1. INTRODUCTION
Candida is a common fungus that exists on the skin, as well as in the gastrointestinal and urinary tracts, and it is a representative fungal infectious agent as an opportunistic pathogen for humans (Enoch et al., 2006; Pfaller and Diekema, 2007; Soll et al., 1991; Yapar, 2014). The strain most commonly isolated from Candida-infected patients is C. albicans, suggesting that it is the major causative agent of Candida infection (Ng et al., 2015; Pfaller and Diekema, 2007). C. albicans causes candidemia, Candida vaginitis, oral candidiasis, and invasive candidiasis in the human body. Among these diseases, invasive candidiasis has a mortality rate of 46%–75% (Brown et al., 2012; Richter et al., 2005; Sandven, 2000; Taylor and Raja, 2021). Oral candidiasis leads to dysgeusia, dysphagia, loss of appetite, and weight loss (Sharon and Fazel, 2010) and candida vaginitis decreases the quality of life of women due to symptoms such as odor, itching, fever, and difficulty urinating (Landers et al., 2004). Therefore, it is important to develop a therapeutic agent for C. albicans infection.
It has been reported that the biological mechanisms behind the activity of antifungal agents against C. albicans include inhibition of the synthesis of ergosterol, destruction of the membrane structure by binding to ergosterol, and inhibition of glucan synthesis (de Oliveira Santos et al., 2018). Fluconazole is commonly used for treating candidiasis because it exhibits higher efficacy at lower concentrations than other antifungal agents (Saag and Dismukes, 1988). Fluconazole inhibits ergosterol synthesis by acting on sterol demethylase, which transforms lanosterol into ergosterol (Vincent-Ballereau et al., 1991). However, antifungal-resistant C. albicans infections are being reported frequently and fluconazole-resistant C. albicans has been more widely reported than other resistant Candida species (Espinel-Ingroff et al., 2014; Pfaller et al., 2008b; Pristov and Ghannoum, 2019; Rex et al., 1995; Whaley et al., 2017). In addition to the problem of resistant Candida, given that fungi are eukaryotes like humans, treatment is always associated with a particular risk of side effects (de Oliveira Santos et al., 2018).
Many studies have been conducted to overcome the limitations of antifungal agents (Zida et al., 2017), mainly focusing on triazoles (Cuenca-Estrella et al., 2004; González et al., 2009; Irfan et al., 2017; Park et al., 2004b; Sanglard and Coste, 2016), peptides (Dartevelle et al., 2018; do Nascimento Dias et al., 2020; Lum et al., 2015; Ramamourthy et al., 2020; Wang et al., 2015), and plant compounds (Bernardes et al., 2012; Bona et al., 2016; Chen et al., 2013; D’Auria et al., 2005; Lemar et al., 2005; Patel and Coogan, 2008; Serra et al., 2018; Shai et al., 2008; Tampieri et al., 2005; Violante et al., 2012).
Plants that have been used as herbal medicines for a long time are relatively safe even when used as antifungal agents. Cynomorium coccineum has antifungal efficacy not only against C. albicans, but also against C. tropicalis, C. krusei, C. guilliermondii, C. parapsilosis, and C. neoformans (Gonçalves et al., 2015). Acetone extracts of Punica granatum L., Quercus suber L., and Vicia faba L. showed the minimum inhibitory concentration (MIC) at 0.090, 0.105, and 0.010 mg/mL, respectively, for C. albicans (Akroum, 2017). However, natural plant extracts tend to be less effective than conventional chemical antifungal agents. The MIC ranges for C. albicans of fluconazole, amphotericin B, voriconazole, and caspofungin were reported to be 0.25–32, 0.03–0.25, 0.03–0.25, and 0.015–0.25 μg/mL, respectively (Eksi et al., 2013). The MIC values of ethanol extracts of the plants Trachyspermum ammi, Teucrium polium, Piper nigrum, Pistachia vera, and Camelia sinensis were between 6.25 and 25 mg/mL (Masomi and Hassanshahian, 2016). Meanwhile, the MIC values of alcoholic extracts of the plants Lawsania inermis, Portulaca oleracea, Salvadora persica, and Asphodelus tenuifolius were between 10 and 50 μg/mL (Soliman et al., 2017). Recently, the antifungal activity of Cinnamomum loureirii Nees (Lee et al., 2021) and C. parthenoxylon (Adfa et al., 2020) has also been reported. In addition to plants, the fungi, Pestalotiopsis theae and Curvularia sp., also produced antifungal chemicals (Hidayat et al., 2019). Antifungal activity derived from natural products provides an eco-friendly method for inhibiting mold (Ham et al., 2021; Hidayat et al., 2019; Jung, 2019; Suprapti et al., 2020; Yun et al., 2021) that causes wood deterioration.
In order to effectively use the antifungal activity, many studies have been conducted to show synergistic antifungal activity by mixing the compounds. The antifungal efficacy of D-penicillamine increased against C. albicans by mixing with fluconazole (Li et al., 2019). As plant extracts, a synergistic antifungal effect against C. albicans and Aspergillus niger was reported by mixing clove, cumin and caraway essential oils or by mixing their major active components (Hassan et al., 2020).
