1. INTRODUCTION
The abundance of biomass is currently attracting attention as a source of renewable and sustainable materials for the future. Lignin is the most abundant aromatic biomass comprising 15%–30% of the total aromatic biomass, whereas cellulose is the primary material in woody biomass, accounting for 30%–50% of the total (Kim et al., 2019; Upton and Kasko, 2015; Yang et al., 2017). As the most abundant aromatic compound in the world, lignin has excellent potential as a raw material in various industrial applications such as the manufacture of engineering plastics, foam materials, membranes, nanofibers, hydrogels, adhesives, and other new materials (Bang et al., 2022; Fatriasari et al., 2020; Hwang and Choi, 2018; Hwang et al., 2021; Lee et al., 2019, 2021; Min and Um, 2017; Yang et al., 2019). Among various technical lignins, kraft lignin (KL) extracted from black liquor in the kraft pulping process is the most abundant industrial lignin (Gadhave et al., 2019; Li et al., 2016; Tejado et al., 2007). Lignin precipitation is a possible technique for isolating lignin (Gigli and Crestini, 2020). Acid precipitation of black liquor is a convenient method of extracting KL and is commonly used to extract lignin from black liquor by gradually decreasing the pH with a strong acid (García et al., 2009; Helander et al., 2013; Lourençon et al., 2015; Pang et al., 2021; Wang and Chen, 2013). For example, Helander et al. (2013) used acid precipitation for separating low molecular weight KL from softwood black liquor. Precipitation at low pH produces a relatively higher yield and less heterogeneous lignin from the black liquor (García et al., 2009; Helander et al., 2013; Lourençon et al., 2015; Sun et al., 1999). Furthermore, the lignin precipitation pH significantly influences thermal properties and molecular weight (García et al., 2009). However, there is a clear difference in the molecular weight of KL under alkaline pH (9) as compared with a strong acid pH (2) (Wang and Chen, 2013). Lignin, with a specific molecular weight, and thus defined properties, is needed to enhance its value. High heterogeneity with high polydispersity and aromaticity of industrial lignin causes the chemical structure and contents of certain lignin functional groups to vary to some degree with molecular weight. This issue restricts the potential of lignin to produce value-added products. To solve this problem, various attempts have been made at lignin fractionation (Gigli and Crestini, 2020; Helander et al., 2013; Jiang et al., 2017; Sadeghifar et al., 2017; Wang and Chen, 2013).
Lignin fractionation has been widely studied to produce low polydispersity, low molecular weight, and high lignin purity. Organic solvent fractionation has been reported as a promising technique with the advantage of controlling the molar mass distribution of various lignin fractions (Arefmanesh et al., 2022; Park et al., 2020). Acetone has been investigated as a facile solvent to dissolve lignin. The advantages of using this solvent are low cost, biodegradability, minimization of waste, and good adsorption efficiency (Crestini et al., 2017; Li et al., 2019). For example, Sadeghifar et al. (2017) fractionated organosolv lignin with acetone in water to obtain relatively homogeneous lignin fractions. The fractionated organosolv lignin showed different molecular weights and functions, while higher molecular weight fractions exhibited more aliphatic and less phenolic OH than those of lower molecular weight fractions.
It is important to determine specific precipitation pH levels to obtain proper lignin fractions with high yield and low molecular weight distribution (Wang and Chen, 2013). Acetone fractionation has been proposed to generate acetone-soluble KL (AS-KL) and acetone-insoluble KL (AI-KL) fractions (Gigli and Crestini, 2020). In particular, the development of KL-based adhesives for wood bonding requires the selection of low molecular weight KLs for easy reaction and cross-linking to build a three-dimensional network structure. However, there is limited research on the effects of the precipitation pH and acetone fractionation on the KL characteristics. The aim of this study is to examine the effects of the precipitation pH and acetone fractionation on the characteristics of KL to attain less heterogeneous and low molecular weight KLs for lignin-phenol-formaldehyde (LPF) resins as wood adhesives. Thus, we extracted KLs from the black liquor of mixed hardwood species with precipitation at different pH levels and fractionated the obtained KL with acetone as a green solvent prior to its characterization.
