1. INTRODUCTION
Some termite species are potential wood-destroying organisms, resulting in the economic loss of building materials. Subterranean termites are the most important wood pest in the world, with the Formosan subterranean termite causing extensive damage in Asian countries (Arinana et al., 2022; Kuswanto et al., 2015). Currently, the use of insecticides to prevent termite activity on wood has been restricted due to their negative impact on human health and the environment (Lee et al., 2020).
Plant products have been used as insecticides, insect repellents, antifeedants, as well as growth and development regulators for insects (Adfa et al., 2020; Arsyad et al., 2020; Ella Nkogo et al., 2022; El-Wakeil, 2013; Hadi et al., 2020; Isman, 2020; Oramahi et al., 2022). Phytochemicals or crude plant extracts are used in a variety of ways, including suppression of calling behavior (Lamy et al., 2017), growth retardation (Anshul et al., 2014), feeding inhibition, toxicity, oviposition deterrence (Abdullah et al., 2015; Sinthusiri and Soonwera, 2014), and reduced fecundity to destroy insects (Schmutterer, 2019).
The most promising plant families include Annonaceae, Asteraceae, Labiatae, Meliaceae, Piperaceae, and Rutaceae (Jiménez-Durán et al., 2021). Due to the presence of triterpenoids known as limonoids, two families, Meliaceae and Rutacae, have gained much attention. Azadirachtin is the main component responsible for the antifeedant and growth-inhibitory effects against insect pests. It is a tetranortriterpenoid limonoid extracted from the Neem seed (Azadirachta indica) in the family Meliaceae (Lin et al., 2021). Insecticides produced from this seed have proven to be effective against hundreds of insect pests in the order Diptera, Hemiptera, Lepidoptera, and Isoptera (Adhikari et al., 2020; Grace and Yates, 1992). Insecticides derived from neem have also a negligible impact on natural insect enemies, their potential for use in integrated pest management programs is extensive (Senthil-Nathan, 2015).
The biological activity of extracts from the family Meliaceae in relation to Coptotermes gestroi (Wasmann) was investigated (de Fatima Inacio and Carvalho, 2012). The effect of dichloromethane and methanol extracts of its three species, such as A. indica A. Juss, Melia azedarach L., and Carapa guianenses Aubl., on C. gestroi survival was examined. The survival time of the insect was significantly reduced by A. indica dichloromethane extract with 70% superior mortality beginning on the seventh day until 17th days. A. indica methanolic extract produced similar results. Termite survival was also significantly reduced by M. azedarach dichloromethane extract (de Fatima Inacio and Carvalho, 2012).
Seed kernel neem oil (A. indica) inhibited feeding of Reticulitermes speratus (Kolbe) at a dose of 5.44% and reduced R. speratus survival to 4% after 30 days in a no-choice bioassay (Serit et al., 1992). Ahmed et al. (2020) observed that Acacia nilotica sapwood and heartwoods treated with neem oil showed resistance to termite attack of Odontotermes obesus. In addition to the Indian neem tree (A. indica), the genus Azadirachta also includes Azadirachta excelsa (synonym: A. integrifoliola) is believed to be native to Indonesia, Malaysia, Papua New Guinea, the Philippines, and Vietnam (Kaewnang-O et al., 2011). Furthermore, the leaves of A. excelsa have been used as a traditional medicine to control blood glucose levels (Nurdiana et al., 2014).
Coptotermes curvignathus workers responded specifically to soils and pine blocks treated with acetone, hexane, and methanol extracts of A. excelsa leaves, which reduced wood consumption and survival of C. curvignathus, and also inhibited the termites’ tunneling activities in the treated soils (Sajap and Aloysius, 2000). Under controlled laboratory conditions, the larvicidal activity of fixed oil and crude extract from A. excelsa seed kernel showed LC50 values of 403.6 and 518.7 ppm, respectively, against Aedes aegypti (Kaewnang-O et al., 2011). Despite being a different species, A. excelsa has the potential to be a bioinsecticide similar to A. indica.
To the best of our knowledge, no studies have been conducted to investigate the anti-termite activity of A. excelsa seed kernel. This study examines the anti-termite activity of A. excelsa seed kernel n-hexane and methanol extracts, as well as its anti-termite compounds against C. curvignathus, through their chemical components analyzing using gas chromatography with mass spectrometry (GC-MS) and liquid chromatography linked to tandem mass spectrometry (LC-MS/MS).
2. MATERIALS and METHODS
GC-MS data was recorded using GCMS-QP2010S (Shimadzu, Kyoto, Japan). Separation and profiling of methanol extract was conducted using Vanquish Tandem UHPLC-Q-Exactive plus Orbitrap-HRMS (Thermo Fisher Scientific, Waltham, MA, USA). 1H NMR (400 MHz) and 13C NMR (100 MHz) spectra were recorded with a JEOL ECX 400 spectrometer with tetramethylsilane as an internal standard.
A. excelsa seeds were collected from Taba Lagan village, Talang Empat District, Central Bengkulu Regency, Bengkulu Province, Indonesia. Plant samples were identified at Herbarium Bogoriense, Biology Research Center of Indonesian Institute of Sciences (LIPI), and specimens (MA0207) were stored at the University of Bengkulu’s organic chemistry laboratory.
