1. INTRODUCTION
Wood is the most in-demand construction material worldwide and widely used in various areas from civil engineering to landscaping, furniture and flooring materials. Its demand is on the rise (Lee and Lee, 2018). These uses take advantage of some of its main characteristics, such as good strength to weight relation, low thermal and acoustic conductivities, and renewability, among others (Acosta et al., 2020). Currently, in some temperate countries, the most important wood species present fast growing and, because of that, poor overall performance. In this context, several species belonging to the genus are required by the timber sector, due to their desirable characteristics, such as excellent productivity, quality and rapid growth in a short period of time (Hubbard et al., 2010). As a result, the Eucalyptus grandis wood becomes a promising alternative aiming to supply the market's demand for raw materials, considering that its wood has technological characteristics that enable its use for several purposes, such as: furniture industry, civil construction and cellulose. Brazil is the world's largest Eucalyptus producer, with a cultivated area of about 7.5 million hectares (IBGE, 2019) and an average stemwood mean annual increment (MAI) of around 36 m3 h-1 yr-1 (IBÁ, 2019). However, some of Brazilian eucalyptus, as the Eucalyptus grandis, has low dimensional stability, low mechanical properties, low thermal stability, and unattractive colour patterns (marked by light yellow shades). To overcome such disadvantages, several wood treatments have been developed and, among of them, thermal modifications stand out.
Many thermal treatments were already employed to increase the performance of fast-growing woods, especially pines and eucalypts. The temperature range (180 ºC and 225 ºC) applied in these treatments coincides with the thermal degradation of the main wood macromolecules (namely cellulose, hemicellulose, and lignin) and these controlled chemical changes are responsible for important already reported improvements in the wood performance (Herrera et al., 2018). The thermal treatment is also benign to the environment, since do not need of chemicals and a low amount of energy is demanded along the process (Delucis et al., 2014). Decreased dimensional stability, anatomy modification, improved colour pattern and stability, increased durability are some of the main findings for thermally treated woods (Kang et al., 2019; Kang et al., 2018; He et al., 2019).
Regarding the mechanical properties, for Poubel et al. (2013), the thermal treatments often induce to losses in specific gravity and, consequently, mechanical properties, which are mainly related to thermal degradation mechanisms in hemicelluloses. For instance, Gunduz et al. (2008) studied a pine wood (Pinus nigra) subjected to three different temperatures (120 °C, 150 °C and 180 °C) and reported losses in certain mechanical properties. Also, Korkut et al. (2008) treated the Pinus sylvestris wood under high temperatures and reported losses in certain mechanical properties. For example, in Brazil there is a pioneer in the treatment of wood with temperature, a TWBrazil ™, however the high loss of mechanical properties caused by the rectification limits the use of this material for wooden structures, making the application only yield to the cases where no mechanical effort is required. The present study aims to evaluate thermal, chemical, optical and hardness properties of the Eucalyptus grandis wood planted in Brazil thermally treated under three different temperatures.
2. MATERIALS and METHODS
Eucalyptus grandis which 22 years old trees were selected in a homogeneous forest from Tapes/Brazil. Seven prismatic samples with the dimensions of 20 mm × 20 mm × 80 mm (radial × tangential × longitudinal) were cut for each thermal treatment (including the control group), 28 wood samples in all. These samples were conditioned in a climatic chamber (at 20 ºC temperature and 65 % RH) until reach constant mass. The thermal treatments were carried out in a laboratorial oven under 160 °C, 200 °C and 240 °C, always for 2 h. The final samples were again conditioned in the mentioned climatic chamber until reaching equilibrium moisture content.
The weight percentage loss (WPL) and specific gravity (at equilibrium moisture content) were determined using a digital calliper (0,01 mm precision) and an analytical balance (0,001 g precision), following the (ASTM D 2017-05 2014), while the Janka hardness test was performed on tangential plane of the same samples, following the method described in (ASTM D 143-94 2014).
The chemical composition was evaluated by means of Fourier-transform infrared spectroscopy (FTIR). For this, powder samples were analysed with diffuse reflectance, in the 1800 cm-1 to 600 cm-1 range, using a spectrophotometer FT/IR-6000 producer by Jasco, Mary’s Court Easton, United States. Thermal stability was evaluated by thermogravimetric (TG) analysis, under nitrogen atmosphere at a heating rate of 10 ºC⋅min-1, using a thermal gravimetric analyser TGA 1000, producer by Navas Instruments, Conway, South Carolina, United States. For this test, were used a cubic sample (1 x 1 x 1 cm).
