1. INTRODUCTION
Trees of the Quercus genus are distributed across the southern regions of South Korea including Jeju-do as well as Japan and China. The genus consists of five species: Quercus myrsinifolia, Quercus gilva, Quercus acuta, Quercus glauca, and Quercus salicina (Kim et al., 2020). These trees are recognized as the recommended species for afforestation against global warming, while their distribution has extended to the regions in the northern hemisphere due to global warming (Kira, 1991). Among the five species, Q. gilva is mostly planted as a garden tree or used as a material for window frames and handles. In addition, the acorns of the Quercus trees have long been used as an ingredient of acorn jelly for food in the southern regions according to evidential data. For chemical composition, acorns are composed mostly of starch (approximately 70%) with tannins accounting for approximately 6%–9% (Kwon et al., 2002; Lee et al., 1992). Tannins have the characteristic acerbic taste and as they are a type of polyphenols that exist in varying forms and the main component exhibiting an antioxidant effect (Darley-Hill and Johnson, 1981), it is predicted that they may serve valuable not only in food production but also as a potential functional resource.
With increased interest in health, the use of natural plant resources with antioxidant effects but without side effects in the human body has been actively investigated among a diversity of health functional food materials (Barlow, 1990; Branen et al., 1975; Ebrahimzadeh et al., 2014). The increase in reactive oxygen species (ROS) including superoxide, nitric oxide, hydroxyl, peroxyl, alkoxyl, and hydroperoxyl radicals, as a result of stress or drug use, has been pointed out as a cause of disease (Block, 1993; Feskanich et al., 2000; Halliwell and Gutteridge, 1990). An adequate level of ROS provides signal molecules that can regulate cellular responses, but an excess amount of ROS is able to destroy the main cellular components; sugars, DNA and proteins, to cause such diseases as cancer and diabetes as well as aging (Lee et al., 2021; Mun et al., 2020). So far, various ongoing studies are actively exploring the bioactivity and the antioxidative, antimicrobial and anti-inflammatory effects of natural materials in the plant body (Hidayat et al., 2019; Manurung et al., 2019; Yang et al., 2019), and the identified natural antioxidants are vitamins, quercetin, kaempferol, flavonoids such as naringin, tannins such as catechin, phenolic acids, gallic acid, and ascorbic acid (Chen et al., 1996; Mira et al., 2002; Yang et al., 2022). These compounds are known to exert antioxidant effects in the body through the removal of oxidative products or genetic regulation. The demand for natural antioxidant materials to be identified is continuously increasing. For the Quercus trees, studies have reported on phenolic contents, but only the leaves have mainly been investigated, and there is a general lack of studies on the functional materials with antioxidant effects in these trees.
In addition, as the Earth rotates through day and night in a 24-hour cycle, biological organisms on the Earth have developed a 24-hour biorhythm attuned to the changes in day and night. This is the circadian physiological rhythm in the body of biological organisms, generated through rhythmical genetic expressions of the biological clock found in each cell. The circadian rhythm is endogenously regulated by the hormones secreted by the suprachiasmatic nucleus in the hypothalamus, while the exogenous factors include the environment and individual’s behaviors. On the molecular level, the proteins found in the negative feedback loop; CLOCK (circadian locomotor output cycle kaput), BMAL1 (Brain and muscle ARNT-Like 1), PER (Period) and CRY (Cryptochrome), regulate the circadian rhythm (Lellupitiyage Don et al., 2020). In this study, the effect of the Q. gilva extract on the circadian rhythm was investigated in relation to the real-time activity of the clock gene Bmal1 promoter, and the correlation between the circadian rhythm and the antioxidant activity was determined.
In the extraction from a natural material, the detected components and contents vary according to the type of solvent and the extraction method (Ella Nkogo et al., 2022; Jung et al., 2021). In this study, hot water and ethanol were used to obtain extracts from the leaves, branches, kernels, and pericarp of Q. gilva, and in varying extraction conditions, the antioxidant activity, circadian rhythm, and plant components were analyzed. In this way, this study aimed to provide the basic scientific data for identifying novel natural antioxidant materials that can regulate the cellular circadian rhythm.