Formic acid, water, and methanol pro analysis (LC-MS grade) were purchased from Merck, while PTFE filter 0.22 μm was obtained from Anpel Laboratory Technology (Shanghai, China). Column chromatography (CC) was performed on silica gel (Wakogel® 60 N, 38–100 μm), and thin-layer chromatography (TLC) plates pre coated with Silica gel 70 F254 (Fujifilm Wako Pure Chemical Corporation, Osaka, Japan). Whatman filter paper number 3 with 9 cm of a diameter, and paper disc 1.5 mm thick and 8 mm in diameter (Advantec, Tokyo, Japan) were used for the anti-termite test.
A total of 1 kg of fresh A. excelsa seed kernel was macerated for 4 days in two different solvents with different levels of polarity (n-hexane and methanol). The solvents were evaporated using a rotary evaporator at 45°C. The re-maceration process was repeated five times to obtain the maximum extract from both solvents. Furthermore, 63.46 g of concentrated yellow n-hexane and 47.18 g of blackish-red methanol extracts were obtained with yields of 6.346% and 4.718%, respectively (Adfa et al., 2015).
The chemical components of the n-hexane and methanol extracts of A. excelsa seeds were analyzed using a GCMS-QP2010S with a capillary column DB-5ms [part number: 122-5532, 30 m (length) × 0.25 mm (inner diameter) × 0.25 μm (film thickness), Agilent, Santa Clara, CA, USA]. The column oven temperature started from 70°C (hold 5 min), then programed at 5°C/min to 300°C, and held for 19 min. The injection temperature was 300°C, and the carrier gas was helium. At 70 eV, the mass spectrometer was set to EI mode. The mass spectra were obtained by scanning the mass range from 28 to 600 in ACQ mode. The interface and ionization source temperatures were 305°C and 250°C, respectively. The GC peak area was used to calculate the percentage of components. The compounds were identified by comparing their retention time (RT) and mass spectra from Wiley Registry 11th Edition / NIST 2017 Mass Spectral Library.
Ultrahigh-performance liquid chromatography coupled with quadrupole-Orbitrap high-resolution mass spectrometry (UHPLC-Q-Orbitrap HRMS) was also used to analyze the methanol extract. This analytical instrument has a high sensitivity and accuracy in determining metabolite molecular weight. An amount of 50 mg of extract was dissolved in 5 mL of methanol and sonicated for 30 min. The solution was filtered into the vial with a 0.2 μm PTFE filter and was ready for analysis on the Vanquish Tandem UHPLC-Q-Exactive Plus Orbitrap-HRMS with a Thermo Fisher Scientific Accucore C18 column (100 mm × 2.1 mm × 1.5 μm). The mobile phase used was 0.05% formic acid in water (A) and methanol (B), and the elution times were as follows: 0–1 min (15%–20% B), 1–26 min (20%–55% B), 26–28 min (55%–95% B), 28–30 min (95% B), and 30–30.1 min (95%–15% B). The injection volume was 5 μL and the flow rate was 0.2 mL/min. The MS ionization source used was ESI with positive and negative ionization modes in the m/z range of 100–1,500 with a Q-Orbitrap mass analyzer, and the collision energy deployed for ionization was 18, 35, and 53 eV, automatic gain control was set at 3 × 106, and the injection time was set to 100 ms. The spray voltage was 3.8 kV, the capillary temperature was 320°C, and the sheath gas and auxiliary gas flow rates were 15 and 3 mL/min, respectively. The scan type is full MS/dd MS2 and the data set is compete scan with a resolving power of 70,000 FWHM. Thermo X-Calibur 2.2 was used to analyze the chromatogram data, and Compound Discoverer version 2.2 (Thermo Fisher Scientific) with an in-house database, chemical literature, and spectral database was used to perform the putative identification.
The methanol extract (18 g) was partitioned with n-hexane, and ethyl acetate in that order. Furthermore, 4.74 g of the ethyl acetate fraction was subjected to silica gel CC with n-hexane−ethyl acetate, and ethyl acetate−methanol stepwise (100:0 to 0:100) to obtain 14 fractions (A1-A14), which were then combined using TLC analysis. Finally, a single compound (colorless needles crystals, 40 mg) were obtained from fraction A5 by re-crystallization with methanol.
C. curvignathus Holmgren workers and soldiers were collected from termite-infested trees near the University of Bengkulu (Bengkulu, Indonesia). The colony was kept in a container at 28°C ± 2 and 80% ± 5 of relative humidity, and was fed wet filter paper until being used for the test. According to the previous method, a no-choice test was used to evaluate the termiticidal and antifeedant activity of A. excelsa seed kernel extract and its isolate.
A. excelsa seed kernel extract doses of 0% (solvent as a control), 2%, 4%, and 8% [sample mass (mg) / filter paper mass (mg) × 100%] were prepared, while 1.67% 6-deacetylnimbin was applied (0.5 mg in 0.03 g paper disc). The samples were dissolved in 500 μL n-hexane/methanol/acetone for extract and 100 μL for 6-deacetylnimbin, and the resulting solution was applied to Whatman No. 3 filter papers for extracts and to paper discs (1.5 mm thick and 8 mm in diameter for 6-deacetylnimbin, which were then dried overnight at room temperature, followed by two hours in a vacuum desiccator. Four replications were performed for each dose, after which the dried filter paper was placed in a Petri dish (90 mm diameter × 20 mm height). Finally, twenty workers and two soldier termites from active C. curvignathus were tested.