2. MATERIALS and METHODS
Industrial black liquor from the kraft pulping of mixed hardwood species was obtained from a local pulp mill (Moorim P&P, Ulsan, Korea). Sulfuric acid (98%) and acetone were purchased from Daejung Chemicals (Siheung, Korea). Pyridine was obtained from Sigma-Aldrich (St. Louis, MO, USA). Hydrochloric acid and acetyl anhydride were obtained from Duksan Chemical (Incheon, Korea).
The selected pH levels for the precipitation in this study were 3, 5, 7, and 9. KL was precipitated by adjusting the pH of the black liquor with 4 N H2SO4 and then stirring for 1 h at 70°C. Then, the precipitated lignin was separated by filtration using filter paper (Whatman no. 41, Whatman, Maidstone, UK). The precipitated lignin was washed using a 10-folds volume of sulfuric acid (0.4 N) to remove any impurities. The lignin mixture was then stirred again for 1 h at 70°C. Finally, the product was filtered and washed with distilled water (precipitated lignin: distilled water, 1:10). Then, it was dried at 60°C for 72 h to obtain crude KL (C-KL) in a powdered form. The yield of C-KL extracted from black liquor was obtained by drying it for 3 days at 60°C.
Prior to acetone fractionation, the C-KL was washed with distilled water and then stirred at 300 rpm at room temperature for 2 h. Then, the C-KL was filtered with Whatman no. 1 filter paper and dried at 50°C for 36 h. The dried C-KL was stirred (300 rpm) at room temperature for 24 h. Next, the acetone fractionation of C-KL was done to obtain AS-KL and AI-KL by vacuum filtration through filter paper (Whatman no. 41). The AS-KL fraction was recovered by removing the acetone using a rotary evaporator at 45°C. Then, AI-KL and AS-KL were dried for 48 h at 50°C to prepare KL in a powdered form. Finally, the yields of AS-KL and AI-KL were obtained by drying them for 2 days at 50°C.
Gel permeation chromatography (GPC; Waters Alliance e2695 system; Waters, Milford, MA, USA) was used to determine the molecular weight of the KLs. Polystyrene standards with molecular weights ranging from 1,060 Da to 2,330 kDa were used for GPC calibration. The precipitated KL samples were subjected to acetylation to enhance their solubility in tetrahydrofuran (THF). Approximately 2 mg of each sample was dissolved in 1 mL of THF and then sonicated for 5 s before filtering through a 0.45 μm PTFE syringe filter. The column temperature was 35°C under an isocratic THF flow rate of 1 mL/min.
For the acetylation, 300 mg of AS-KL and AI-KL were added to a beaker, followed by 18 mL of pyridine: acetic anhydride (1:1) mixture, and then stirred (200 rpm) at room temperature for 48 h. Then, 250 mL of 0.1 N HCl was added to the solution to precipitate the acetylated KL. It was then filtered using a 0.45 mm Whatman nylon membrane. While filtering, it was washed up to three times with 0.01 N HCl and H2O. As shown in Fig. 1, the acetylation was successful in replacing the hydroxyl groups with acetyl groups. It has been reported that the acetylation process does not influence the lignin structure; therefore, the product is neat lignin (Buono et al., 2016).
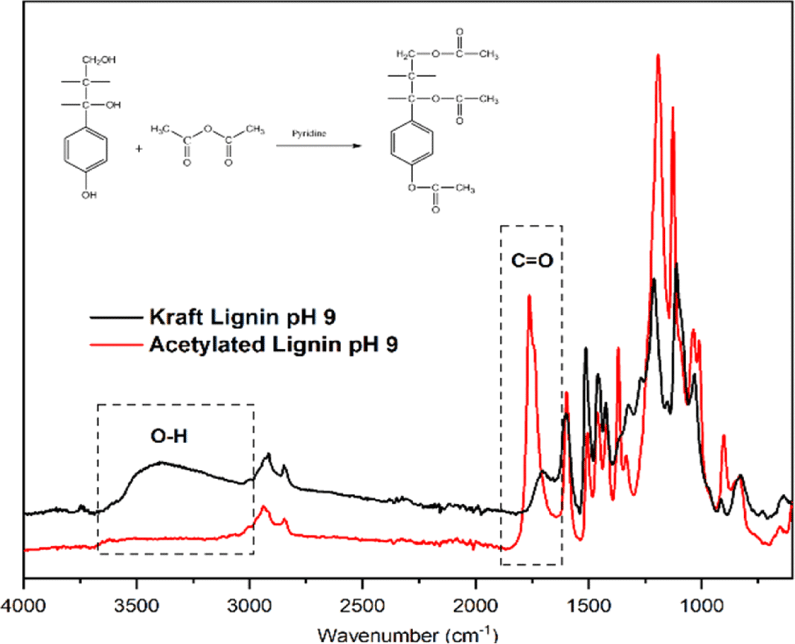
Attenuated total reflectance infrared spectroscopy (ALPHA, Bruker Optics, Ettlingen, Germany) was used to determine the functional groups of KLs precipitated by each pH and acetylated kraft lignin. FTIR spectra were recorded in the range of 4,000–400 cm–1 with a 4 cm–1 resolution and 200 scans.