Phellodendron amurense grows in China, Korea, Japan, Vietnam, and eastern Russia. Its bark, Phellodendri Cortex, has been used for treating meningitis, cirrhosis, dysentery, pneumonia, and tuberculosis (Ryuk et al., 2012; Sun et al., 2019). In recent studies, Phellodendri Cortex was shown to have anti-inflammatory (Fujii et al., 2017), anti-viral (Kim et al., 2016), anti-diarrhea (Xu et al., 2020), neuroprotective (Xian et al., 2013), and anti-diabetic activities (Tian et al., 2020).
Magnolia officinalis is a large tree found in central China, the bark (Magnoliae Cortex) of which has been used as a medicine for treating abdominal swelling and anxiety in East Asia and Southeast Asia for more than 2,000 years (Luo et al., 2019). Pharmacological studies have indicated that Magnoliae Cortex has a wide range of therapeutic effects, including antimicrobial (Chan et al., 2008), anticancer (Saito et al., 2009), analgesic (Luo et al., 2019), anti-inflammatory (Ni et al., 2020), and antioxidant activities (Chan et al., 2008; Luo et al., 2019). Moreover, Magnoliae Cortex and Phellodendri Cortex have been reported to have antifungal efficacy against C. albicans (Chan et al., 2008; Park et al., 1999).
In this study, we propose a synergistic treatment by mixing two extracts that achieves higher antifungal efficacy at a lower dose. Combination therapy has the advantage of increasing the antifungal efficacy, enabling the treatment of infections with antifungal-resistant strains, reducing the emergence of resistant strains, and minimizing side effects by reducing the dose of agents (Baddley and Pappas, 2005). However, there have been few studies on synergistic antifungal activities between plant extracts. In this study, the synergistic efficacy of antifungal activity by mixing Magnoliae Cortex and Phellodendri Cortex extracts, which are strong antifungal active extracts discovered from the extract library (Yoon and Kim, 2021), was evaluated.
2. MATERIALS and METHODS
C. albicans KCTC 7965 was purchased from Korean Collection for Type Cultures in the Korea Research Institute of Bioscience and Biotechnology (Jeongeup, Korea) and stored at −80°C with 15% glycerol.
Phellodendri Cortex and Magnoliae Cortex were purchased from Jiundang Oriental Pharmacy (Seoul, Korea).
RPMI 1640 (catalog number: R6604-10X1L) and MOPS[3-(N-morpholino)propanesulfonic acid] (catalog number: M1254-250G) were purchased from Sigma-Aldrich (Seoul, Korea). Tween 20 (catalog number: 6571-1405) and sodium hydroxide (catalog number: 7571-4400) were purchased from Daejung Chemicals & Metals (Siheung, Korea). Sodium chloride (catalog number: S0476) and dimethyl sulfoxide (DMSO) were purchased from Samjeon Chemical (Seoul, Korea), and Duksan Pharmaceutical (Ansan, Korea), respectively. Berberine (catalog number: B0451) and magnolol (catalog number: D3971) were purchased from Tokyo Chemical Industry (Tokyo, Japan). Amphotericin B (catalog number: J61491.MC) was purchased from Fisher Scientific Korea (Seoul, Korea).
For the water extracts, the herbal plants were minced into pieces of 1 mm or less, 10 g of which was immersed in 100 mL of distilled water. Extraction was performed at 100°C for 4.5 h using a reflux extractor (EP1010-250P; Labdia, Seoul, Korea), and the solid content was removed by filtration using Whatman® Qualitative Filter Paper (Grade 1; GE Healthcare Life Science, Seoul, Korea). The filtered extract was dried using a freeze dryer (FDU-1200; Sunileyela Co., Ltd., Seongnam, Korea) after the removal of water in a rotary evaporator (RV 10 digital V, catalog number: 00100047 99; IKA® Korea, Seoul, Korea) at 90°C.
For the ethanol extracts, the herbal plants were minced into pieces of 5 cm or less, 30 g of which was immersed in 300 mL of 95% ethanol. The plants were extracted at 50°C in a water bath for 3 h. After extraction, the solid particles were removed using Whatman® Qualitative Filter Paper (Grade 1, catalog number: 1001-0155; GE Healthcare Life Science). The extracts were concentrated at 50°C using a rotary evaporator (RV 10 digital V; IKA® Korea) and dried in a dryer at 60°C for 2 weeks.
For the carbon dioxide supercritical extraction, Phellodendri Cortex and Magnoliae Cortex were purchased from Sunil Herb (Hongcheon, Korea). Each extract was produced by EnSNature (Anseong, Korea). Phellodendri Cortex (3,290 g) and Magnoliae Cortex (3,200 g) were added to a 10 L supercritical extractor, and extracted for 6 and 5 h, respectively, using supercritical carbon dioxide at 350 bar and 50°C. Impurities were removed using Whatman® Qualitative Filter Paper (Grade 4; GE Healthcare Life Science).
