1. INTRODUCTION
Indonesia cultivates many types of fast-growing wood to overcome the shortage of raw materials and meet the needs of its population. Sengon (Falcataria moluccana Miq.) is a fast-growing wood species widely planted in Indonesia. Sengon wood is a light wood having a specific gravity of 0.33, ranging between 0.24–0.49, and belongs to the strength class IV–V and durability class IV–V (Martawijaya et al., 2005). Typically, sengon wood is typically used as a raw material for making wood crates, particleboards, fiberboards, matches, and handicrafts. The productivity of fast-growing wood is high, but it has several problems, especially those related to physical properties and others (Priadi et al., 2019). Bowyer et al. (2007) stated that fast-growing wood has disadvantages such as low density and durability. Therefore, it is necessary to improve the properties, produce new functions, and enable the broader application of wood.
In this study, we used the impregnation method to improve wood quality. Impregnation using vacuum pressure tubes can improve or enhance the physical properties of wood (Hadi et al., 2018; Hartono et al., 2016; Oh and Park, 2015). Wood impregnation involves introducing chemicals into pores, lumens, and cell walls to precipitate and store chemicals without damaging the wood. Impregnation methods have been applied to fast-growing wood to improve wood quality using chemicals such as methyl methacrylate (Cahyono et al., 2020; Hadi et al., 2019), phenol-formaldehyde (Sumardi et al., 2020), melamine formaldehyde, furfuryl alcohol (FA); (Prihatini et al., 2020), nano TiO2 (Rahayu et al., 2022b) and magnetite (Fe3O4) nanoparticles (Rahayu et al., 2022a).
One of the materials used in manufacturing includes nano iron oxides, such as Fe3O4, widely used as a non-ferrofluid material (Kumar et al., 2010). Magnetite nanoparticles (nano Fe3O4) have been extensively applied in the manufacture of magnetically charged wood because these are easily synthesized and have good biocompatibility (Zhang et al., 2021). Wood impregnated with a magnetic liquid becomes magnetic wood, which has novel magnetic properties (Merk et al., 2014). Magnetic wood can be used as a construction material to retain heat (Oka et al., 2012) and can also be used in energy harvesting, adsorption, and removal of contaminants such as heavy metals, dyes, and organic pollutants (Sezer et al., 2021). In addition, magnetic wood offers many attractive characteristics, such as being able to attract or be attracted to magnets, absorb electromagnetic waves, and increase dimensional stability (Ayrilmis and Kaymakci, 2013; Trey et al., 2014).
Magnetic wood is composed of a combination of wood and a magnetic powder or magnetic liquid. Wood can be magnetized using both ex-situ and in-situ processes. The current study used the ex-situ method to manufacture magnetic wood because this method does not utilize strong and weak base precursors (Dong et al., 2016; Rahayu et al., 2022a) directly as in the in situ method, which could cause the degradation of lignin (Schmiedl et al., 2012), and the materials needed are easy to obtain. The ex-situ method involves impregnation with a solution containing nano Fe3O4, readily available in the market (Gan et al., 2017; Kumar et al., 2021; Tang and Fu, 2020).
The use of nano Fe3O4 to manufacture magnetic wood has limitations because the compound is washed easily out of the wood. In addition, it is unstable, readily agglomerates, and oxidizes (Masoudi et al., 2012; Theerdhala et al., 2010). Magnetic particles dispersed in a liquid have a natural tendency to interact with each other and form aggregates because of the attraction between them (Vennapusa et al., 2008). The weakness can be reduced or prevented by mixing nano Fe3O4 and demineralized water with FA, which acts as a dispersant. The nano Fe3O4 forms a colloidal phase when mixed with FA, which allows nano Fe3O4 to be impregnated more easily into the wood. Mixing with FA is also a practical and environmentally friendly approach for manufacturing magnetic wood. In addition, Dong et al. (2016) reported that using FA protected the nanoparticles from leaching, thereby increasing the dimensional stability of wood and supporting a high percentage of weight gain. This study aimed to analyze and characterize the physical properties of magnetic sengon wood, including density and dimensional stability.