2. MATERIALS and METHODS
For the test plant Q. gilva, samples were collected from Jinju-si, Gyeongsangnam-do, South Korea. The acorn and leaf samples collected for each tree species were air-dried in a shaded area, then the weight, length and width were measured (Table 1).
The leaves, branches, kernels, and pericarp of each tree species were washed, dried and ground to prepare the samples for subsequent analyses. To test the activity of each part of Q. gilva according to the extraction method, hot water and 95% ethanol were used as extraction solvents. For ethanol extraction, 30 g of Q. gilva sample was added to 1,000 mL of 95% ethanol for 72 hours of extraction at room temperature, while the extraction was repeated three times to obtain an adequate amount of extract solutions. For hot water extraction, 20 g of Q. gilva sample was added to 600 mL of distilled water (D.W.) for 20 min autoclaving at 0.25 MPa, 121°C, using an autoclave reactor (HB-506-4, Hanbaek Sci., Bucheon, Korea). The extract solutions were filtered using Whatman No. 2 paper, then the crude extracts after vacuum evaporation (Rotavapor R-100, Buchi, Flawil, Switzerland) were freeze-dried for use as experimental samples.
The antioxidant activity test involved the test of 2,2-diphenyl-1-picrylhydrazyl (DPPH) radical scavenging activity and the test of 2,2’-azino-bis (3-ethylbenzothiazoline-6-sulfonic acid) (ABTS+) radical scavenging activity. For the DPPH radical scavenging activity, the method of Blois MS (Blois, 1958) was modified and used. To 20 μL sample, 180 μL of 0.15 mM DPPH solution (dissolved in methanol) was added and left to react for 30 min in a dark room. To measure the residual amount of DPPH, the absorbance was measured at 517 nm using the UV-vis spectrophotometer. Ascorbic acid was used as the positive control in a comparative analysis at the concentration range between 3.125 - 100 μg/mL. The radical scavenging activity of the sample was calculated using Equation (1).
A: Absorbance of the DPPH sample group
B: Absorbance of the control group
For the ABTS+ radical scavenging activity, the method of Jeong et al. (2009) was modified and used. The 1:1 mixture of 7 mM ABTS dissolved in D.W. and 2.45 mM potassium persulfate was diluted with PBS (pH 7.4) so that the absorbance reached 0.70 ± 0.02. The solution was then left for 12–16 h in a dark room. To 800 μL of the diluted radical stock solution, 200 μL of the sample in each varying concentration was added, then after 15 min, the absorbance at 730 nm was measured using the UV-vis spectrophotometer. Ascorbic acid was used as the positive control in a comparative analysis at the concentration range between 3.125 - 100 μg/mL. The radical scavenging activity of the sample was calculated using Equation (2).
A: Absorbance of the ABTS sample group
B: Absorbance of the control group
The HaCaT cell, a human skin cell line that expresses mouse Bmal1 promoter-luciferase (obtained from Dr. Kramer, Charite Universitatsmedizin Berlin, Berlin, Germany) was treated with 100 nM dexamethasone for 2 h, then after replacing the medium with the culture solution containing the extract and 100 μM luciferin, the luciferase activity was measured in real-time using a luminometer (Kronos HT, ATTO, Tokyo, Japan) for four days. Next, the bioluminescence activity was determined using the detrending data processing and the respective graph was drawn.
The HaCaT cell (Thermo Fisher Scientific, Waltham, MA, USA), a type of human epidermal keratinocytes, was cultured using Dulbecco’s modified Eagle’s medium (DMEM, Hyclone, Logan, UT, USA) containing 10% fetal bovine serum (Hyclone) and 1% penicillin/streptomycin, in a 37°C incubator with continuous supply of 5% CO2 for retention culture.