3. RESULTS and DISCUSSION
As presented in Fig. 2, the yield of C-KL decreased with increased precipitation pH. The precipitation at pH 3 showed the highest yield, whereas that at pH 9 produced the lowest yield, indicating that a lower pH tended to generate a higher yield. The yields at pH 9 and pH 7 were 18.80% and 19.55%, respectively. The yields at pH 5 (23.31%) and pH 3 (25.29%) were quite similar to the reported values (dos Santos et al., 2014; García et al., 2009). For example, García et al. (2009) reported that the acidification of black liquor from pH 6–5 provided an approximate 20% yield. The yield could be increased to 80% at pH 2 (dos Santos et al., 2014).
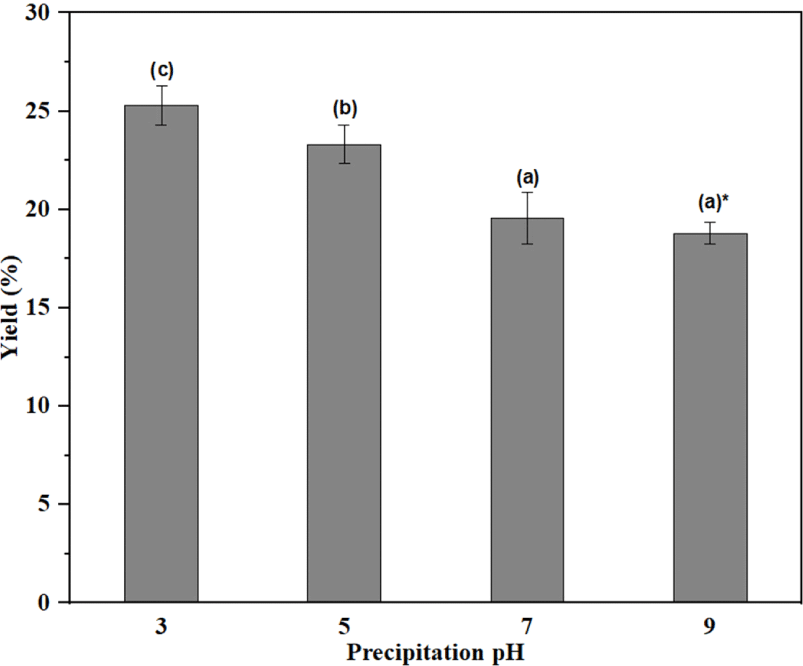
The phenol hydroxyl groups of KL in black liquor are in an ionized state at a very high alkaline pH (approximately 13 to 14). This means that most of the phenolic hydroxyl groups in the KL in black liquor are in a deprotonated state (Helander et al., 2013). When the pH of the black liquor is lowered by adding sulfuric acid, the phenolic hydroxyl groups of KL are protonated and then precipitated. In other words, the KL is precipitated with acid because of the protonation of the ionized phenolic groups of the lignin molecules. The protonation of lignin molecules is more favorable with a higher pKa value, which depends on temperature, ion strength of the solution, type of solvent, and molecular structure of lignin in the apparent pKa of kraft lignin (Zhu et al., 2016). KL precipitated at a high pH produces a low yield with a large lignin molecular weight. When the pH is decreased, the KL precipitated has lower pKa values closer to those of small lignin molecules, thereby increasing the total precipitation yield (Zhu et al., 2016). The authors also reported that the precipitation pH strongly influenced the yield (Zhu et al., 2016). Moreover, it has been found that the molecular weight and functional groups of lignin also play an important role in lignin precipitation (Zhu et al., 2014, 2016).