C. albicans was cultured according to previous studies (Yoon and Kim, 2021). A Sabouraud’s dextrose (SD) agar plate was prepared by adding 1.5% w/v agar (catalog number: 214010, BD Biosciences Korea, Seoul, Korea) to 3% w/v SD broth (catalog number: 238230, BD Biosciences Korea). C. albicans was streaked on SD agar plates and incubated at 35°C for 1 day twice in order to obtain a single colony before cultures. RPMI 1640 medium was prepared with 0.165 mol/L MOPS, and the pH was adjusted to 7.0 with 1 M sodium hydroxide.
Antifungal activity of samples was evaluated according to the CLSI M27-A2 method (Pfaller et al., 2008a). Phellodendri Cortex water extract (PC-WE) was dissolved in RPMI 1640 medium to a concentration of 50 g/L and then centrifuged at 11,400×g for 10 min to remove undissolved solids. Magnoliae Cortex carbon dioxide supercritical extract (MC-CDSE) was dissolved in RPMI 1640 medium with 50% v/v of Tween 20 to a concentration of 100 g/L and then centrifuged at 11,400×g for 10 min to remove undissolved solids. Berberine and magnolol were dissolved in DMSO to concentrations of 80 and 75.09 mM, respectively.
To evaluate the antifungal effect, PC-WE was diluted 5 times with RPMI 1640 medium, and then diluted 2 times sequentially with RPMI 1640 medium. MC-CDSE was diluted 50 times with RPMI 1640 medium, and then diluted 2 times sequentially with RPMI 1640 medium and 1% Tween 20. Berberine and magnolol were diluted 50 times with RPMI 1640 medium, and then diluted twice sequentially with RPMI 1640 medium and 2% v/v DMSO.
One hundred microliters of the prepared sample were dispensed into a Corning® 96-well Clear Round Bottom Polystyrene Not Treated Microplate (catalog number: 3788; Corning, New York, NY, USA). As a solvent control, RPMI 1640 medium for PC-WE test, RPMI 1640 medium with 0.5% Tween 20 for MC-CDSE test, and RPMI 1640 medium with 1% DMSO for berberine and magnolol test were used. To measure the absorbance of the sample itself in the medium, 100 μL of the corresponding medium was dispensed in the wells instead of 100 μL of the bacterial inoculation.
C. albicans from a single colony was suspended in 0.145 mol/L sodium chloride and vortexed for 15 s. The absorbance of the suspension was measured at 530 nm using an Optizen 2120 UV Plus spectrophotometer (Mecasys, Daejeon, Korea), and the cell concentration was adjusted to 0.5 McFarland standard. The prepared cell suspension was diluted 1,000-fold with RPMI 1640 medium, so that the cell concentration was 1 × 103 to 5 × 103 CFU/mL. One hundred microliters of the diluted cell suspension was dispensed into wells and incubated at 35°C for 48 h. The cell density was measured by the absorbance at 540 nm using a SynergyTM LX Multi-Mode Reader (BioTek Instruments Korea, Seoul, Korea).
Synergistic antifungal activity was evaluated by microdilution checkerboard assay (Sun et al., 2009). The sample was prepared in the same manner as described in the above section “Evaluation of antifungal activity” with dilution with RPMI 1640 medium to four times higher than the final treatment concentration. The synergistic antifungal activity of sample mixture was measured using Corning® 96-well Clear Round Bottom Polystyrene Not Treated Microplate (product number: 3788; Corning) with 50 μL of each diluted sample. C. albicans cells were inoculated and incubated in accordance with the CLSI M27-A2 method in the same manner as described in the above section “Evaluation of antifungal activity”. The cell density was measured by the absorbance at 540 nm using a SynergyTM LX Multi-Mode Reader (BioTek Instruments Korea, Seoul, Korea).
The antifungal activity was expressed as relative growth inhibition (GIsample), which was calculated as the decrease in cell concentration (ODsample) of the sample relative to the cell concentration (ODcontrol) of the untreated control group.
When the GIA+B of the mixture of two samples was more than twice the GIA of one sample and the GIB of the other sample, it was judged as evidence of synergistic antifungal activity.
Synergistic antifungal activity:
The levels of berberine and magnolol in extracts were analyzed by high-performance liquid chromatography, using the instrument used in a previous study (Yoon and Kim, 2021) with YMC-Triart C18 (catalog number: TA12S05-2546WT; YMC Korea, Seongnam, Korea) as the analytical column. The operating conditions for the analyses of both berberine and magnolol were similar to those in a previous study (Kim and Kim, 2004), with minor modifications.
The mobile phase used for berberine analysis was a solution of water:acetonitrile = 1:1(v:v) with 3.4 g/L potassium dihydrogenphosphate and 1.7 g/L sodium lauryl sulfate. Analysis was performed under isocratic conditions with a flow rate of 1 mL/min, detection of berberine using absorbance at 345 nm, column temperature of 40°C, and total analysis time of 60 min.