The petri dishes were placed on a wet cloth in a plastic tray and kept in the darkroom at room temperature (28°C ± 2) and 80% ± 5 of relative humidity for 14 days. The termite’s mortality was determined, from which the termiticidal activity was evaluated. Furthermore, the antifeedant activity of the isolated compound (6-deacetylnimbin) was determined using the percentage weight loss of paper discs.
3. RESULTS and DISCUSSION
Figs. 1 and 2 show the GC-MS total ion current chromatogram of A. excelsa seed kernel extracts. The n-hexane extract contained 47 components, while its methanol counterpart contained 30. Given that the GC-MS technique for analyzing volatile compounds was properly performed, it is understandable that there were more compounds in the n-hexane extract than in the methanol extract. Tables 1 and 2 show that after comparing the similarity index MS spectrum of each component with mass spectral libraries (NIST 62 and, Willey 229 Libraries), 20 putative components were identified from the n-hexane extract, while 19 were from its methanol counterpart.
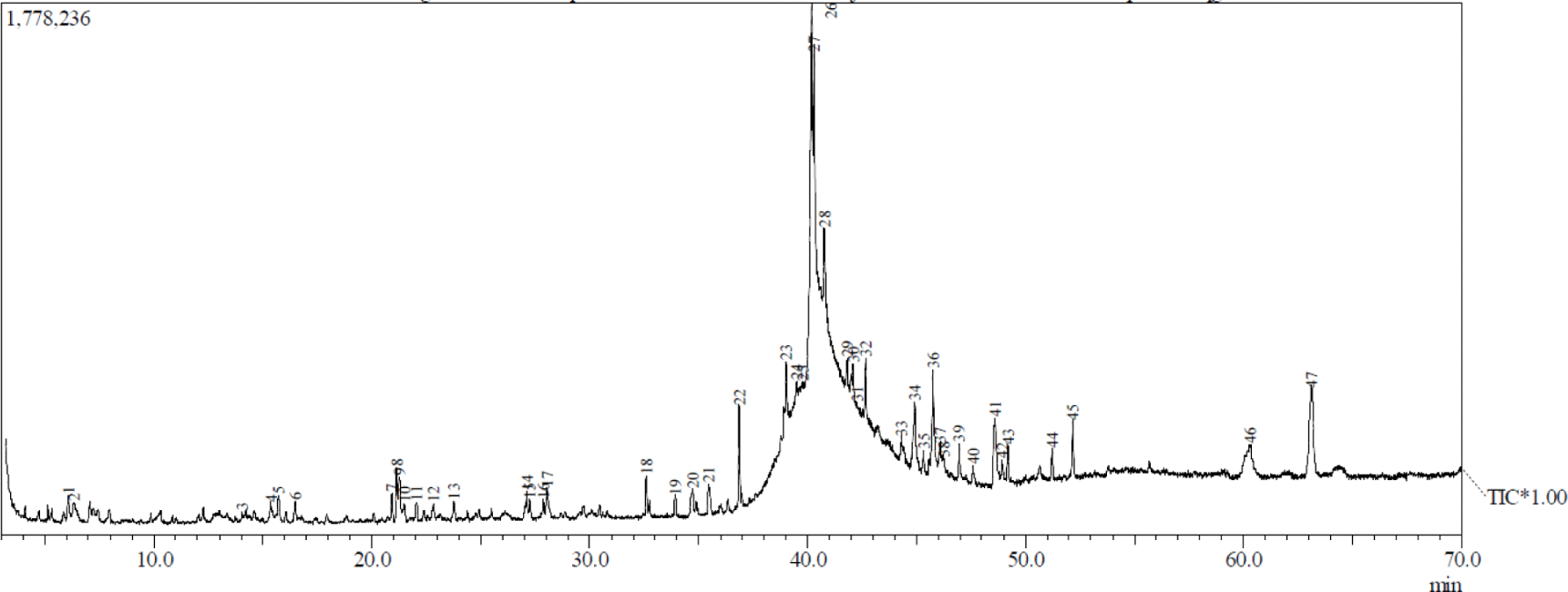
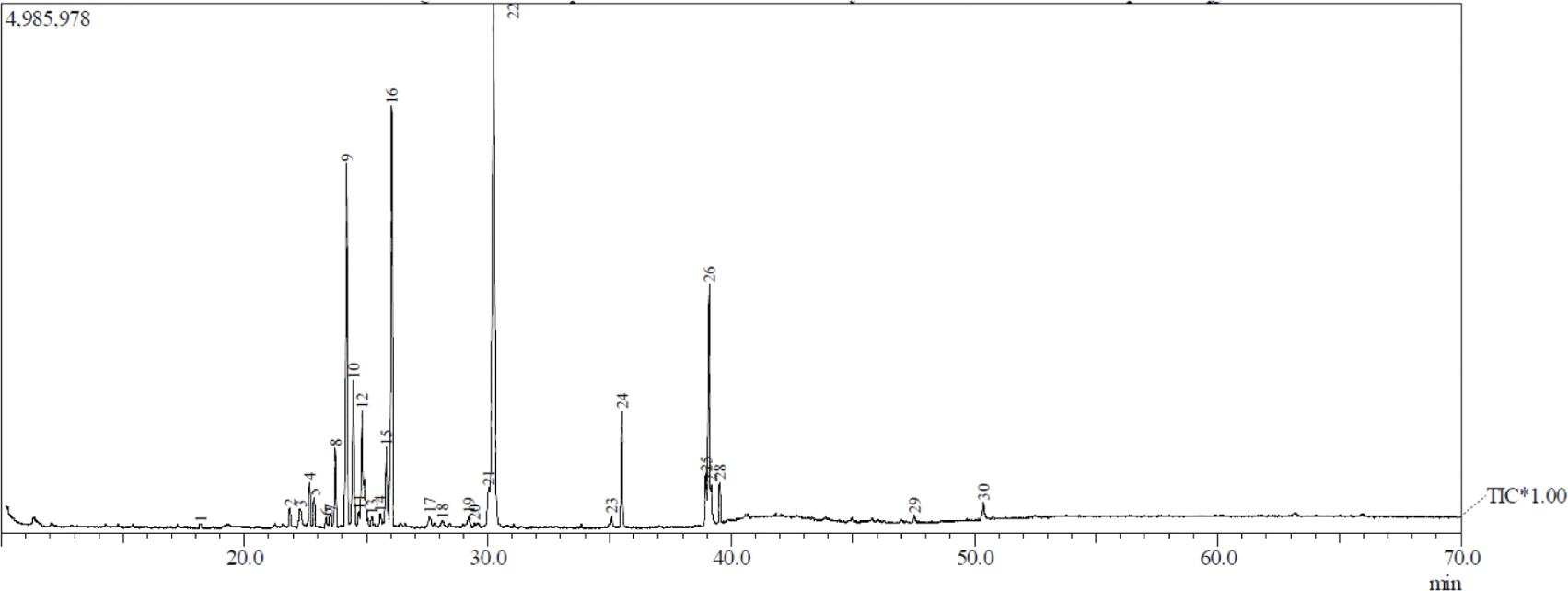
The highest peak region of n-hexane extract was obtained in peak 28 at 18.99% and was identified as hexadecanoic acid, ethyl ester from the fatty acid esters. The others above 16% were peak 26 at 16.31% and peak 27 at 16.23% and were identified as 9,12-octadecadienoic acid (Z,Z)- and 9-octadecenal, respectively. Patchouli alcohol was discovered as the highest peak region of methanol extract obtained in peak 22 at 28.1%. The others above 6% were identified as delta-guaiene, alpha-guaiene, 11-octadecenoic acid, methyl ester, and alpha-patchoulene, and were peak 16 (15.15%), peak 9 (11.93%), peak 26 (7.84%), and peak 12 (6.09%), respectively. The majority of the compounds detected by GC-MS in both extracts were fatty acids, fatty acid esters, sesquiterpenes, monoterpenes, aldehyde, long-chain hydrocarbons, and alcohols.