The KL yield based on this theory was found to vary significantly with precipitation pH level. For example, different precipitation pH levels also resulted in KL fractions with different molecular weights (Lourençon et al., 2015). In addition, the presence of hemicelluloses and other impurities in the raw material could also influence the yields at different pH levels (García et al., 2009). In fact, it has been reported that the KL sample used in this work also contained a small amount of hemicellulose (Wibowo and Park, 2023).
Fig. 3 shows the yield of acetone fractionation at various pH levels. As the pH increased from 3 to 9, the yield of AS-KL increased to a maximum at pH 7 and rapidly decreased at pH 9. In other words, the AS-KL showed the highest yield (96%) at pH 7, whereas the AI-KL showed the lowest yield (21%) at pH 9. The results show that the AS-KL was almost completely dissolved at pH 7. The high yield of AS-KL was quite close to that obtained in another study that reported 70 and 30 wt.% of AS and AI fractions, respectively, for softwood kraft lignin (Arefmanesh et al., 2022). Sadeghifar et al. (2017) also reported that the fractionation of organosolv lignin with 60% acetone concentration produced a 100% soluble fraction, while acetone concentrations lower than 60% led to a partial precipitation.
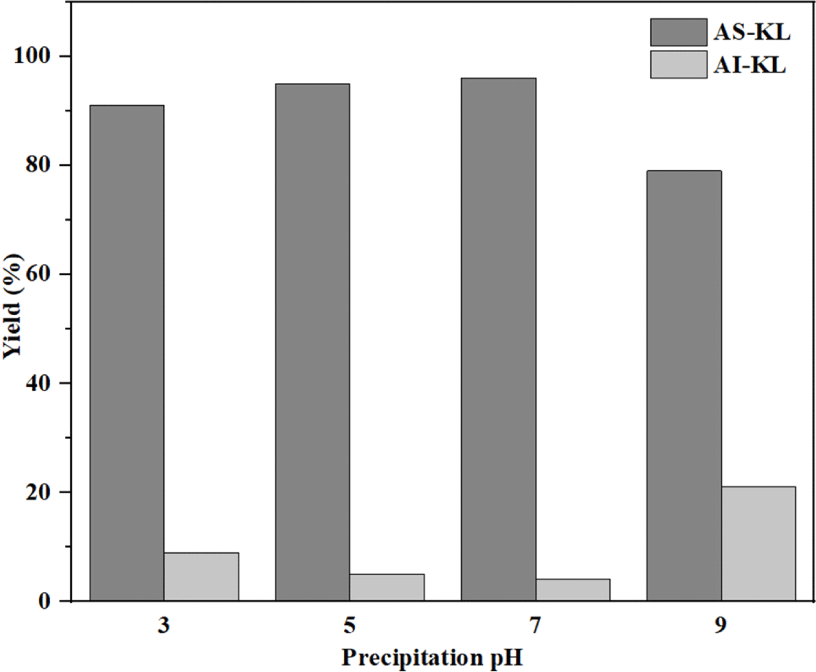
These results indicate that the AS-KL fraction at pH 7 could be a candidate for the synthesis of LPF resins. However, the molecular weight of AS-KL fraction at pH 7 is greater than that of AS-KL at pH 9 (Fig. 4). Therefore, it is believed that the AS-KL fraction at pH 9 has a good potential as a raw material for LPF resins because it provides a relatively good yield and small molecular weight.