When analyzing magnolol, the mobile phase was a solution of acetonitrile:2% acetic acid = 1:1 under isocratic conditions with a flow rate of 1 mL/min. Magnolol was detected using absorbance at 289 nm, column temperature of 30°C, and total analysis time of 60 min.
The disruption of cell integrity was evaluated by measuring the extracellular DNA concentration, following a slightly modified version of a process used in previous studies (Bennis et al., 2004; Rajkowska et al., 2016; Zorić et al., 2017). Phosphate-buffered saline (PBS) solution was prepared by dissolving 8 g of sodium chloride, 0.2 g of potassium chloride, 1.44 g of disodium phosphate, and 0.24 g of potassium dihydrogen phosphate in 1 L of distilled water. The pH of the PBS solution was adjusted to 7.4 using hydrogen chloride.
A suspension of C. albicans cells having 1 × 103 to 5 × 103 CFU/mL was prepared in the same manner as described in the above section “Evaluation of antifungal activity”. The cell suspension (10 mL) was dispensed into a 250 mL baffled flask with an additional 10 mL of RPMI 1640 medium, and cultured at 35°C for 48 h without shaking. Berberine (0.62 mM), magnolol (0.41 mM), and amphotericin B (6.49 μM) were prepared in PBS solution with 2% DMSO.
Berberine (80 mM), magnolol (75.09 mM), and amphotericin B (32.46 mM) were dissolved in DMSO, diluted 50 times with PBS first, and further diluted with PBS containing 2% DMSO to obtain a final treatment concentration.
The culture medium was diluted with RPMI 1640 medium so that the absorbance at 530 nm was 0.5, and aliquoted at 200 μL into a Corning® 96-well Clear Round Bottom Polystyrene Not Treated Microplate (product number: 3788; Corning). The plate was centrifuged at 840×g for 15 min at room temperature and the supernatant was removed. To the cell pellet, 50 μL of solution was added per sample, and PBS was added to make up the volume to 200 μL. In the positive and negative controls, 100 μL of solution was added to the cell pellet, and PBS was added to make up the volume to 200 μL. The plate was incubated for 24 h at 35°C and centrifuged at 840×g for 15 min. The amount of extracellularly released DNA in the supernatant was measured using absorbance at 260 nm.
Ergosterol was purchased from Tokyo Chemical Industry (catalog number: E0018). Ergosterol binding assay was performed to determine whether compounds directly bind to ergosterol in C. albicans cell membranes to clarify whether it causes cell membrane destruction. The ergosterol binding assay was performed following a slightly modified version of a previously reported procedure (Escalante et al., 2008; Leite et al., 2014a). Berberine, magnolol, amphotericin B, and ergosterol were dissolved in DMSO and diluted with RPMI 1640 medium. One hundred microliters of sample were dispensed into a Corning® 96-well Clear Round Bottom Polystyrene Not Treated Microplate, and C. albicans was inoculated and incubated in the same manner as described in the above section “Evaluation of antifungal activity”.
Quantitative analysis of ergosterol was used here as a method to measure the change in ergosterol production in C. albicans according to the sample treatment, following a partially modified version of a previously described method (Arthington-Skaggs et al., 1999).
Berberine, magnolol, and fluconazole were dissolved with RPMI 1640 medium with 2% DMSO. Five milliliters of sample solution were added to a 250 mL flask and RPMI 1640 medium with 2% DMSO was added to make the volume up to 10 mL. A cell suspension of C. albicans having 1 × 103 to 5 × 103 CFU/mL was prepared as described in the above section “Evaluation of antifungal activity”. Ten milliliters of prepared cell suspension were added to the flask and incubated at 35°C for 2 days without shaking. The cell pellet was collected by centrifugation at 680×g for 5 min, washed with PBS solution (pH 7.4), and weighed. Three milliliters of 25% alcoholic potassium hydroxide solution (25 g of potassium hydroxide, 35 mL of distilled water, 100% ethanol added to make up the volume to 100 mL) was added and mixed well. The cell suspension was transferred to a sterilized glass tube and incubated in a water bath at 85°C for 1 h. After adding 1 mL of distilled water and 3 mL of n-heptane to the cooled cell suspension, sterol was extracted by mixing for 3 min. The n-heptane layer was transferred to a clean glass tube and diluted with 100% ethanol, if necessary, to measure the absorbance at 281.5 nm and 230 nm. The ergosterol concentration was calculated in accordance with a previously reported method (Arthington-Skaggs et al., 1999) using these absorbance values.
Statistical analysis was performed using SPSS Statistics ver. 25 (IBM Korea, Seoul, Korea). The statistical significance of differences was tested by one-way analysis of variance (ANOVA) using Tukey’s method. Statistical analysis was performed by comparing the values of the negative control group to which no sample was added with those of the experimental groups treated with sample.