There is currently limited information on A. excelsa seed constituents. According to Kurose and Yatagai (2005), the primary components of the essential oil in the seeds growing in the Philippines were oleic acid (31.3%), hexadecanoic acid (14.2%), octadecanoic acid (13.0%), 4-octylphenol (9.7%), and O-methyloximedecanal (6.8%). In comparison, Shafie et al. (2015) reported that GC-MS data of a 70% ethanol extract of A. excelsa leaves collected in Selangor, Malaysia had fatty acids being the primary chemical components detected. Its major compounds were 9,12,15-octadecatrienoic acid (42.34%), pentadecanoic acid, 14-methyl-, methyl ester (28.99%), phytol (10.63%), 9,12,15-octadecatrien-1-ol (5.37%), octadecanoic acid, methyl ester (4.36%), 9,12-octadecadienoic acid, methyl ester (4.24%), and hexadecanoic acid, ethyl ester (4.06%).
The positive mode ionization chromatogram in Fig. 3 shows the metabolite profile of A. excelsa seed kernel methanol extract using UHPLC-Q-Orbitrap HRMS. A total of 15 limonoid compounds were putatively identified based on confirmation of the ion precursor values and MS2 fragmentation patterns with the available literature, as shown in Table 3. At a RT of 17.622 min, the main chemical components were detected. The primary constituents of the A. excelsa seed kernel methanol extract were putatively identified as 6-deacetylnimbin after comparing the MS data with comparative information and supporting literature. Also, 14 other limonoid compounds were identified.