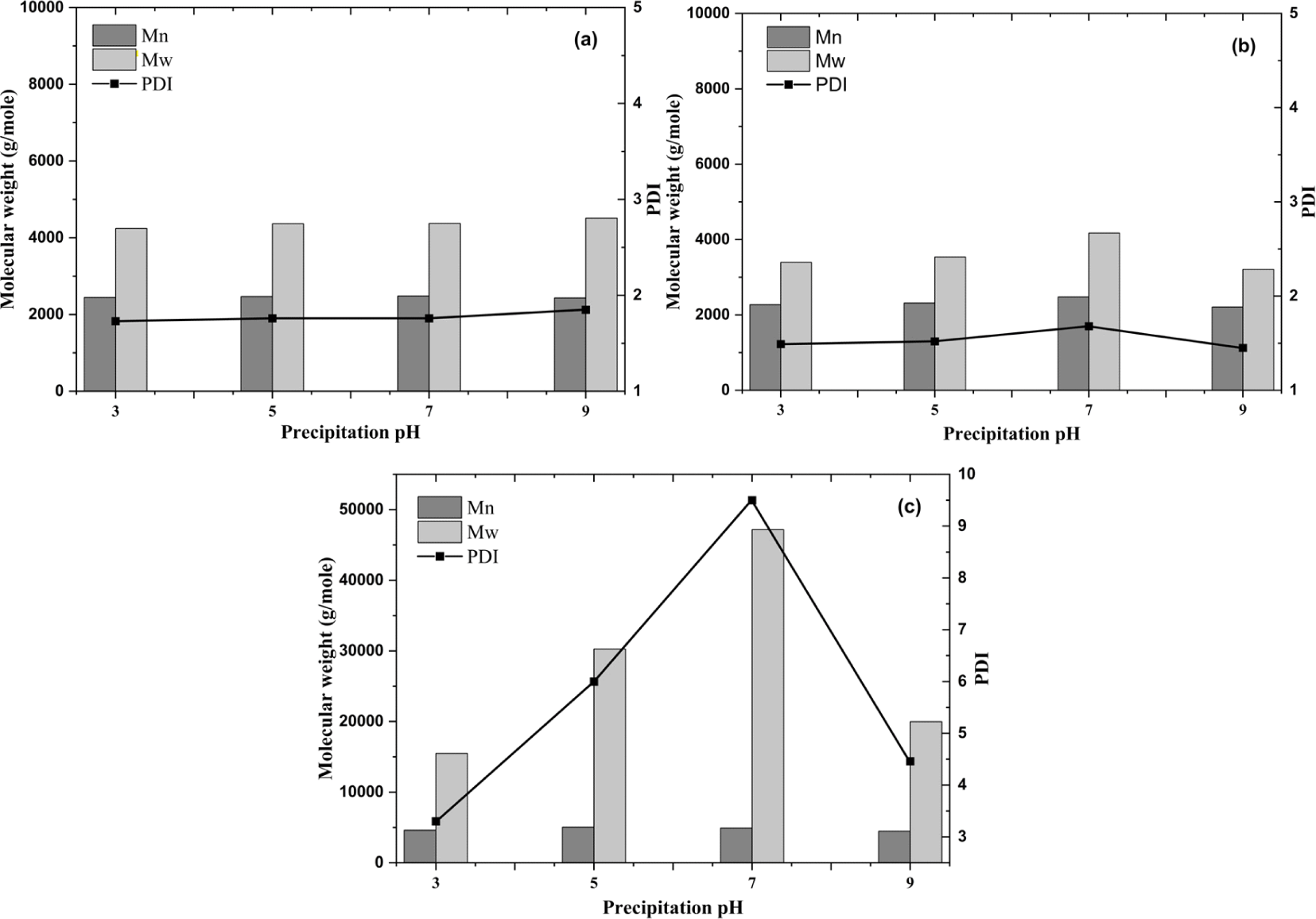
As shown in Fig. 5, the KL FTIR spectra show the main functional groups present in the precipitated KLs. The absorption band assignments of the FTIR spectra are also given in Table 1. In general, the FTIR spectra showed most of the typical vibration bands, such as hydroxyl groups (3,375 cm–1), C-H axial deformation of CH2 and CH3 in aliphatic side chains (2,917–2,845 cm–1), and the various skeletal vibrations of aromatic rings (1,600 cm–1) of C-KL, AS-KL, and AI-KL precipitated at each pH (Lourençon et al., 2015; Mun et al., 2021). This result shows that the intensity of hydroxyl groups at 3,375 cm–1 of C-KL is quite similar for the precipitates obtained at different pH levels [Fig. 5(a)]. This is in contrast to other results that indicated a decrease in the precipitation pH resulted in a high phenolic hydroxyl group intensity (Lourençon et al., 2015; Zhu et al., 2016). García et al. (2009) showed that the functional groups of lignin changed at very low pH such as 0.72. The most visible change in the lignin structure of the AI-KL at pH 9 is related to C-C at 1,107 cm–1 [Fig. 5(c)]. In addition, the peak around 1,031 cm–1 is attributed to hemicellulose and silicate contributions. As expected, the presence of syringyl (1,324 cm–1) and guaiacyl (1,267 cm–1) is also apparent. The spectra of C-KL, AS-KL, and AI-KL indicated that the fractionation changed the intensity of kraft lignin functional groups [Fig. 5(b); Jiang et al., 2017; Pang et al., 2021].