3. RESULTS and DISCUSSION
A previous study revealed the antifungal activities of the methanol extract of Phellodendri Cortex and Magnoliae Cortex against C. albicans (Yoon and Kim, 2021). To determine a suitable extraction method enabling these effects to be applied to the human body, three extraction methods, using ethanol, hot water, and supercritical carbon dioxide, were used to extract Phellodendri Cortex and Magnoliae Cortex. Although the growth of C. albicans was completely inhibited with all three solvent extracts of Magnoliae Cortex at 1 g/L, a supercritical carbon dioxide extract was selected for the subsequent experiment considering the highest concentration of 36% magnolol, the active compound of Magnoliae Cortex. In the case of Phellodendri Cortex, the hot water extraction method was selected based on cell growth inhibitory activity, extraction yield, and the highest concentration of 2.4% berberine, a candidate active compound in Phellodendri Cortex.
Zazharskyi et al. (2020) evaluated the antimicrobial activity of 38 plant extracts by a disk diffusion method. Among the tested extracts, 70% ethanol extract of the sprouts and leaves of Phellodendron amurense Rupr. showed antifungal activity against C. albicans via the formation of a clear region of 8.7 ± 0.7 mm around the disk. In addition, the screening of antifungal activity with a disk diffusion method using a library of 226 plant extracts in another study (Min et al., 1996) showed that the diethyl ether extract and methanol extract of Phellodendri Cortex exerted antifungal activity against C. albicans with a clear ring of 10 mm.
Chan et al. (2008) tested the antimicrobial activity of extracts made with eight plants including Magnoliae Cortex. In the disk diffusion method using 100 μg of extract, hot water extract or methanol extract did not show any antifungal effect on C. albicans, but ethanol extract of Magnoliae Cortex showed a clear ring 0.89 times the size of that of chlorhexidine used as an antifungal standard. It is suggested that the discrepancy between the results of this study and Chan’s results (Chan et al., 2008) on the antifungal activity of methanol extract on C. albicans is due to the difference in the amount of extract tested.
The antifungal activity against C. albicans according to extract concentration was measured using the hot water extract of Phellodendri Cortex (PC-WE) and the carbon dioxide supercritical extract of Magnoliae Cortex (MC-CDSE) (Fig. 1). The antifungal activity of PC-WE increased rapidly as the concentration of the extract increased, and showed antifungal activity of about 80% at 1.25 g/L. At concentrations above 1.25 g/L, the antifungal activity gradually increased with increasing concentration of the extract, and the antifungal activity was almost 100% at 5 g/L. MC-CDSE showed little antifungal activity up to 0.5 g/L, but about 90% antifungal activity at 1 g/L.
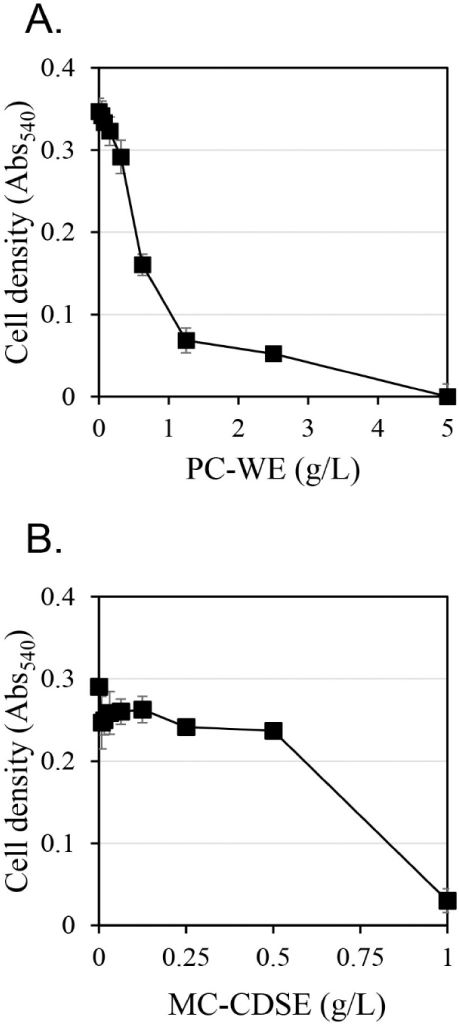
The synergistic antifungal activity against C. albicans upon mixing MC-CDSE and PC-WE was evaluated (Fig. 2). If the antifungal activity of mixtures was twice or more in both cases compared with the antifungal effect when each single extract was administered, it was judged that synergistic activity occurred. Synergistic antifungal activities were observed when the concentration of MC-CDSE was 0.59 g/L and the concentration of PC-WE was 0.55 g/L. MC-CDSE only had antifungal activity of 9.6% at 0.59 g/L, and PC-WE only had antifungal activity of 24% at 0.55 g/L, but the mixture of these two extracts at the same concentration showed an antifungal effect of 93.3%.