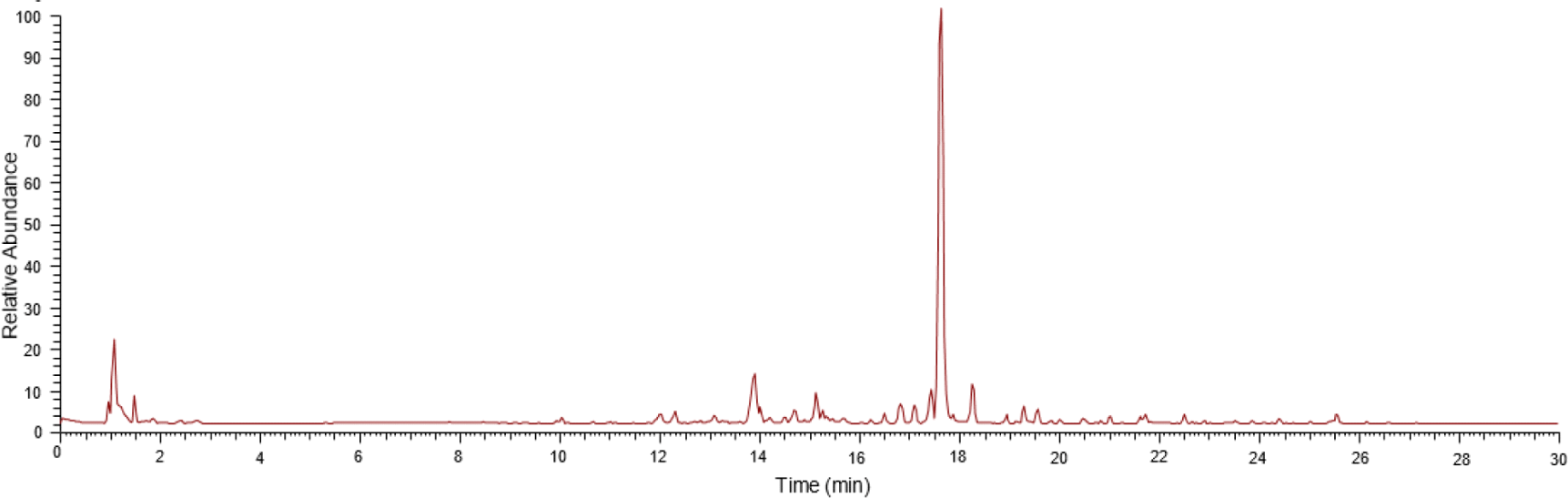
Limonoids are bioactive molecules that have undergone complex functionalization in the skeletal structure of steroid skeleton consisting of A, B, C, and D rings. The C ring is cleaved and opened to create a group known as C-seco limonoids. In contrast, those without this modification are known as ring-intact (basic) limonoids, and D-seco limonoid rings are another type (Lin et al., 2022; Luo et al., 2022).
A. excelsa seed kernel contains three ring-intact limonoids, including epoxyazadiradione (compound 10, RT = 25.546), azadirone (compound 11, RT = 22.498), and nimbocinol (compound 14, RT = 18.264) which has an azadirone skeleton. Additionally, one D-seco limonoid gedunin (compound 8, RT = 19.554) was identified. The mass product ions at m/z 435 and 407 of compound 10 was generated by the neutral loss of [M + H – MeOH]+ and [M + H – AcOH]+. Cleavage of the B-ring of the product ion at m/z 407 from compound 10 generates a product ion at m/z 271 (C17H19O3)+ and a low mass at m/z 121 (C9H13)+ (Mulani et al., 2021). The neutral loss of [M + H – AcOH]+ yields the highest mass production of compound 8 at m/z 423.
Furthermore, the fragmentation of compounds 8, 10, 11, and 14 resulted in the formation of product ions at m/z 109, 135, and 173. According to Mulani et al. (2021) identified the basic limonoid framework on the ion production at m/z 109 (C6H5O2)+ and m/z 135 (C9H11O)+ containing intact furan rings generated by protonation initiated in the D-ring. The ion at m/z 109 is produced by protonation beginning with the A-ring and including the cleaved portions of the A- and B-rings. Furthermore, protonation and subsequent elimination of the C7 acetoxyl group and fragment containing an intact A-ring, initiated the formation of the product ion at m/z 135. The protonation initiated at the D-ring after cleavage at the B-ring produced the product ions at m/z 173 (C12H13O)+.
Ten other C-seco limonoids are identified in the methanol extract of A. excelsa seed kernel. Ten compounds detected were nimbolidin C (compound 2), nimbolide (compound 3), 6-acetylnimbandiol (compound 4), 6-deacetylnimbinene (compound 5), salannol (compound 6), 28-deoxonimbolide (compound 7), nimbandiol (compound 9), 2’,3’-dihydrosalannin (compound 12), marrangin (compound 13), and azadirachtin (compound 15) in Table 3. The key fragments of C-seco limonoids were observed to have m/z greater than 300, resulting from the loss of functional groups as neutral molecules such as water, methanol, carbon monoxide, acetic acid, formaldehyde, tiglic acid, and isovaleric acid. Lower-mass fragments were formed as a result of C-C bond cleavages and rearrangements that resulted in skeletal fragmentation (Aarthy, 2019).
The key low-mass fragment of compounds 3, 4, 5, 6, 7, 9, and 12 were observed at m/z 147 (C10H11O)+ with highest intensity, corresponding to the presence of typical C and D-ring substituted with 17-furan moiety. Two other fragments with high abundance were discovered at m/z 171 (C13H15)+, and 187 (C13H15O)+. At m/z 171, it was most likely a decalin ring containing a conjugated polyene system and lacking oxygenated functional groups. Meanwhile, the fragment at m/z 187 was likely formed by cleaving the C-ring, C-9, and C-6 substituents from the decalin ring system with an intact A-ring.