2. MATERIALS and METHODS
A 43-cm-diameter log of sengon wood was sourced from a tree with a branch-free height of 7–9 m harvested in Sukabumi, Indonesia. Other materials used in the study included nano Fe3O4 (particle diameter 297 ± 10 nm; Anhui Elite Industrial, Hefei, China), FA (Sigma Aldrich, St. Louis, MO, USA), and demineralized water.
Test samples measuring 2 × 2 × 2 cm (BS-373:1957) were cut out of sengon wood log. The samples were tested for weight percent gain (WPG), anti-swelling efficiency (ASE), water uptake (WU), bulking effect (BE), and density (ρ). The impregnation solution included demineralized water (untreated), nano Fe3O4 and FA (1:1 mol). In this study, three concentrations of nano Fe3O4 (w/v%) were used, namely 7.5%, 10%, and 12.5%. After the nano Fe3O4, and FA were mixed based on the treatment concentration, the impregnation solution was stirred for 15 min, followed by a sonication (Cole Parmer) with an amplitude of 40% for 30 min.
The impregnation process was initiated by oven-drying the test sample at a temperature of 103 ± 2°C. Subsequently, the dimensions were measured and weighed. The test samples were then placed in a container, followed by adding the impregnation solution into the container. The test sample and solution were then placed into the impregnation tube under a vacuum of –0.5 bar for 2 h, followed by subjection to a pressure of 1 bar for 2 h. When the impregnation process was over, the test samples were wrapped in aluminum foil and dried in an oven at 65°C for 12 h. After drying the test samples for 12 h, we removed the aluminum foil covering the samples and placed the samples back into the oven for drying at a temperature of 103 ± 2°C until the samples achieved a constant weight. The impregnated test samples were then immersed in demineralized water for 24 h and dried in an oven at 103 ± 2°C.
The physical properties of magnetic wood were tested according to Hill (2006) and Bowyer et al. (2007), including WPG, BE, density (ρ), ASE, and WU, using the following formulas:
where,
W0 = Sample oven-dried weight before impregnation treatment (g)
W1 = Sample oven-dried weight after impregnation treatment (g)
W2 = Sample weight after being immersed in water for 24 hours (g)
V0 = Sample oven-dried volume before impregnation treatment (cm3)
V1 = Sample oven-dried volume after impregnation treatment (cm3)
Su = Volume shrinkage of the untreated sample
St = Volume shrinkage of the treated sample
The penetration and distribution of nano Fe3O4 in the wood cell walls were analyzed using SEM (JEOL JSM-6510LA series). The untreated and impregnated wood samples were cut to a size of 0.5 cm × 0.5 cm × 0.5 cm on a tangential plane, placed on conductor adhesive, coated with gold, and observed under SEM at a voltage of 20 kV. Wood samples were also analyzed using EDX to determine the chemical content of the wood (Rahayu et al. 2022a).
Untreated and impregnated wood samples were milled into 200-mesh particles and embedded in potassium bromide pellets. Then, the finished pellets were analyzed using FTIR (Perkin-Elmer Spectrum One) and scanned in the wavenumber range of 4,000–400 cm–1 with a resolution of 4 cm–1 for 32 scans (Rahayu et al. 2022a).
Wood samples were cut into 2-mm-thick pieces in a tangential direction. The degree of crystallinity of the wood sample (incision) was analyzed using an XRD (PANanalytical Empyrean) instrument with a 1D PIXcel detector. The parameters used in the device were Cu Kα radiation with a graphite monochromator, a voltage of 40 kV, a current of 30 mA, and a scan range of 2θ between 5° and 80° for the degree of crystallinity and between 5° and 90° for phase analysis. The scanning speed was set at 2°/min (Rahayu et al. 2022a).
The magnetic loop hysteresis of the differentially treated wood specimens was determined using a Lake Shore 7410 VSM meter (Lake Shore Cryotronics, Westerville, OH, USA) at 300 K outside the magnetic field from –20 to 20 kOe in 100 Oe steps and a mean time of 100 ms. In addition, the saturation magnetization (Ms), coercivity (Hc), and remanence (Mr) were evaluated from the hysteresis curve. The magnetic properties of the samples were evaluated using VSM. The dimensions of the specimens for the magnetic test were 3 mm × 3 mm × 7 mm in the longitudinal direction (Rahayu et al. 2022a).