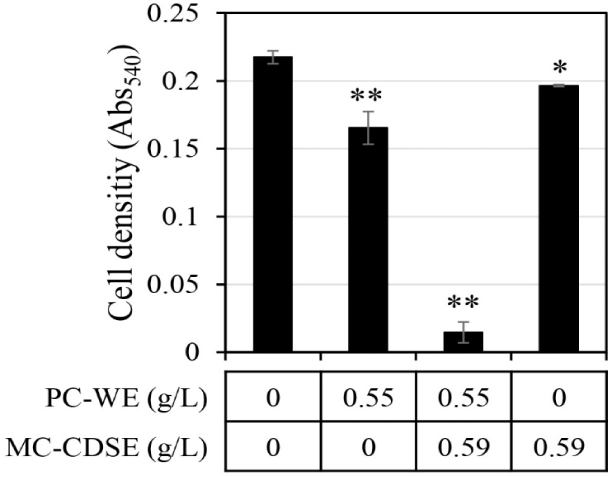
Alkaloid compounds in Phellodendri Cortex were reported to exhibit antifungal activities (Campos et al., 2018; Kim et al., 2018; Sun et al., 2019; Xie et al., 2020). The major compound among alkaloids in the extract of Phellodendri Cortex was shown to be berberine (Chan et al., 2007). Berberine is known to have antimicrobial (Čerňáková and Koš’álová, 2002; Freile et al., 2003), anti-inflammatory (Kuo et al., 2004), anticancer (Kumar et al., 2015), and antioxidant activities (Kumar et al., 2015). It has also been reported to exhibit antifungal activity against C. albicans by disrupting the cell membrane (Zhao et al., 2010; Zorić et al., 2017).
The level of berberine in the extracts of Phellodendri Cortex was analyzed using high-performance liquid chromatography. Berberine was found in hot water extract at 2.4% w/w but not in carbon dioxide supercritical extract of Phellodendri Cortex in this study. The level of berberine ranged between 0.515% w/w and 7.425% w/w depending on the batch when pulverized Phellodendri Cortex was extracted with methanol containing 1% HCl in a previous study (Zhu et al., 2011). Although the extraction solvent differed, the berberine concentration of PC-WE produced in this experiment fell within that previously reported concentration range (Zhu et al., 2011).
Magnolol is a polyphenolic compound that is mainly isolated from Magnoliae Cortex and has anti-inflammatory (Park et al., 2004a; Shen et al., 2018), antimicrobial (Park et al., 2004a), antioxidant (Parray et al., 2018), and anticancer activities (Cheng et al., 2016). Magnolol is also widely known to have antifungal activity, including against C. albicans, by destroying the cell membrane (Behbehani et al., 2017; Chen et al., 2019; Oufensou et al., 2019; Sun et al., 2015a; Zhou et al., 2017).
In the analysis of magnolol in the Magnoliae Cortex extract, it was hardly observed in the hot water extract, but the proportion of magnolol in the carbon dioxide supercritical extract was 36.1% w/w. The amount of Magnoliae Cortex used for MC-CDSE was 3,200 g, and the mass of MC-CDSE was 55 g. The solid yield of the extract was 1.72%. The amount of magnolol in MC-CDSE from 3,200 g of Magnoliae Cortex was 20 g in this study. Considering that the magnolol content in Magnoliae Cortex is 0.33%–0.75% w/w (Chen et al., 2006; Tsai and Chen, 1992), the 0.62% w/w magnolol extraction yield in this study was quite high. According to a study by Jiang et al. (2012), the concentration of magnolol in the methanol extract using powdered Magnoliae Cortex ranged from 0.005% w/w to 9.191% w/w. The magnolol concentration (36.1% w/w) of the MC-CDSE in this study was about 3.9 times higher than the maximum concentration (9.191% w/w) in a previous study (Jiang et al., 2012). Considering this extraction yield and the high magnolol content of the extract, it was suggested that the carbon dioxide supercritical extraction method was suitable for extracting magnolol for antifungal activity from Magnoliae Cortex.
Since berberine and magnolol had antifungal activity against C. albicans and contributed a significant part of the extracts of Phellodendri Cortex and Magnoliae Cortex, respectively, studying the synergistic antifungal efficacy of berberine and magnolol against C. albicans should provide a clue to understand the synergistic antifungal efficacy of Phellodendri Cortex and Magnoliae Cortex.
First, the antifungal efficacy of berberine and magnolol was quantitatively analyzed (Fig. 3). Berberine rapidly increased antifungal activity in proportion to the concentration up to 200 μM, showing around 80% growth inhibitory activity at 200 μM, but gradually increased its antifungal activity thereafter at concentrations higher than 200 μM, showing almost 100% growth inhibitory activity at 400 μM (▲ in Fig. 3B). In the case of magnolol, the antifungal activity increased in proportion to the concentration, and growth inhibition was almost 100% at 370 μM (▲ in Fig. 3C). Based on the antifungal activities of berberine and magnolol as shown in Fig. 3, the synergistic antifungal activity was evaluated at the concentrations of 160 μM for berberine and 100 μM for magnolol (Fig. 4). Although 160 μM berberine showed 24.0% growth inhibition and 100 μM magnolol showed 25.9% growth inhibition, their combination at the same concentrations inhibited C. albicans growth by 77.1%. The growth inhibitory activity by the combination of these two compounds increased about three times compared with the activity upon administering each compound alone, so growth was synergistically inhibited.