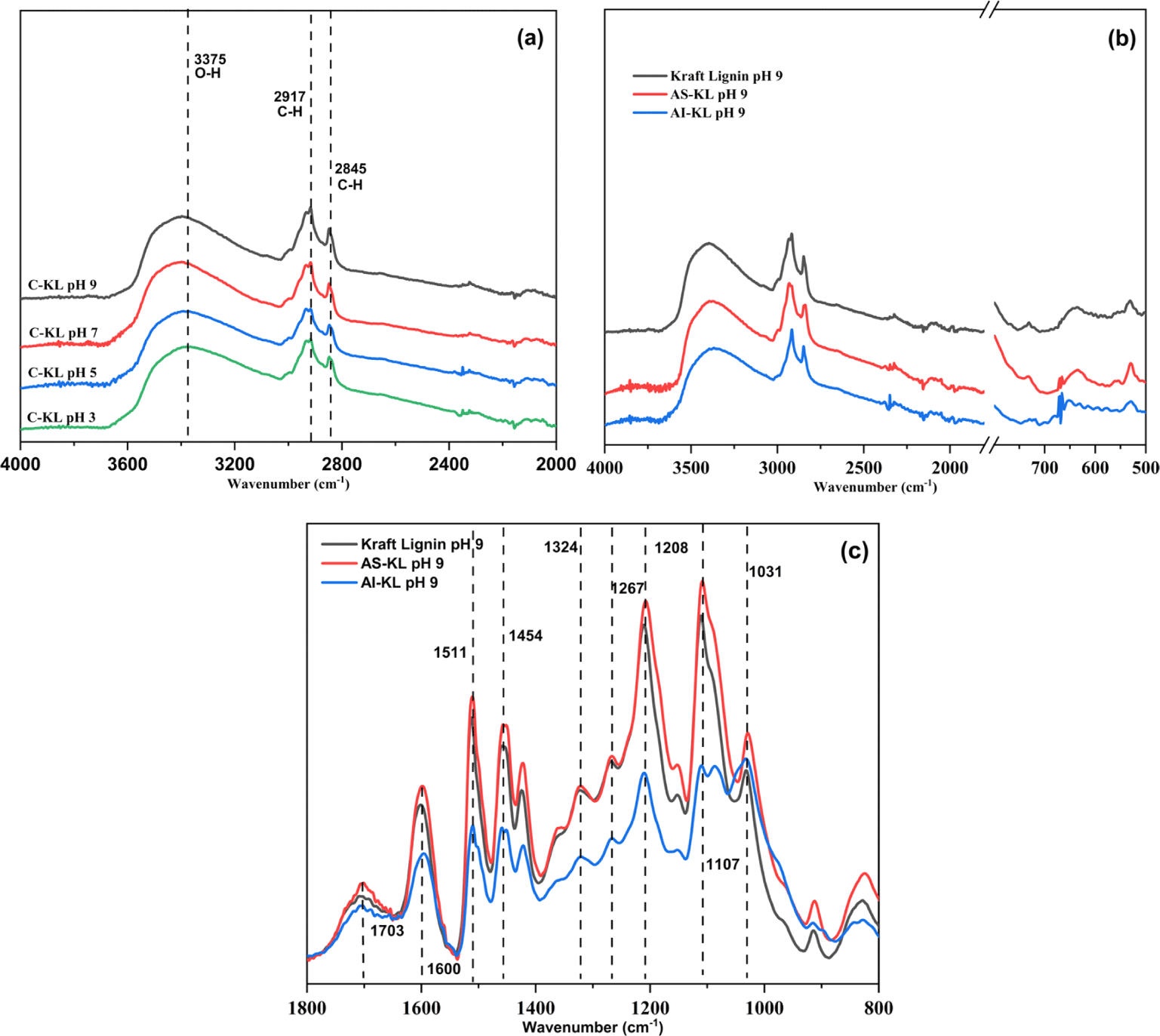
Adapted from Li et al. (2016) with permission of Royal Society of Chemistry.
Adapted from Lourençon et al. (2015) with permission of Elsevier.