For the cytotoxicity analysis, HaCaT cells were cultured in a 96-well plate for 24 h or more, and as the cell growth reached 90% or above, the cells were treated with the extracts for 24 h. The culture solution was then removed, and the cells were washed three times with phosphate-buffered saline (PBS, iNtRON Biotechnology, Seongnam, Korea). According to the manufacturer’s manual, the Cell Counting Kit-8 (Dojindo, Kumamoto, Japan) was subsequently applied, and the absorbance was measured at 450 nm (Infinite 200 Pro, Tecan, Männedorf, Switzerland) to estimate the cytotoxicity based on cell viability.
The yield of the extraction from the Q. gilva sample using 95% ethanol and hot water was tested to confirm the optimum extraction conditions. The extraction yield was calculated in percentage for the amount of the raw material used in the extract preparation after freeze-drying each extract and estimating the dry weight [Equation (3)].
W1: The weight of dry extract
W2: The weight of the plant powder
To measure the total polyphenol content, the Folin-Denis method (Folin and Denis, 1915) was modified and used. To 10 μL of each sample solution, 200 μL of Na2CO3 was added for a 3 min reaction, after which 10 μL of D.W and 10 μL of Folin-Ciocalteu’s phenol reagent were added for a 30 min reaction at 37°C. The absorbance was measured at 750 nm using the microplate reader (EPOCH, Biotek Instrument, Winooski, VT, USA). Gallic acid (GAE) was used as the reference material to draw the calibration curve, and the total polyphenols was expressed as GAE/g.
For quantitative analysis of polyphenols contained in the extracts, HPLC (Nexera X2, Shimadzu, Kyoto, Japan) was performed. The extracts obtained from four parts of Q. gilva in two different solvents were prepared to the concentration of 30 mg/mL and filtered using a 0.45 μm syringe filter before use. For the HPLC, C18 column (Quasar 50 × 2.1 mm, 1.7 um, PerkinElmer, Waltham, MA, USA) was used, and the mobile phase was water (0.1% formic acid, A) and acetonitrile (0.1% formic acid, B). The analytic conditions were, based on the mobile phase (B), the gradient condition was 0.5 min: B (5%), 1 min: B (5%), 10 min: B (95%), 18 min: B (95%); the injected amount of the sample was 5 μL; the reference materials were protocatechuic acid (purity 98%, catalog no. CFN97568, Lot no. CFS202002), catechin (purity 98%, catalog no. CFN99646, Lot no. CFS202101), ellagic acid (purity 98%, catalog no. CFN98716, Lot no. CFS202003), epicatechin (purity 98%, catalog no. CFN 98781, Lot no. CFS202003), epicatechin gallate (purity 98%, catalog no. CFN98570, Lot no. CFS202002), gallic acid (purity 98%, catalog no. CFN99624, Lot no. CFS20 2101), isoquercitrin (purity 98%, catalog no. CFN98753, Lot no. CFS202101), kaempferol–3–O-(2’-6’-di–O–trans- p–coumaroyl)-beta-D-glucopyranoside (purity 98%, catalog no. CFN92386, Lot no. CFS202001), myricitrin (purity 98%, catalog no. CFN99840, Lot no. CFS202003), quercetin (purity 98%, catalog no. CFN99272, Lot no. CFS202102), rutin (purity 98%, catalog no. CFN99642, Lot no. CFS202101) and tiliroside (purity 98%, catalog no. CFN98026, Lot no. CFS202002), all of which were purchased from ChemFaces.
To measure the total flavonoid content, the method of Zhishen et al. (1999) was modified and used. To 25 μL of sample, 100 μL of D.W. and 7.5 μL of 5% NaNO2 were added for a 5 min reaction, and then 15 μL of D.W. and 15 μL of AlCl3 were added for a 6 min reaction, after which 50 μL of 1M NaOH was added for an 11 min reaction at 37°C. The absorbance was measured at 510 nm using the microplate reader (EPOCH, Biotek Instrument). Catechin was used as the reference material to draw the calibration curve, and the flavonoids was expressed as CAE/g.