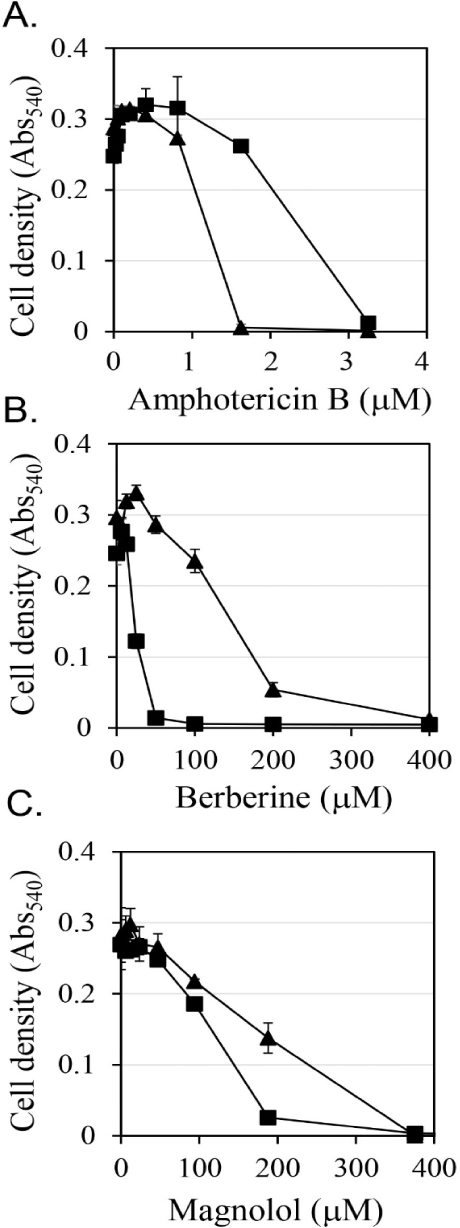
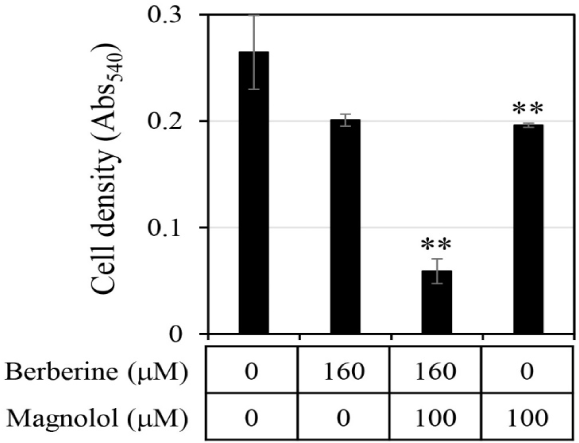
How much the antifungal activities of the mixture of berberine and magnolol contributed to the antifungal activities of the mixture of PC-WE and MC-CDSE was calculated. Since PC-WE contained 2.4% berberine, the concentration of 0.55 g/L PC-WE tested as shown in Fig. 2, which showed 24.0% growth inhibition, contained 39 μM berberine. As shown in Fig. 3B, berberine at 39 μM did not show any growth inhibitory activity. These results indicated that PC-WE contained other antifungal compounds such as palmatine in addition to berberine.
In the case of MC-CDSE, the content of magnolol was 36.1%, so 0.59 g/L MC-CDSE tested as shown in Fig. 2, which showed 9.6% growth inhibition, contained 800 μM magnolol. The growth of C. albicans was completely inhibited at a concentration of 800 μM (data not shown in Fig 3C). The low antifungal activity of MC-CDSE despite the high concentration of magnolol suggests the presence of compounds that interfere with the antifungal activity of magnolol in MC-CDSE.
We investigated how berberine and magnolol inhibited the growth of C. albicans synergistically on the basis of previously reported antifungal mechanisms, releasing chromosomal DNA by cell destruction (Hammer et al., 2004; Rajkowska et al., 2016), inhibiting the synthesis of ergosterol (Arthington-Skaggs et al., 1999; Khan et al., 2013), and interfering with the antifungal activity by exogenous ergosterol (Lee and Kim, 2016; Miron et al., 2014).
The amount of chromosomal DNA released from C. albicans was measured by treatment with 160 μM berberine and 100 μM magnolol (Fig. 5). When berberine and magnolol were administered alone, absorbance at 260 nm for measuring DNA was 0.165 and 0.070, respectively, which were levels 5.3 times and 2.3 times higher than the negative control. This confirmed that berberine and magnolol inhibited the growth of C. albicans by destroying cell integrity, as shown in previous studies (Sun et al., 2015b; Zorić et al., 2017). When berberine and magnolol were mixed, the absorbance at 260 nm was 0.43, which was 13.87 times higher than that of the negative control, and the degree of chromosomal DNA release was comparable to that of amphotericin B, an antifungal compound used as a positive control. The release of DNA by the mixture of berberine and magnolol was 2.63 times higher than that of berberine alone and 6.15 times higher than that of magnolol alone, suggesting the synergistic disruption of the integrity of C. albicans cells by mixing these two compounds.