In addition, nimbolide is detected at 17.621 min with the ion precursor m/z 467 [M + H]+ and other MS2 fragment patterns include m/z 449 [M + H – H2O]+, and 435 [M + H – MeOH]+. Similarly to nimbolide, 28-deoxonimbolide with ion precursor m/z 453 [M + H]+ was generated at m/z 421 [M + H – MeOH]+.
The fragment m/z 161 and 83 of azadirachtin (RT = 17.488) as well as marrangin (RT = 15.009) corresponds to the hydrofuran acetal moiety and tiglate group. Meanwhile, the mass product ions at m/z 605 of marrangin were produced by the neutral loss of [M + H – tiglic acid]+. Similar to azadirachtin and marrangin, the most intense at m/z 161 was produced by nimbolidin C, while the parent ion at m/z 687 [M + H]+ is the second abundant limonoid compound in the A. excelsa seed kernel. Among the 15 limonoids identified in A. excelsa seed kernel, 6-deacetylnimbinene, nimbandiol, and 6-acetylnimbandiol, constitute the class of penta-nortriterpenoids (Kraus and Cramer, 1981).
There has been limited information on the secondary metabolite content of A. excelsa seed. David Morgan and Wilson (1999) reported that it contains marrangin, azadirachtin, and 1-tigloyl-3-acetylazadirachtol. In addition, the 15 limonoids identified in this study have previously been reported in Neem (A. indica) oil and extracts.
The daily termite mortality of C. curvignathus treated with A. excelsa seed kernel n-hexane and methanol extracts was determined for 14 days using the no-choice feeding test. The results showed that both extracts reduced C. curvignathus survival at all concentrations tested compared to the corresponding control. At all test doses, the methanol extract exhibited greater anti-termite activity than its n-hexane counterpart. Termites can be eradicated entirely by the methanol extract at a dose of 2% after six days. At 8% concentration, 98.86% and 100% mortality are recorded on the second and third day, respectively. However, at 4%, the termites were completely killed on the fifth day. An n-hexane extract of A. excelsa seed kernel at doses of 2%, 4%, and 8% killed 37.50%, 79.55%, and 97.73% of the termites on the 14th day, respectively. Based on Fig. 4, it can be concluded that the methanol extract contains compounds that are more effective at killing the test termites than the n-hexane extract. Previous reports showed that the n-hexane and methanol extracts of A. excelsa seeds had larvicidal activity against A. aegypti, and the methanol extract of A. excelsa wood had the potential as an antifeedant against the Crocidolomia binotalis Zeller (Kaewnang-O et al., 2011; Teik et al., 2003).
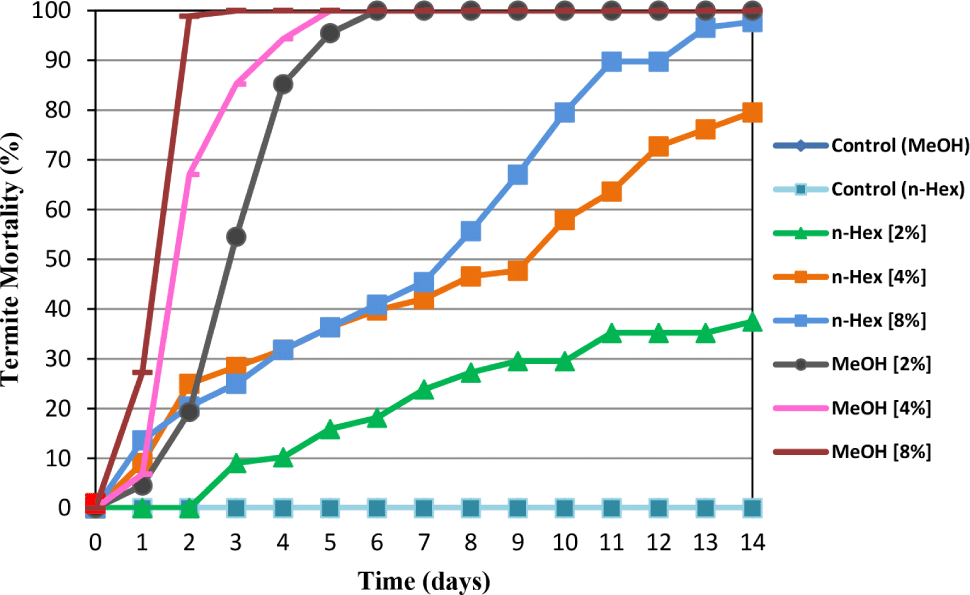
The chemical components of n-hexane and methanol extracts was detected by GC-MS and LC-MS/MS. For n-hexane extract, it was dominated by fatty acids, while the methanol extract detected several compounds thought to reduce termite survival. The largest peak area of A. excelsa TIC GC-MS methanol extract chromatogram detected patchouli alcohol, while LC-MS/MS suggested the presence of 6-deacetylnimbin. Previous research discovered that patchouli alcohol killed all Formosan subterranean termites tested (Coptotermes formosanus) within 72 hours at a dose of 50 mg per gram of sand. It was observed to be toxic, repellent, and have a neurotoxic mode of action toward termites (Zhu et al., 2003). Adfa et al. (2013) also reported that 6-deacetylnimbin inhibited the feeding of C. formosanus Shiraki. In addition to 6-deacetylnimbin, 14 putative limonoid compounds were discovered in the methanol extract of A. excelsa seed kernel (Table 3) and might be associated with anti-termite activity. According to previous study, nimbolidin C was isolated from the root bark of Melia toosendan and has property as an insect feeding inhibitor against Japanese pest insect Spodoptera eridania (Nakatani et al., 1996). Also, nimbolide isolated from A. indica leaves showed insecticidal efficacy against S. frugiperda (Navinraj et al., 2021). Azadirachtin, derived from A.indica and A. excelsa, was the most widely used botanical insecticide. It was observed to have potent insecticidal properties against over 400 insect species. Azadirachtin has antifeeding, growth inhibition, repellent, stomach poisoning, and sterilizing properties (Lin et al., 2021). Azadirone was isolated from an acetone extract of Trichilia havanensis seeds and demonstrated significant antifeedant activity against Leptinotarsa decemlineata at 300 ppm (Rodríguez et al., 2003). In another report, gedunin, nimbandiol, and 6-deacetylnimbin, isolated from Neem oil, were discovered to have antifeedant activity against R. speratus Kolbe, with 95% protective concentrations of 218.4, 245.4, and 1,581.2 g/disc, respectively (Ishida et al., 1992).