To measure the total tannin content of Q. gilva extracts, the method of Duval and Shetty (2001) was modified and used. To 1 mL of each extract, 1 mL of 95% ethanol and 1 mL of D.W. were added, and after thorough mixing, 1 mL of 5% sodium carbonate solution was added, then the mixture was left to stand at 25°C for 3 min. Next, 1 mL of 1N Folin-Ciocalteu phenol reagent was added for a 50 min reaction in a dark room. The absorbance was measured at 700 nm using the microplate reader (EPOCH, Biotek Instrument). Tannin acid was used as the reference material to draw the calibration curve, which was used to calculate the content of tannin acid so that the total tannins was expressed as mg TAE/g (TAE: tannin acid equivalent). For each extract, measurements were taken in triplicate and the mean was obtained.
Each experiment was conducted in triplicate, and the mean ± SD was obtained as result values. For the respective statistical analysis, the SPSS 12.0.0 for windows (SPSS, Chicago, IL, USA, 2003) program was used to perform Duncan’s one-way ANOVA. The two-way ANOVA was used to test the significance of the analyzed antioxidant activity. The values with p < 0.05 were regarded as being statistically significant.
For the cytotoxicity analysis, the GraphPad Prism was used to analyze the significant of results through one-way ANOVA and Dunnett’s multiple comparisons test.
3. RESULTS and DISCUSSION
The antioxidant activity of Q. gilva was measured based on the DPPH radical scavenging activity and the ABTS radical scavenging activity. The activities in the hot water and ethanol extracts from the four parts (leaves, branches, kernels, and pericarp) of Q. gilva are presented in Fig. 1.
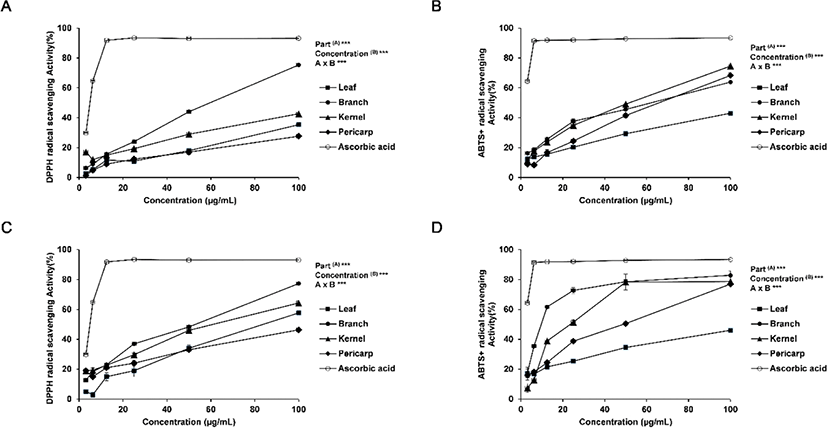
For the DPPH radical scavenging activity, the radical scavenging activity of ascorbic acid as the positive control was 93% at 25 μg/mL, while that of ethanol extract was the highest at 24% for the branch extract and in the range of 11%–19% for the leaf, kernel and pericarp extracts. The radical scavenging activity of hot water extract was the highest at 36% for the branch extract as with the ethanol extract and in the range of 19%–30% for the leaf, kernel and pericarp extracts. An overall concentration-dependent increase in the radical scavenging activity was observed, with statistical significance within the concentration range of 3.125 - 100 μg/mL. In both ethanol and hot water extracts, the branch extract exhibited the highest radical scavenging activity; the hot water extract showed a higher antioxidant activity than the ethanol extract.
For the antioxidant activity based on the ABTS radical scavenging activity, the result was similar to that of the DPPH radical scavenging activity. The highest antioxidant activity of 38% was shown by the branch extract in ethanol, while the leaf, kernel and pericarp extracts fell in the range of 20%–35% radical scavenging activity. The radical scavenging activity of hot water extract was the highest at 73% for the branch extract as with the ethanol extract, which was close to ascorbic acid at 92%. Overall, the antioxidant activity was higher in the hot water extract than in the ethanol extract, with the branch extract exhibiting the highest radical scavenging activity. The differences in the activity are presumed to be due to the differences in the extract composition according to the part of the tree species and the extraction method (Lapornik et al., 2005; Pinelo et al., 2005).