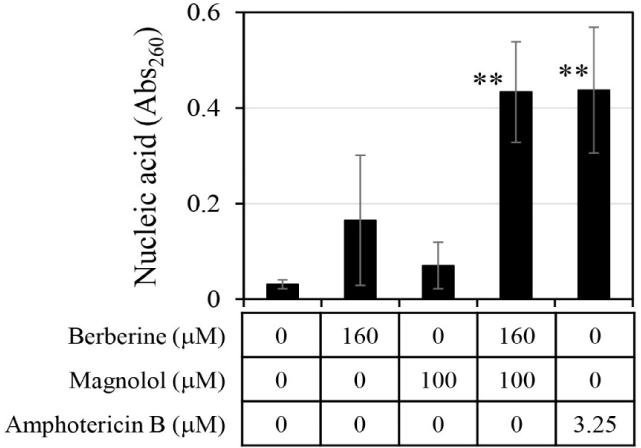
The ergosterol level in the C. albicans cells was analyzed after treatment with antifungal agents (Fig. 6). When berberine or magnolol was administered alone, the intracellular ergosterol levels were 70.8 ppm and 50.0 ppm, respectively, involving reductions by 25.0% and 47.0% compared with the negative control lacking any antifungal treatment. When berberine and magnolol were mixed, the level of ergosterol in cells was 35.6 ppm, constituting a decrease in ergosterol production by 62.3% compared with the negative control. However, there was still significant ergosterol production upon treatment with the mixture of berberine and magnolol, compared with the level upon fluconazole treatment as a positive control. Although the level of ergosterol in cells upon mixing berberine and magnolol decreased more than that upon treatment with a single compound, it was an additive not synergistic effect. These results suggest that berberine and magnolol affected the synthesis of ergosterol, but this was not a significant biological mechanism for the synergistic antifungal activity.
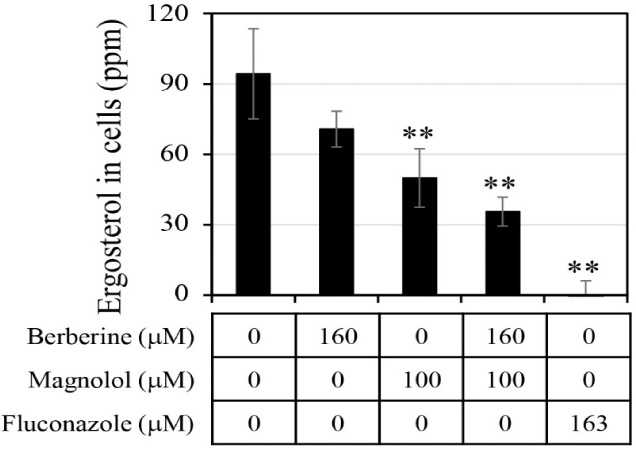
If antifungal compounds inhibit cell growth in a way that interferes with the biological function of ergosterol, then additional ergosterol from outside of cells may restore cell growth. The effects of the addition of ergosterol on the antifungal activities of amphotericin B, berberine, and magnolol were evaluated (Fig. 3). Amphotericin B is known as an antifungal agent that binds to ergosterol and destroys the function of the membranes of fungal cells (Yamamoto et al., 2019). It has been reported that the antifungal activity of amphotericin B was reduced by the addition of ergosterol (Leite et al., 2014b; Miron et al., 2014). In this study, when 0.25 mM ergosterol was added, it was observed that the antifungal activity of amphotericin B was reduced by about half (Fig. 3A). These results are consistent with the results of previous studies. In the case of berberine, the addition of 0.25 mM ergosterol increased the antifungal activity by more than double (Fig. 3B), which is the opposite of the decrease in antifungal activity due to the addition of ergosterol in amphotericin B treatment. Magnolol also showed a tendency to enhance the antifungal activity upon the addition of 0.25 mM ergosterol, but the degree of enhancement was smaller than that of berberine (Fig. 3C). The increase in antifungal activity against C. albicans upon the addition of ergosterol confirmed that the synergistic antifungal activity of the combination of berberine and magnolol was not associated with a decrease in the synthesis of ergosterol.
4. CONCLUSION
In this study, it was suggested that synergistic antifungal activity against C. albicans occurred through a combination of the hot water extract of Phellodendri Cortex and the carbon dioxide supercritical extract of Magnoliae Cortex. It was also suggested that berberine and magnolol were the active ingredients of each extract, respectively. Berberine and magnolol also showed a synergistic antifungal effect against C. albicans upon mixing.
The synergistic antifungal activity of the combination of berberine and magnolol was due to the destruction of the cell structure according to the findings on the release of chromosomal DNA, and was not correlated with inhibition of the biological function of ergosterol. Interestingly, the addition of extracellular ergosterol reduced the antifungal activity of amphotericin B, but in the case of berberine, the antifungal activity was increased by extracellular ergosterol. This suggested that the biological mechanism for the antifungal activity of berberine differed from that of amphotericin B, and it was suggested that the extracellular ergosterol facilitated cell destruction by berberine.