3. RESULTS and DISCUSSION
The density, WPG, BE, WU, and ASE of untreated and treated samples are presented in Table 1. Compared with untreated wood, the density, WPG, BE, and ASE values of sengon wood treated with Fe3O4-FA increased, whereas the WU value decreased, revealing considerable differences from the values for untreated wood samples. The increased dimensional stability and density observed in this study agree with the results of Dong et al. (2016) and Rahayu et al. (2022a), who reported the same trends in poplar and jabon wood.
The WPG value of treated samples increased with the concentration of Fe3O4-FA, with the 10% Fe3O4-FA treatment resulting in the highest WPG value. The WPG value of sengon wood treated with Fe3O4-FA was higher than that of the untreated wood. The result is in agreement with that of a previous study by Dirna et al. (2020), reporting higher WPG values of fast-growing wood when impregnated with monoethylene glycol and nano-silica, and that of Hartono et al. (2016), also observing the same trend in oil palm trunks when impregnated using phenol–formaldehyde. The WPG of the sample treated using 12.5% Fe3O4-FA is lower than that of sengon treated with 10% Fe3O4-FA because the size of Fe3O4 at a concentration of 12.5% is greater than that at a concentration of 10% (for details see discussion of the XRD analysis). Moreover, the Fe3O4 solution is denser and thicker at 12.5% concentration. Therefore, it is difficult for nano Fe3O4 to enter and penetrate sengon wood, which only covers the surface of the wood at 12.5% concentration. However, based on Duncan’s test results, WPG values between 10% and 12.5% were not significantly different (p-value < 0.01).
The increase in the density of sengon wood in this study was followed by increased WPG value in agreement with the results of Hadi et al. (2022), who reported that the furfurylated sample had a higher density than the untreated sample and the increase in WPG value followed the increase in density. The higher the density of the treated wood, the greater the content that filled the wood cell walls and the thicker the wood cell walls (Bowyer et al., 2007). Wood density is a critical factor in determining the physical properties of wood (Lee and Lee, 2018; Zhang etal., 2018). The impregnation treatment with Fe3O4 and FA solutions resulted in the penetration of these solutions into the wood cell walls, resulting in the formation of bonds with the wood cell wall components, which caused the cell walls to swell and fill the microvoids in the cell walls (Hadiyane et al., 2018; Hill, 2006).
The ASE and BE values tended to increase with treatment. The increase in the ASE value with impregnation treatment was in agreement with Priadi et al. (2020). Adding Fe3O4 and FA to sengon wood makes the treated wood denser and has higher dimensional stability. Prihatini et al. (2020) showed that FA combined with melamine-formaldehyde increased the BE values. The higher the BE value, the more the polymer fills the cell wall, thereby increasing dimensional stability (Hill, 2006). The WU value showed a decreasing trend with treatment. This indicates that less water can enter the wood. The addition of FA can form a barrier on the lumen surface and reduce water absorption. However, the hydrophobic nature of FA also affects its water-repellent properties (Dong et al., 2014).
The morphologies of the untreated and impregnated sengon wood were observed using SEM (Fig. 1). The condition of the wood that initially appeared empty [Fig. 1(a)]. After impregnation, the treated wood looked like nano Fe3O4-FA covering the cell walls, and deposits were also present in the intercellular spaces marked with color (yellow) in Fig. 1(b), (c), and (d). Yellow spots were observed, indicating the presence of nano Fe3O4 and FA on sengon wood treated with Fe3O4-FA 7.5%, 10%, and 12.5%. The presence of nano Fe3O4-FA that enters and covers the wood cell walls has a BE on sengon wood. This can prevent the entry of water into the cell walls of the wood, which is indicated by the decreased WU value.