Colorimetric analyses were performed on the tangential plane of the samples, using a CR-400 Spectrophoto colorimeter produce by Konica Minolta Sensing Americas, Inc, 101 Williams Drive Ramsey, NJ 07446 United States. Adjusted for a D65 source light and an angle of observation of 2 °. This equipment reported brightness (L*), green-red coordinate (a*), blue-yellow coordinate (b*), chroma (C*) and hue angle (h), which are used to calculate the total colour variation (E), as described in previous studies (Romagnoli et al., 2013; Gallio et al., 2019). Some wood properties (WPL, density, hardness, and colorimetric properties) were subjected to analyses of variance and whenever the null hypothesis was rejected, the averages were compared using Fisher tests at 5 % of significance. The program utilized for the statistics analyses were the Statgraphics Technologies, Inc. Virginia, United States.
3. RESULTS and DISCUSSION
The pristine eucalypt presented the highest density of 0,548 g . cm-3, followed in a decreasing order by T160 ºC, T200 ºC and T240 ºC. Those results displayed in Fig. 1 show that the higher the treatment temperature, the higher the losses in both values for weight percentage loss (WPL) and hardness (σH). In this sense, compared the control group, the treatment at 240 ºC stood out with decreases of 16,16 % and 60,81 % for WPL and σH, respectively.
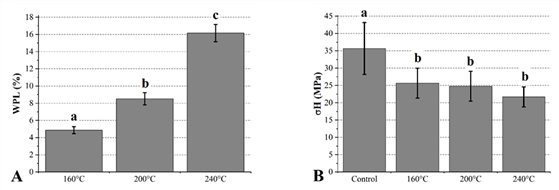
Cademartori et al. (2014) also reported this same direct trend for WPL and the treatment temperature, which was attributed to particular thermal degradation mechanisms related to all the wood macromolecules. Some authors reported close relations between mechanical properties and the treatment temperature for several wood species, as the increase to modulus of elasticity of 4,9% to Pyrus elaeagnifolia (Gunduz et al., 2009), 3,77% to Eucalyptus grandis (Modes et al., 2017) and 2% to Pterocarpus soyauxii (Corleto et al. 2020). As the temperature rises, degradation of the major components of wood occurs, such as hemicellulose, cellulose, lignin. (Kim et al., 2018, Park et al., 2020). They attributed the main effects related to the mechanical properties to the degradation in certain hemicelluloses, which must be related to those hardness results shown in Fig. 1.
Qualitative chemical changes were found using the infrared spectra shown in Fig. 2. Compared to the control group, the treated samples at 200 ºC and 240 ºC presented higher peaks at 1592 cm-1, 1508 cm-1and 1460 cm-1, which are attributed to different vibrations in certain chemical groups from lignin (C=O, C=C and C—H, respectively), as reported for the Pinus elliottii wood (Gallio et al., 2018).
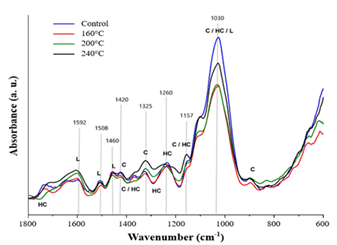
Also, compared to the control group, among the treatments, only at 240 ºC, there were higher peaks at 1030 cm-1, 1157 cm-1, and 1325 cm-1, which are attributed to stretching vibrations in syringyl units from lignin, C—O—C stretching vibrations in cellulose/hemicellulose and CH2 rock vibration in cellulose (Gallio et al., 2018; Zhang et al., 2019, Chang et al., 2019). Probably, this last finding occurred because 240 ºC is almost when the lignin starts its slow thermal degradation process and also occurs the degradation of crystalline segments from cellulose, as previously reported for the species Pinus elliottii, Eucalyptus grandis, Mezilaurus itauba and Dipteryx odorata (Poletto et al., 2012). This explains why only the T240 ºC yielded these chemical modifications. Attenuations in peaks at 1030 cm-1and 1260 cm-1were verified for all the treated samples. The peak at 1260 cm-1 is attributed a C—O stretching in xylan, while the peak at 1030 cm-1 is associated to C—H deformation in guaiacyl units from lignin (Gallio et al., 2018).