Fig. 4 shows the weight average molecular weight, number average molecular weight, and polydispersity index of the samples obtained at different precipitation pH levels before and after acetone fractionation. The molecular weight of C-KL increases as the precipitation pH increased from 3 (4,240 g/mol) to 9 [4,510 g/mol; Fig. 4(a)]. In other words, at low pH, the C-KL contained a more significant amount of lignin with low molecular weight which means large lignin molecules degraded. Therefore, the average molecular weight decreased at low pH (Zhu et al., 2016). Generally, the high pH C-KL is easier to destabilize because the proportion of hemicelluloses is higher, thereby making the molecular weight higher (dos Santos et al., 2014). In fact, Lourençon et al. (2015) reported that higher pH values generated lignin with higher molecular weight, whereas lower pH values generated lignin with lower molecular weight which was compatible with the results of this work. Wang and Chen (2013) also suggested that low molecular weight lignin forms the colloids that contain higher negative charges due to a greater number of negative groups (e.g., phenolic OH and COOH), yielding high colloidal stability over high molecular weight lignin. Moreover, it has been found that the precipitated lignin with low molecular weight had a greater number of phenolic groups (Zhu et al., 2016).
Acetone fractionation produced a relatively low molecular weight for AS-KL compared with AI-KL and C-KL. The same result was reported in a previous study that reported that soluble fractions have lower molecular weight and polydispersity (Wibowo and Park, 2023). The molecular weights of AS-KL and AI-KL at pH 7 increase to maximums of 4,170 and 47,190 g/mol and then decrease to 3,210 and 19,970 g/mol at pH 9, respectively [Fig. 4(b)]. Both AS-KL and AI-KL have low molecular weights of 3,210 and 15,480 g/mol at pH 9 and 3, respectively. The AI-KL at pH 7 had the highest molecular weight [Fig. 4(c)]. This is comparable with other results. They reported that the molecular weight and polydispersity of insoluble kraft lignin fractions were higher than those of unfractionated lignin because there was no sign of substantial aggregation in the acetone solution. These results indicate that the molecular weight of kraft lignin was also affected by the chemical characteristics of the fractionation solvent (Jääskeläinen et al., 2017).
Theoretically, selecting a solvent for lignin fractionation is important because it can gradually alter the polarity of the environment where lignin molecules are solvated and form a condition of complete dissolution (Cui et al., 2014). Lignin fractionation refers to a subdivision of heterogeneous lignin into several fractions with low polydispersity, exhibiting different molecular weight distributions (Pang et al., 2021). The molecular weight of lignin is also explained by its aromatic structure. The number of C-C bonds influences lignin molecular weight in the structures involving C5 in the aromatic ring. Guaiacyl- and syringyl-units are the most abundant aromatic rings in lignin that can generate the C-C bonds that result in high molecular weight (García et al., 2009; Toledano et al., 2010).
In addition, the results of this study showed that the precipitation pH affected the yield and molecular weight of C-KLs and that the acetone fractionation also influenced the molecular weight of the AS-KL and AI-KL fractions. Therefore, the synthesis of LPF resins should have relatively small molecular weight species of KL that facilitate the cross-linking into polymerization. Thus, the AS-KL obtained from pH 9 appears to have good potential for synthesizing LPF resins as wood adhesives.
4. CONCLUSIONS
Acid precipitation of KLs extracted from the black liquor of mixed hardwood species at different pH levels resulted in different characteristics before and after acetone fractionation. Extracted KL provided a higher yield as the pH decreased from 9, 7, 5, and 3. AI-KL showed changes related to the C-C band at 1,107 cm–1 in FTIR spectra. Differences were found in the molecular weights of the products; the high-pH C-KL generated high-molecular weight and low pH C-KL generated low-molecular weight ones. The highest molecular weight was achieved by AI-KL for precipitation at all pH levels, the maximum and minimum being 47,190 and 15,480 g/mol at pH 7 and 3, respectively. The molecular weight of AS-KL pH 9 was found to be 3,210 g/mol, the smallest among the fractions. These results suggest that the AS-KL fractions at pH 9 have a good potential as a raw KL for synthesizing LPF resins in terms of the yield and molecular weight. In addition, the AI-KL fraction at pH 3 also has a potential for synthesizing polyurethane resins because of its relatively high molecular weight and yield.