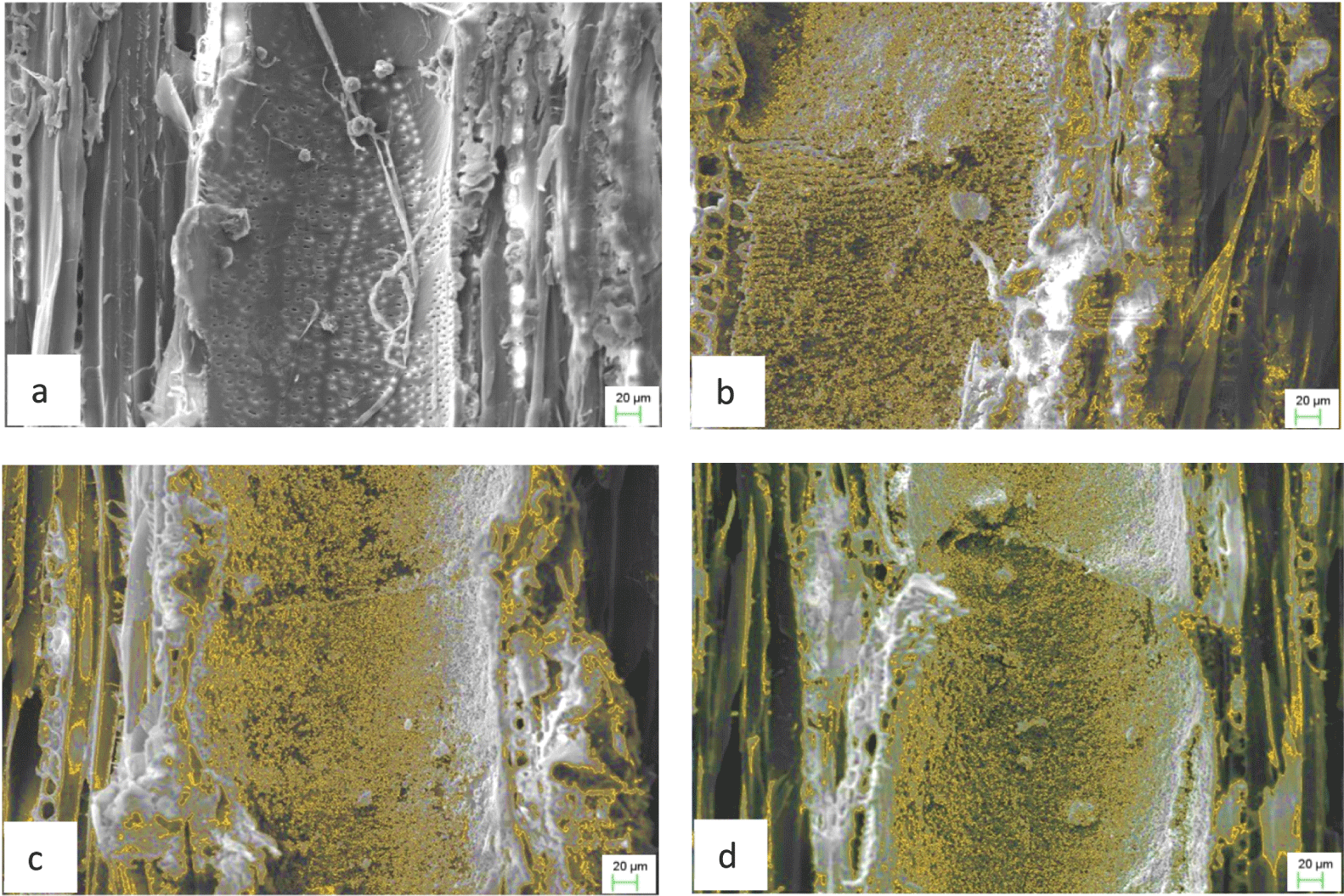
Sengon wood impregnated with Fe3O4-FA caused an increase in the weight of sengon wood, as evidenced by an increase in the WPG value. Based on Dirna et al. (2020), the results of the SEM analysis revealed possible causes of an increase in the value of WPG, ASE, BE, and density and a decrease in the value of WU in treated sengon wood. According to Xue and Zhao (2008), nanoparticles can enter and penetrate wood, particularly through nanosized cavities. Additional information from the EDX analysis in (Table 2) indicates the presence of Fe that enters and covers the cell walls of impregnated sengon wood. The percentage of Fe increased with increasing concentration of Fe3O4-FA solution from 23.53% to 59.63%. The morphological image and the results of the SEM-EDX test showed that nano-magnetite managed to enter, cover, and deposit on the sengon wood cell walls and cavities of the cells.