In addition, the differences between the ABTS radical scavenging activity and the DPPH radical scavenging activity may be attributed to the fact that, while DPPH obtains an electron from the active material in the extract to turn into a non-radical form to generate anionic radicals, ABTS is reduced by the active material to generate cationic radicals (Yu et al., 2002). The two methods, therefore, led to variations in the result due to the variations in the binding between the reactant and the substrate (Lee et al., 2012).
In Moon et al. (2009), the antioxidant activity in the ethanol extract of Q. gilva branch was monitored. In comparison, this study has examined the antioxidant activity in the ethanol as well as hot water extracts from varying parts of Q. gilva. It is noteworthy that the level of radical scavenging activity in the hot water extract but not in the ethanol extract was as high as the activity of ascorbic acid, the the positive control, suggesting a potential high level of bioactivity of the hot water extract from the branch of Q. gilva.
The cytotoxicity of Q. gilva was measured in the hot water and ethanol extracts from four parts of Q. gilva (Fig. 2). Following the treatment of the cells with the hot water extract, the cytotoxicity was measured after 24 hours. The result showed that, for the leaf (Fig. 2E) and kernel (Fig. 2G) extracts, cytotoxicity was not found until 1,000 μg/mL but from 5,000 μg/mL. Cytotoxicity was observed from 1,000 μg/mL for the branch extract (Fig. 2F) and from 100 μg/mL for the pericarp extract (Fig. 2H). In the case of ethanol extract, the cytotoxicity was observed from 1,000 μg/mL for the leaf (Fig. 2A), branch (Fig. 2B) and kernel (Fig. 2C) extracts, and from a far lower level of 10 μg/mL for the pericarp extract (Fig. 2D), whereby the cytotoxicity slowly increased so that from 100 μg/mL, the cell viability fell below 20%.
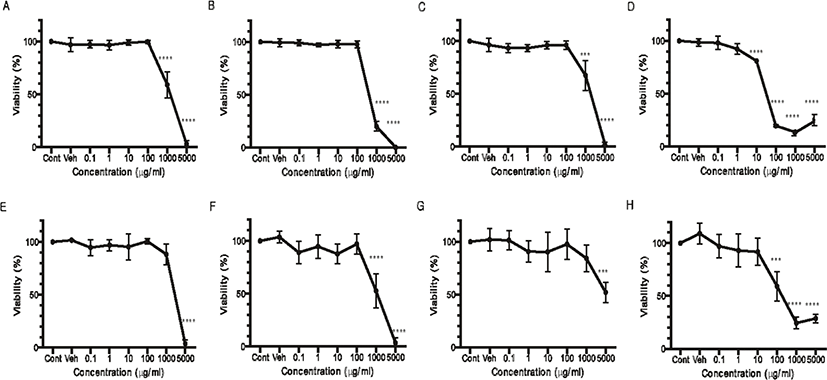
The pattern of the clock gene Bmal1 reporter expression was measured in real-time based on the promoter activity after four days of treatment with the extracts from four different parts of Q. gilva. The concentration applied in the treatment was the maximum concentration without cytotoxicity and 1/10 of the maximum concentration. For the hot water extract of leaves, branches, kernels, and pericarp of Q. gilva, no significant change in the cellular circadian rhythm was observed at 1/10 of the maximum concentration (Fig. 3E–H).