Regarding the thermal gravimetric curves (TG and DTG) shown in Fig. 3, they can be divided into three regions, wherein: region I is related to moisture loss (from 20 ºC to 100 ºC), region II is related to degradation in hemicellulose and cellulose (from 160 ºC to 480 ºC), and region III is related to degradation of cellulose and lignin (from 450 ºC to 600 ºC), as suggested by Pinto et al. (2016).
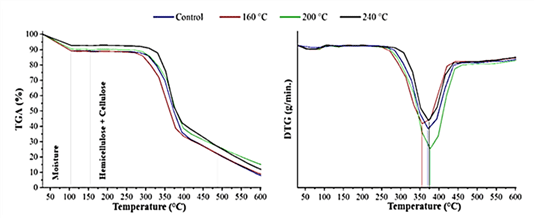
Those woods treated under 240 ºC stand out and presented the slowest thermal degradation rate in both the first and second regions, followed for those wood samples treated at 200 ºC. It is probably due to the high intensity of the previous degradation occurred in organic extractives and amorphous segments from hemicelluloses for those woods treated by the most severe treatments (200 °C and 240 °C).In the last region (region III governed by the lignin’s degradation), the slowest thermal degradation was that of those samples treated at 200 ºC, which is probably associated to plasticization and condensation mechanisms in lignin occurred for those samples treated at 240 ºC (Kim et al., 2014). Besides of that, the residual mass (above 600 ºC) is attributed to few inorganic compounds present in wood extractives and the lignin that partly becomes fixed carbon (Missio et al., 2014).
The most intense colour change was find for L* (Fig. 4), in which all treated samples presented decreased levels if compared to the control group, meaning the well-known darkening of the wood colour when exposed to thermal treatments (Moura and Brito 2011; Pincelli et al., 2012; Zanuncio et al., 2014). According to Moura et al. (2011), the wood darkening is related to the thermal degradation in hemicellulose and cellulose, which normally reflect visible light with high wavenumbers, leading to a perception of a light colour. Regarding the a* and b* levels, the treatments under 200 ºC and 240 ºC showed losses in reddish and yellowish pigments.
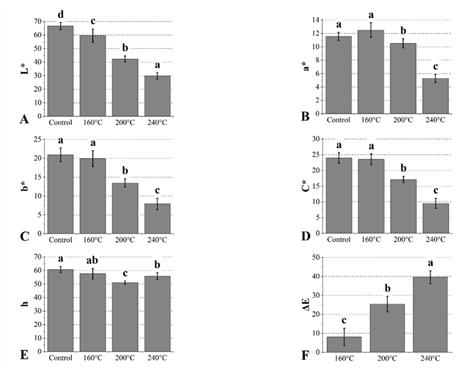
The losses in reddish pigments (a*) for thermally treated woods are often attributed to migration and volatilization of polar extractives (Zanúncio et al., 2014). Also, Delucis et al. (2014) reported a close relationship of the a* levels with phenolic compounds from the wood extractives. Regarding the b* levels, Romagnoli et al. (2013) reported an elucidative study on Tabebuia serratifolia wood and reported that the yellowish pigments belong to the wood are intimately related to the content of particular wood extractives, especially naphtha quinones, such as lapachol and dehydro-α-lapachone. Finally, compared to the control group, again those samples treated at 200 ºC and 240 ºC showed decreases for C* and h, which indicates a fading mechanism of the wood colour. This colour fading may also be attributed to below described reasons since these last two colorimetric parameters are directly dependent of L*, a* and b*. The ΔE levels confirm that the higher was the treatment intensity, the higher were the colour changes. In Priadi’s study with the wood of Antochephalus macrophyllus, found the darkening of the wood's color due to the heat treatment at 90 °C and 180 °C, with a greater increase in the ∆E variable at a temperature of 180 ° C (Priadi et al., 2020).
4. CONCLUSION
The evaluation of the proposed properties for the Eucalyptus grandis wood heat treated under three different temperatures proposed was carried out. In general, compared to the control group, the thermally treated woods presented the same compounds (namely cellulose, hemicellulose, lignin and extractives), as suggested by infrared spectra. The 200 ºC treatment stood out and can be indicated for the production of a product with greater added value. The treatment of Eucalyptus grandis wood under 240 ºC for 2 h proved to be an efficient way to produce a different wood product, with greater thermal stability and desired visual appearance, decreased specific gravity, decreased hardness, as well as darkened and faded colour patterns. These effects can be attributed to chemical changes in all the main wood.