Treatment | Fe (wt. %) |
---|---|
Untreated | 0 |
7.5% Fe3O4-FA | 23.53 |
10% Fe3O4-FA | 49.07 |
12.5% Fe3O4-FA | 59.63 |
The FTIR results are shown in Fig. 2. The wave numbers 3,337 cm–1, 3,341 cm–1, and 3,336 cm–1 in each sample indicate the presence of O-H stretching functional groups. According to Xu et al. (2019), the O-H functional group is indicated by the wave number 3,200–3,600 cm–1and ranges from 3,570 cm–1 to 3,200 cm–1 (Nandiyanto et al., 2019). The detected peak vibrations ranged from 2,895 cm–1 to 2,922 cm–1, which was associated with the presence of asymmetric C-H functional groups and symmetrical strain vibrations (Gan et al., 2017; Wang et al., 2011), and the peak was observed at the wave number 1,727 cm–1, 1,730 cm–1, and 1,731 cm–1, which are characterized by the C = O stretch of unconjugated ketone and ester groups from hemicellulose, and the side chains of lignin were broken by magnetic treatment (Chang and Chang, 2006; Rahman et al., 2011).
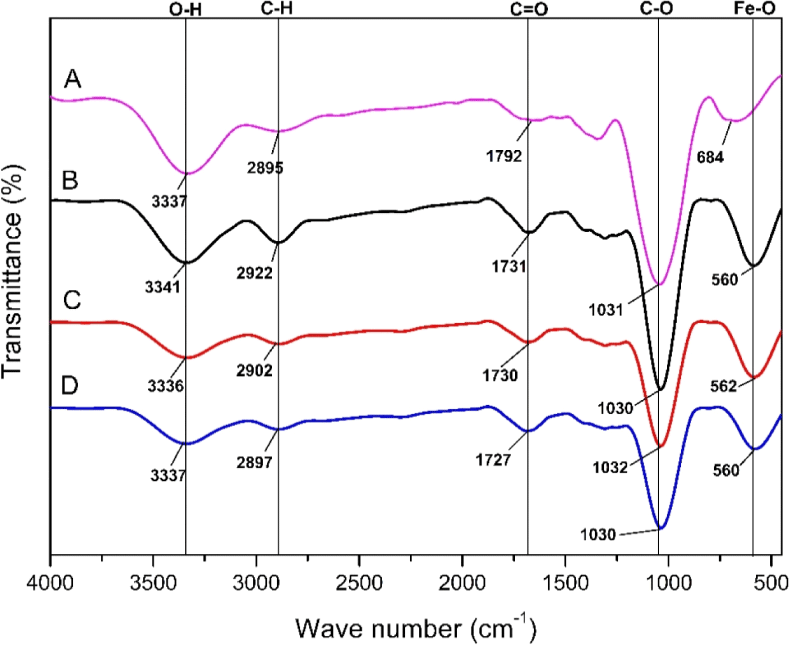
In addition, the weakening of the C-O functional group was also detected in this study, based on the findings of Rahayu et al. (2021) and Wahyuningtyas et al. (2022), who reported a weakening of the peak of the C-O functional group caused by the furan ring from FA at a wave number of 1,030 cm–1, and Xu et al. (2019), who reported a wave number of 1,032 cm–1. Wave numbers of 560 cm–1, 562 cm–1, and 560 cm–1 were seen when the sengon wood was impregnated. This indicates the presence of Fe-O functional groups in the sengon wood at each concentration. This finding indicates that Fe3O4 particles were deposited on the wood substrate. The Fe-O functional group detected in this study agrees with the results reported by Lin and Ho (2014), who found the peak of the Fe-O group at wave number 436–580 cm–1.