The termiticidal activity of the n-hexane, and methanol extracts of A.excelsa seed kernel was investigated. The methanol extract showed higher termiticidal activity than its n-hexane counterpart, hence, it was selected for examination in the present study. Furthermore, it was partitioned with n-hexane, and ethyl acetate. Ethyl acetate fraction of 4.74 g was subjected to silica gel CC, and the re-crystallization with methanol resulted in the purification of a single compound (colorless needle crystals) from fraction A5. Spectroscopic data are used to determine the isolate’s molecular structure. Following its isolation, the compound was tested for anti-termite activity against C. curvignathus.
Isolated compound was obtained as colorless needle crystals. The 1H-NMR spectrum revealed significant signals due to the tertiary methyl groups at δH 1.22, 1.29, and 1.59 (all singlets). A doublet at δH 1.69 was assigned to a methyl group on the double bond, and two singlets at δH 3.66 and 3.70 was assigned to the carbomethoxy groups. Meanwhile, wide doublets at δH 5.85 and 6.41 were assigned to the enone system’s olefinic protons, and the characteristic signals at δH 6.33, 7.24, and 7.33 revealed the presence of a substituted furan ring. These structural moieties, as well as a ketone (δC 202.3) and two carboxylic groups (δC 173.8 and 175.6), were detected in the 13C NMR spectra. The presence of oxygenated methine carbon, bearing a hydroxy group, was suggested by the 13C NMR signal at δC 66.3. Isolated compound was identified as the limonoid 6-deacetylnimbin (Fig. 5) using spectral data from 1H and 13C-NMR, 2D-NMR, and comparison with the literature (Adfa et al., 2013; Anitha et al., 2007). This follows the LC-MS result, which shows that the most abundant non-volatile compound in the methanol extract of A. excelsa is 6-deacetylnimbin.
This is the first report indicating that A. excelsa seed contains limonoid 6-deacetylnimbin. Limonoids have also been isolated from its stem extracts, including 2,3-dihydronimbolide, 3-deoxymethylnimbidate, nimbolide, and 28-deoxonimbolide, using bioassay guidance (Cui et al., 1998). Limonoids are a class of chemicals related to bitter tetranortriterpenoids found primarily in the Meliaceae, Rutaceae, and Simaroubaceae families (Roy and Saraf, 2006). Compounds in this class have demonstrated a wide range of biological activities, including insecticide, insect antifeedant, antibacterial, antifungal, antimalarial, anticancer, and antiviral, as well as growth regulation, and a variety of other pharmacological activities (Endo et al., 2002; Koul et al., 2004; Nakagawa et al., 2001). Furthermore, β-sitosterol has been isolated from A. excelsa stems (Sanjaya et al., 2019).
1H NMR (400 MHz, CDCl3): δ 1.22 (3H, s, H-19), 1.29 (3H, s, H-30), 1.59 (3H, s, H-29), 1.69 (3H, d, J = 1.4 Hz, H-18), 1.99–2.08 (2H, m, H-16), 2.17–2.25 (1H, m, H-11b), 2.77 (1H, t, J = 4.1 Hz, H-9), 2.90 (1H, dd, J = 5.8 and 16.6 Hz, H-11a), 3.39 (1H, d, J = 11.7 Hz, H-5), 3.66 (3H, s, OMe), 3.68 (1H, s, H-17), 3.70 (3H, s, OMe), 3.92 (1H, ddd, J = 3.7, 11.2, and 11.2 Hz, H-6), 4.03 (1H, d, J = 3.2 Hz, H-7), 5.55 (1H, ddd, J = 1.92, 6.60, and 12.65 Hz H-15), 5.85 (1H, d, J = 9.8 Hz, H-2), 6.33 (1H, s, H-22), 6.41 (1H, d, J = 10.4 Hz, H-3), 7.24 (1H, s, H-23), 7.33 (1H, t, J = 1.8 Hz, H-21); 13C NMR (100 MHz, CDCl3): δ 12.9 (C-18), 16.5 (C-30), 17.2 (C-19), 17.6 (C-29), 34.5 (C-11), 39.2 (C-9), 41.5 (C-16), 43.7 (C-5), 47.4 (C-8), 47.6 (C-10), 47.8 (C-4), 49.7 (C-17), 51.8 (COOCH3), 53.1 (COOCH3), 66.3 (C-6), 87.0 (C-7), 87.5 (C-15), 110.5 (C-22), 126.5 (C-20), 126.9 (C-2), 135.0 (C-13), 139.1 (C-21), 143.2 (C-23), 146.9 (C-14), 148.2 (C-3), 173.8 (C-12), 175.6 (C-28), 202.3 (C-1).