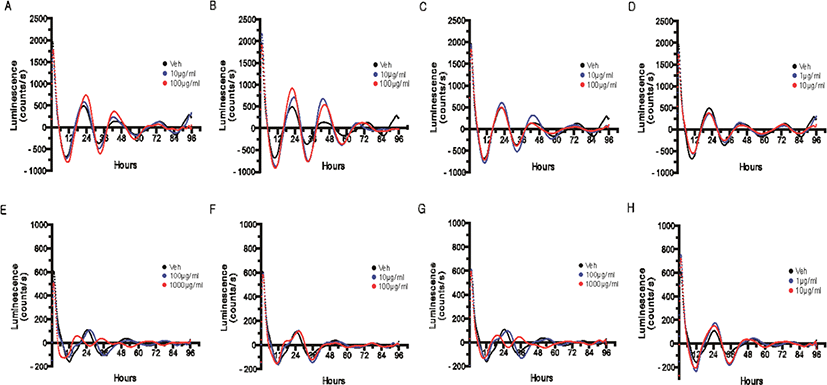
After the treatment with the hot water extract of leaves (Fig. 3E) and kernels (Fig. 3G) of Q. gilva, a change in the inherent waveform of circadian rhythm or lack of circadian rhythm was observed at the maximum concentration without cytotoxicity. This is presumed to be because, at the maximum concentration without cytotoxicity, certain cells underwent apoptosis or stress induction to prevent the circadian rhythm or cause a sudden change in its waveform.
In the case of ethanol extract, a concentration-dependent increase in the amplitude of the cellular circadian rhythm was observed upon the treatment with the branch extract (Fig. 3B) and a minute increase in the amplitude upon the treatment with the leaf extract (Fig. 3A).
An increase in the amplitude may be taken to indicate the reinforcement of the circadian rhythm itself (Gloston et al., 2017; He et al., 2016). As the circadian rhythm was measured in a system based on the expression of the clock gene reporter according to the activity of the promoter, the increase in amplitude in this study may indicate the enhanced promoter activity and the consequent increase in the overall dynamicity of the circadian rhythm. As the dynamicity of the circadian rhythm may fall with aging and stress, which entails abnormal expressions of various genes under the influence of the circadian rhythm, the reinforcement and recovery of the dynamicity of the circadian rhythm can be regarded as the reinforcement of the overall cellular homeostasis.
The extraction yield for each tested part of Q. gilva and in the two extraction solvents is presented in Table 2. The yield was shown to be higher in the hot water extracts than the ethanol extracts for the branch, kernel and pericarp regions, which is presumed to be due to the high pressure in the hot water extraction process deforming or destroying the tissues and cell walls to exude high contents of active materials in the tissues and cells. The result suggested that, for certain parts of Q. gilva, the high-pressure hot water extraction could be an efficient method to increase the extraction yield.
The polyphenolic compounds in natural materials comprise phenolic acids and flavonoids that are known to exhibit antioxidative and anticancer effects (He et al., 2021; Kwon et al., 2001). For the four different parts of Q. gilva in two extraction solvents, the total polyphenol, flavonoid and tannin contents were quantitatively analyzed (Table 2). The reference materials; gallic acid, catechin and tannin acid, were used as the control. The total polyphenol content was the highest at 49.77 mg/g in the hot water extract from the branch of Q. gilva, which was approximately 3-fold higher than the ethanol extract. The total polyphenol content was low for the leaf extract in both hot water and ethanol. The highest content was found for the branch extract in hot water and the kernel extract in ethanol.
Flavonoids are capable of ROS scavenging to delay or prevent aging so that their contents serve as the data to indicate the level of antioxidant activity (Kuwata et al., 1993).
The total flavonoid content in the branch of Q. gilva was approximately 2-fold higher at 34.25 mg/g in the hot water extract than in the ethanol extract. As with the total polyphenol content, the result is indicative of higher antioxidant activity in the hot water extract of Q. gilva branch. The total tannin content was also the highest in the the hot water extract of branch; the level was higher by 10-fold or above than the ethanol extract of pericarp. Overall, the hot water extracts showed higher contents of total polyphenols, flavonoids and tannins than the ethanol extracts to indicate variations according to the extraction solvent and process. Ultimately, the contents were higher in the hot water extract of Q. gilva branch, and in light of the previous result of DPPH or ABTS radical scavenging activity, the findings coincided with Cencioni et al. (2013), where the total polyphenol and flavonoid contents increased as the radical scavenging activity increased.