Fig. 3 presents the results of the XRD analysis of sengon wood, which has peaks at 2θ values of 21.94° and 34.53° with crystal planes (012); (Rahayu et al., 2022a) and (040) showing cellulose (Cave, 1997; Dong et al., 2016). After the sengon wood was treated, cellulose was still detected, but the degree of crystallinity could not be determined because of the strong magnetite diffraction background (Rahayu et al., 2022a). After sengon wood was treated, the two peaks were weakened, and the latter one may be covered by other peaks, indicating that part of the crystal structure in the wood was damaged by magnetic treatment (Dong et al., 2016). Several quasi-crystalline regions in hemicellulose and cellulose contribute to wood crystallinity (Bhuiyan et al., 2000).
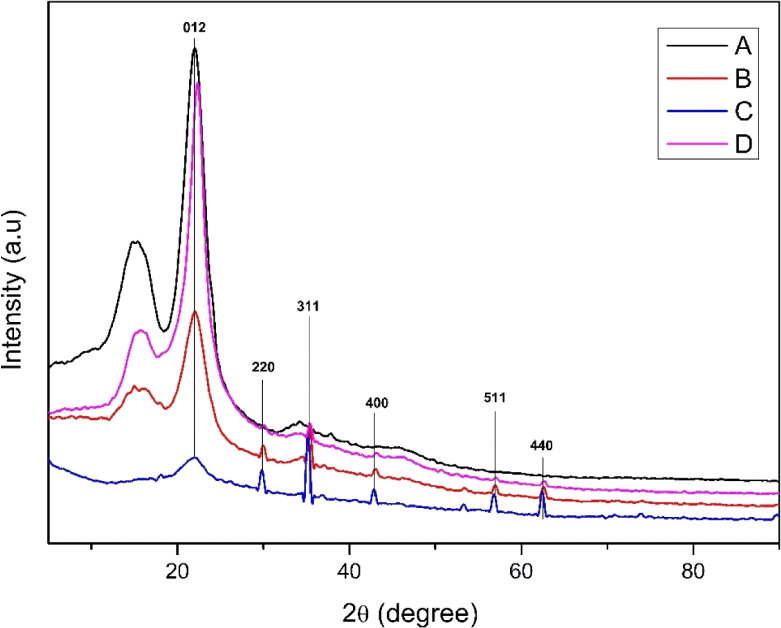
Wood impregnated with Fe3O4-FA produced new peaks, indicating the formation of magnetite compounds in the wood. These peaks occur at the values of 2θ 35.35°, 35.20°, and 35.46° with the crystal plane (311); values of 2θ 43.00°, 42.82°, and 43.10° with crystal planes (400); values of 2θ 43.00°, 42.82°, and 43.10° with crystal planes (400); values of 2θ 56.94°, 56.81°, and 56.92° with crystal planes (511); and values of 2θ 62.57°, 62.36°, and 62.67° with crystal planes (440); (Wu et al., 2011). Fe3O4 peaks occur at approximately 30.1°, 35.4°, 43.1°, 53.4°, 56.9°, and 62.5°, based on the Joint Committee on Powder Diffraction Standard (JCPDS) magnetite card (No. 19-0629); (Yu and Kwak, 2010). The result is in agreement with the findings of Rahayu et al. (2022a) and Dong et al. (2016), who showed the presence of magnetite compounds in the presence of these peaks. After the treatment, all diffraction peaks belonging to Fe3O4 and wood were present in the magnetic wood, indicating that the Fe3O4 magnetic particles were successfully deposited on the wood substrate (Gan et al., 2017).
