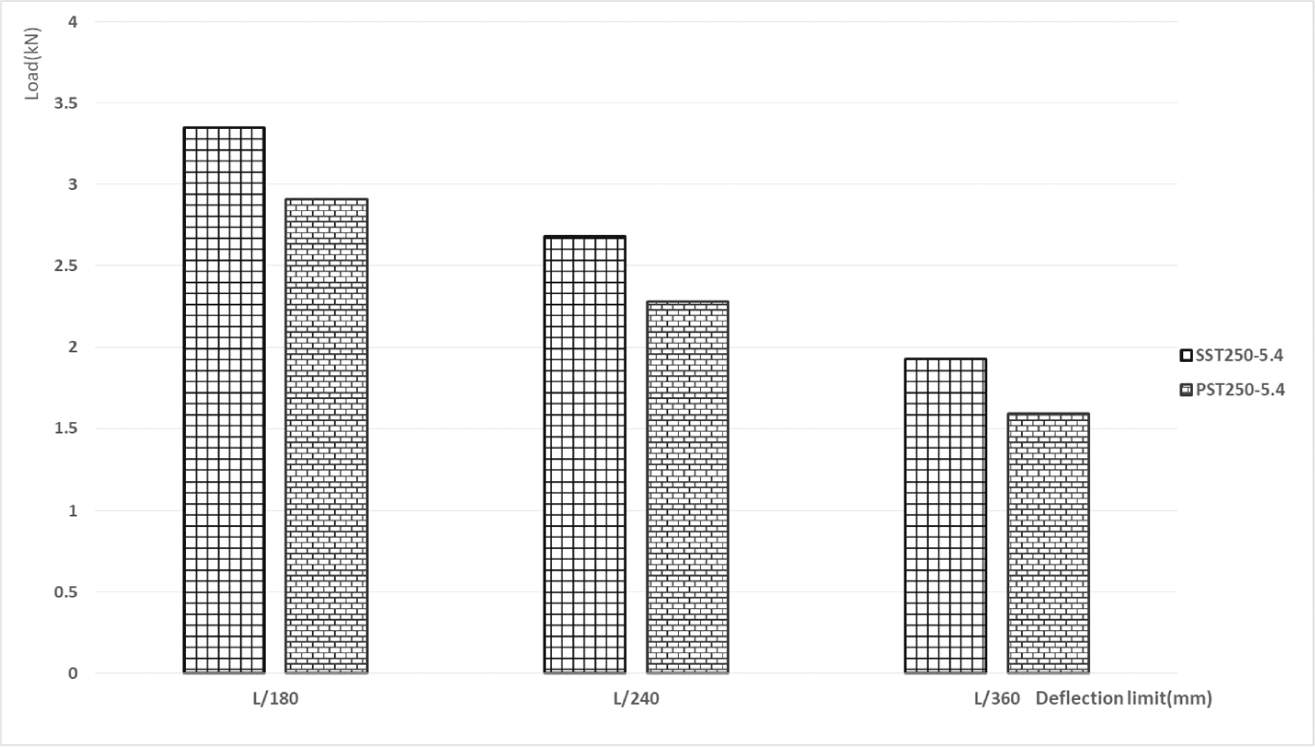
4. CONCLUSIONS
In this study, PCTSW specimens were constructed using timber chords and steel-web members. The bending strength of various types of PCTSW specimens according to their length and height, installation method of steel-web members, and type of steel-web member were tested according to KS F 2150. From the analysis of the test results, the following conclusions were drawn:
-
The load at deflection limits and the deflection limits themselves increased from L/180 to L/360 regardless of the lengths of specimens.
-
The load at deflection limits increased as the height of PCTSW specimens increased from 200 to 300 mm.
-
The successively installed steel-web members (SST) showed almost 2 times higher load at each deflection limit compared with that of SAT specimens (alternate installation of the steel-web members).
-
The load at each deflection limit for SST specimens (using steel-web members manufactured by S Co.) was higher than that of PST specimens (using steel-web members manufactured by P Co.).