For the detailed analysis of the polyphenolic components found in the extracts of Q. gilva leaves, branches, kernels, and pericarp, HPLC was performed (Table 3). Variations were found according to the extraction solvent and the tested part of Q. gilva regarding the content of each polyphenolic component. The gallic acid content was the highest in the hot water branch extract, while catechin, epicatechin, and gallic acid were shown to be the indicators of the antioxidant activity of Q. gilva. In the case of catechin and epicatechin, high contents in tea leaves have been reported, with a role to suppress the release of free radicals and prevent oxidative stress (Bernatoniene and Kopustinskiene, 2018; Tvrda et al., 2019). GAE is also known to be a powerful antioxidant with a role to suppress free radical production (Badhani et al., 2015).
The result on circadian rhythm in this study showed that the ethanol extract of Q. gilva branches led to a change in the circadian rhythm. While there are only a few reported materials related to the circadian rhythm regulation, flavone-based compounds are expected to be the potential regulators of the circadian rhythm (Cha et al., 2019). Based on the HPLC result, the highest levels of catechin and epicatechin at 37,511 mg/kg and 37,433 mg/kg were found in the ethanol branch extract, which predicted that catechin and epicatechin could serve as the indicators to increase the contents of the effective materials that induce changes in the cellular circadian rhythm. In addition, according to a recent report, catechin accumulation increased during the day and decreased during the night with an influence on the circadian rhythm regulation (Wang et al., 2021). Furthermore, catechin is also known to be effective in the improvement of cognitive impairment caused by sleep deprivation (Forouzanfar, 2021).
In addition, among the phenolic compounds detected in the ethanol extract of Q. gilva branches, myricetin was reported to reduce melatonin synthesis by inhibiting serotonin N-acetyltransferase (Shin et al., 2013) and ellagic acid was reported to alleviate memory impairment and anxiety caused by sleep deprivation (Wang et al., 2020). Despite the variations in the molecular clock that generates the circadian rhythm between animals and plants, the rhythmical transcription of the clock genes and the overall rhythmical expression of clock genes through the regulation via a feedback loop are fundamentally shared. For plants, in particular, flavonoids are known to be closely associated with the expression of clock genes. Quercetin, in particular, has been shown to influence the transcription of CCA1, a central clock gene in plants (Hildreth et al., 2021).
4. CONCLUSIONS
This study investigated the antioxidant activity, bioactivity, components and circadian rhythm of the hot water and ethanol extracts of the leaves, branches, kernels, and pericarp of Q. gilva among the Quercus trees naturally growing in South Korea, with an aim to verify the potential development of the functional materials in Q. gilva.
As a result, a high level of antioxidant activity was detected in the hot water extract of the branch of Q. gilva. The antioxidant activity was higher in the hot water extracts than in the ethanol extracts for most tested parts of Q. gilva.
The total polyphenol, flavonoid and tannin contents were all higher in the hot water branch extract than in any other extracts, and a detailed component analysis showed that the Q. gilva extracts had high contents of gallic acid, catechin and epicatechin. The antioxidant activity increased as the poly-phenolic contents increased, with a correlation between polyphenols and antioxidant activity.
The cytotoxicity was generally low across the hot water and 95% ethanol extracts of the four parts of Q. gilva, suggesting that the extracts themselves may be applied for anti-oxidation and circadian rhythm regulation without the problem of cytotoxicity. Notably, the ethanol extract from Q. gilva branches displayed a low level of cytotoxicity as well as an effect to increase the amplitude of the circadian rhythm in a concentration-dependent manner.
While the antioxidant effect was higher in the hot water branch extract, the effect on the circadian rhythm regulation was most distinct in the ethanol branch extract. This implied that the regulation of the circadian rhythm depended on specific materials rather than simply on the flavonoid content. Based on the results in this study, the contents of catechin and epicatechin were relatively higher in the ethanol extract from Q. gilva branches than from any other parts. In addition, although catechin and epicatechin have not been reported to directly regulate the circadian rhythm, they have been shown to indirectly regulate the circadian rhythm as in the improvement of the reduced cognitive ability caused by sleep deprivation. The two materials are thus predicted to engage in the regulation of the clock genes directly on the molecular level, and the findings of further studies could be highly valuable.