The size of Fe3O4 concentrations of 10% and 12.5% in sengon wood was determined based on the XRD diffractogram using the Scherrer equation (Lin and Ho, 2014).
where λ is the X-ray wavelength (0.15418 nm), K is the Scherrer constant (0.89), β is the peak full width at half maximum (FWHM), and θ is the Bragg diffraction angle (Dong et al., 2016). Based on the calculation results, the size of the 10% Fe3O4 concentration was 31.72 nm and the 12% concentration was 41.25 nm. The resulting size was in the nanoscale. According to Khan et al. (2019), if the size is in the range of 1–100 nm, it is classified as nanosized. The most important physical properties of materials that affect solubility are particle size, crystals smaller than 1 m, and high surface area can increase solubility (Cornell and Schwertmann, 2006).
VSM testing of treated sengon wood produces a curve called a magnetization curve or hysteresis loop. The magnetic hysteresis curves of the sengon wood samples treated with various Fe3O4-FA concentrations are shown in Fig. 4. In addition, when an object is exposed to an external magnetic field or the magnetic field is removed, the hysteresis curve has a reverse current that is almost symmetrical and is also observed in the area of the hysteresis curve (Tebriani, 2019). The area of the hysteresis curve indicates the energy required for magnetization. The hysteresis curve generated by VSM is characterized by saturation magnetization (Ms), remanence (Mr), and coercivity (Hc).
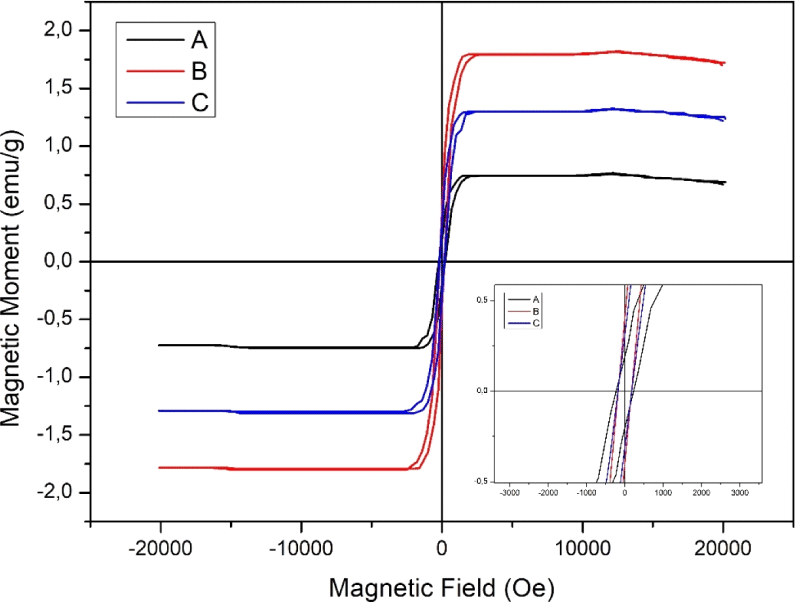
The values of Ms-, Mr-, and Hc-treated sengon wood are shown in detail in Table 3. The Ms values for sengon wood with Fe3O4-FA concentrations of 7.5%, 10%, and 12.5% were 0.685, 1.710, and 1.248 emu/g, respectively. Magnetic sengon wood made with a 10% concentration had a higher Ms value, while the concentration of 7.5% was the lowest. According to Gao et al. (2012), the Ms value is related to the WPG value; the higher the WPG value, the higher the Ms value. The different saturation magnetization values are caused by several factors, namely, the grain size of the nanoparticles and the degree of crystallinity (Riyanto, 2012; Setiadi et al., 2013). The correlation between the WPG and Ms is shown in Fig. 5. The WPG value shows a linear correlation with the Ms value, indicating that the higher the WPG value, the greater the Ms value obtained. This is evident from the coefficient of determination, which is close to 1 (Chicco et al., 2021) and 0.9968. Similar results were reported by Dong et al. (2016) and Rahayu et al. (2022a). The Ms value in this study was lower than that of bulk nano-magnetite (Gan et al., 2017).