After isolating 6-deacetylnimbin from the A5 fraction, its anti-termite activity, which included termiticidal and antifeedant activity, was investigated. The daily termite mortality against C. curvignathus with 6-deacetylnimbin and control was determined for two weeks using the forced feed method or the no-choice test. Furthermore, paper disc consumption by termites after 14 days exposure to the 6-deacetylnimbin was calculated and compared to the control. The results are summarized in Fig. 6(a) and (b). 6-Deacetylnimbin had a higher mortality rate (average 25%) at a dose of 1.67% than the control (solvent only; average 3.41%) after two weeks. 6-Deacetylnimbin caused an average 17.20% loss in paper disc mass, indicating a significantly greater reduction in feeding activity than the control (48.17%).
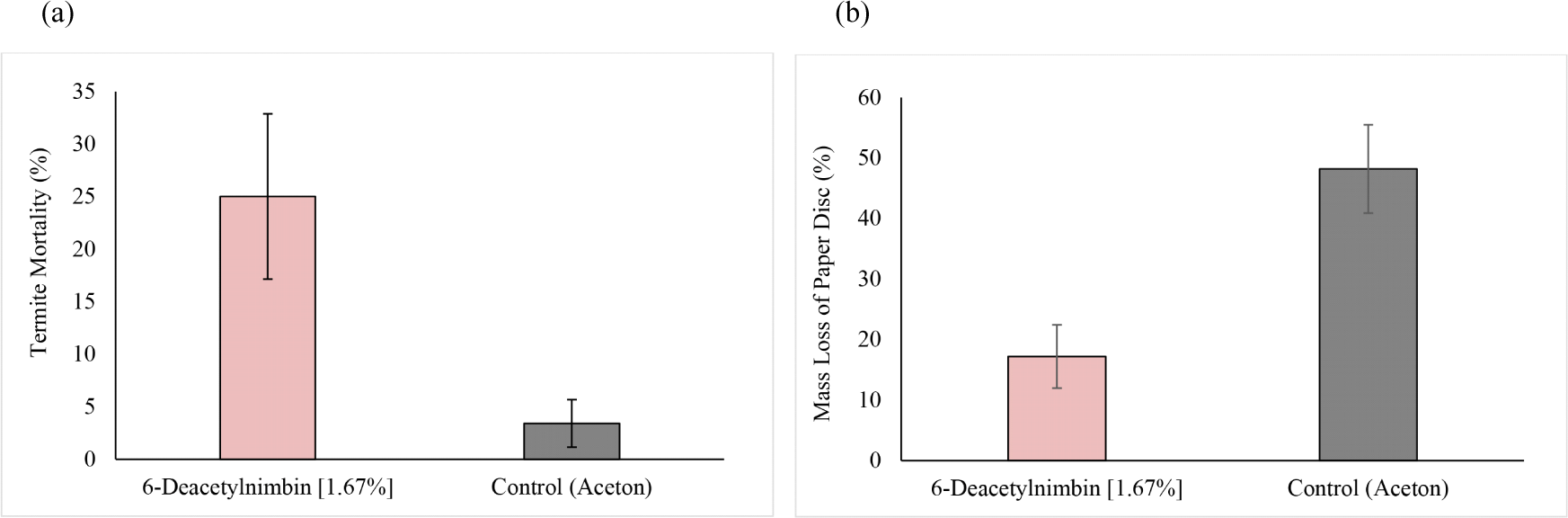
In line with previous studies, 6-deacetylnimbin was discovered to have antifeedant activity against R. speratus and C. formosanus (Adfa et al., 2013; Ishida et al., 1992). The antifeedant and insect growth-regulatory activities of this compound in comparison with azadirachtin have been reported against S. litura, Pericallia ricini, and Oxya fuscovillata. 6-Deacetylnimbin was half as active as azadirachtin as antifeedant but had no detectable growth-regulating activity (Govindachari et al., 1996).
4. CONCLUSIONS
The results showed that A. excelsa seed kernel which is growing in Bengkulu-Indonesia, has anti-termite potential. Its termiticide activity against C. curvignathus was observed in both non-polar and polar extracts. Furthermore, the limonoid 6-deacetylnimbin, a major component of methanol extract, was isolated and found to have termiticidal and antifeedant activity against C. curvignathus. Expectedly, A. excelsa has similar effect on insects as A. indica.