Treatment | Ms (emu/g) | Mr (emu/g) | Hc (Oe) |
---|---|---|---|
7.5% Fe3O4-FA | 0.685 | 0.192 | 2.28 × 10–7 |
10% Fe3O4-FA | 1.710 | 0.409 | 1.87 × 10–6 |
12.5% Fe3O4-FA | 1.248 | 0.310 | 1.08 × 10–7 |
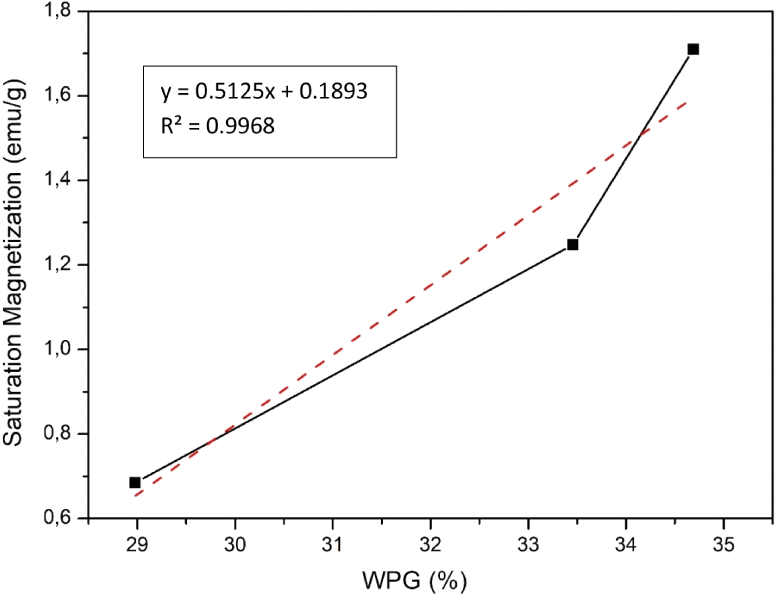
The Mr values for sengon wood impregnated using Fe3O4–FA concentrations of 7.5%, 10%, and 12.5% were 0.192, 0.409, and 0.310 emu/g, respectively. The Mr values in this study were greater than those in Rahayu et al. (2022a) and Moya et al. (2022), which stated that the Mr values of magnetic wood impregnated using the in-situ method were 0.01 emu/g and 0.01 to 0.25 emu/g, respectively. The Mr value increased with increasing concentrations of the Fe3O4–FA solution, although, at a concentration of 12.5%, the Mr value decreased. The result agrees with the observation of Wicaksono et al. (2013) that the greater the Fe3O4 concentrations in the formation, the greater the residual magnetism.
The Hc values for sengon wood with Fe3O4–FA concentrations of 7.5%, 10%, and 12.5% were 2.28 × 10–8 Oe, 1.87 × 10–6 Oe, and 1.08 × 10–7 Oe, respectively. The Hc value of sengon wood treated with Fe3O4–FA was classified as the low coercivity field value. This can be said to be low because the Hc value is almost zero or small and the area of the hysteresis curve tends to be narrow and slender. This indicates that the magnetic sengon wood may have superparamagnetic properties, as can be seen from the Hc value, which is close to zero (Hui et al., 2008; Rahayu et al., 2022a; Xu et al., 2005). In addition, magnetic sengon wood was included in the soft magnetic-type category (Karbeka et al., 2020; Marta et al., 2020; Widanarto et al., 2015). Soft Magnetic Properties are easily influenced by the external magnetic field, which is easily magnetized, and the magnetic intensity easily returns to a value of 0 when the external magnetic field is removed (Wicaksono et al., 2018).
4. CONCLUSIONS
Impregnation treatment of sengon wood with nano Fe3O4 and FA concentration of 10% in several parameters, such as WPG, BE, and density, is optimal for the time being. The parameters of physical properties have an increasing trend (WPG, BE, density, and ASE) and a decreasing trend for WU. SEM results showed changes in the morphology of sengon wood, with the presence of Fe (yellow color), which covers and deposits the cell walls in the wood. The results of FTIR analysis showed the presence of Fe-O functional groups in the treated wood. The treated wood exhibited new peaks, indicating the formation of magnetite compounds in the wood (XRD analysis). The sengon wood treated with Fe3O4–FA was classified as soft magnetic with superparamagnetic properties.