1. INTRODUCTION
In industrial societies, most people spend more than 90% of their lives inside buildings. The indoor relative humidity (RH), an important factor influencing indoor environments, is the primary cause of condensation and biological pollution such as mold (Sundell, 1996). Many studies have shown that hygroscopic materials can moderate the indoor RH conditions (Ten Wolde, 1994; Plathner and Woloszyn, 2002; Simomson et al., 2002; 2004). Therefore, the importance of natural humidity in controlling the performance of interior materials has received considerable attention.
Wood is a hygroscopic material, owing to the -OH functional groups in the hydrophilic polymers such as celluloses and hemicelluloses, which form fixed water molecules by means of hydrogen bonds. This property allows the use of wood for interior finishing or furnishing materials that moderate the indoor humidity of buildings. However, the hygroscopic performance of wood leads to undesirable properties, such as swelling and shrinkage caused by various indoor RH conditions, which limit the use of wood in various applications.
The hygroscopicity of wood can be modified via physical or chemical treatments. For example, this ability can be reduced through exposure of the wood to high temperatures, thereby leading to a decrease in the hemicellulose content (Nuopponen, 2005; Byeon et al., 2008; Kocaefe et al., 2008; Lee and Lee, 2018). Furthermore, heat treatment increases the dimensional stability of wood, and increases the applicability of low-strength material to interior finishing or furnishing material (Edvardsen and Sandland, 1999; Chung et al., 2016; Park et al., 2016).
The hygroscopic properties of wood are affected by morphological differences and the surface roughness. The anatomical structures resulting from the heat treatment process were examined by means of scanning electron microscopy (SEM) (Huang et al., 2012; Priadi and Hiziroglu, 2012). The correlation between the surface roughness values and the drying speed of wood was determined via correlation analysis. The results revealed that the surface roughness has no effect on the drying speed of the wood veneer in radial cut (Gungor et al., 2010). In this study, the hygroscopic property of some interior finishing or furnishing wooden materials was investigated. Moreover, the effects of heat treatment on the surface chemistry, morphology, and roughness were also evaluated.
2. MATERIALS and METHODS
Specimens for the sorption test were prepared from Yellow poplar (Liriodendron tulipifera) logs (25–30 year-old trees) obtained from Seoul National University forest, Gyungki-do, Korea. Sawn 50mm (thickness) × 150mm (width) × 1,000mm (length) lumber was prepared for air-drying (AD), kiln-drying (KD), and high-temperature heat treatment (HT).
In addition, AD from the green condition (average initial moisture content 68%) was conducted for three months. Moreover, KD was carried out according to Forest Products Laboratory (FPL) drying schedule where an initial dry bulb temperature (DBT), initial RH, final DBT, and final RH of 60°C, 87%, 82°C, and 26%, respectively, were employed. The HT experiments were performed for 6 h at a DBT of 200°C.
To compare the hygroscopicity of the interior materials, Douglas-fir plywood and oriented strand board (OSB), which was commonly used for wooden wall materials, were employed.
Thirty pieces of yellow poplar (thickness 10mm, widths of 100mm × 100mm and 150mm × 150mm) for AD, KD, and HT were prepared for the experiment. Furthermore, 10 pieces of each OSB and plywood also were prepared with same size. Except for the surface areas (radial section) to be measured, all the sections were sealed by aluminum tape. Using a temperature and humidity chamber, the mass of wood at each equilibrium condition was measured during 24 h until the mass change was less than 0.01g. Afterward, to raise the RH, the adsorption process was performed for 12 h and the mass of the specimen was measured. The RH was then reduced and the desorption process was performed for 12 h. Subsequently, the mass of the specimen was measured (Table 1).
Humidity Conditions (Temp. 23°C) | Relative Humidity (%) | |
---|---|---|
Adsorption (12 h) | Desorption (12 h) | |
Low (Equilibrated at 30%RH) | 55 | 30 |
Medium (Equilibrated at 50%RH) | 75 | 50 |
High (Equilibrated at 70%RH) | 95 | 70 |
The moisture ad/desorption content and rates of moisture ad/desorption after 12 h at each state were calculated as follows:
ρa = Moisture adsorption content (kg/m2)
ρd = Moisture desorption content (kg/m2)
Gn = Moisture sorption rate at nth state (kg/(m2·h))
wo = Weight of final state at equilibrium (kg)
wa = Weight of final state at adsorption (kg, after 12h)
wd = Weight of final state at desorption (kg, after 12h)
wn = Weight at nth state (kg, after 12h)
wn-1 = Weight at n-1th state (kg, after 12h)
A = Surface area (m2)
t = Time (h)
Heat-treatment-induced changes in the functional groups of the wood were evaluated via FT-IR spectrum analysis of the wood. The specimens were prepared for FT-IR via ball milling (Pulverisette 23, Fritsch) using a 100 mesh. The corresponding spectra were recorded (resolution: 8cm-1) on a FT-IR-6100 (Jasco, Japan) spectrometer, operating over a spectral range of 650-4000 cm-1.
The microstructures of the untreated and heat-treated wood were characterized by means of field-emission scanning electron microscopy (FE-SEM; SUPRA 55VP, Carl Zeiss, Germany). A microtome was used to prepare 10 mm × 10 mm (widths) cross-sections (thickness: 100 μm) of the untreated and heat-treated wood.
Surface roughness analysis was performed, in accordance with ISO 4287 (1998), using spiny sweeping equipment (TR-200, Time High Tech.). The measurements were conducted at ten different points on each sample, along the vertical direction of the grains. The measurements were performed at a temperature of 20°C and a RH of 50%. The device, which was adjusted to 0.25-mm measurement steps and five measurement numbers (cut-off), was located between two lines with a rift of 5 mm. The surface roughness was measured by constructing a profile of the valleys and ridges on the surface by moving the sweeping needle (with a 5-μm-diameter diamond tip) across the surface of the sample (Budakçı et al., 2001). The surface section of the HT wood was ground by 180-grit and 1000-grit sandpaper and the adsorption rate of this section was then measured (temperature: 20°C, RH: 50%).
3. RESULTS and DISCUSSION
Fig. 1 shows the calculated moisture ad/desorption content associated with 12 h under each condition. In the case of relative humidity, the highest moisture ad/desorption content was obtained under high humidity conditions. This may be attributed to the sorption property of bound water on wood where capillary condensed water occurs under high relative humidity conditions. Similar moisture ad/desorption levels were observed for different surface area and thickness values.
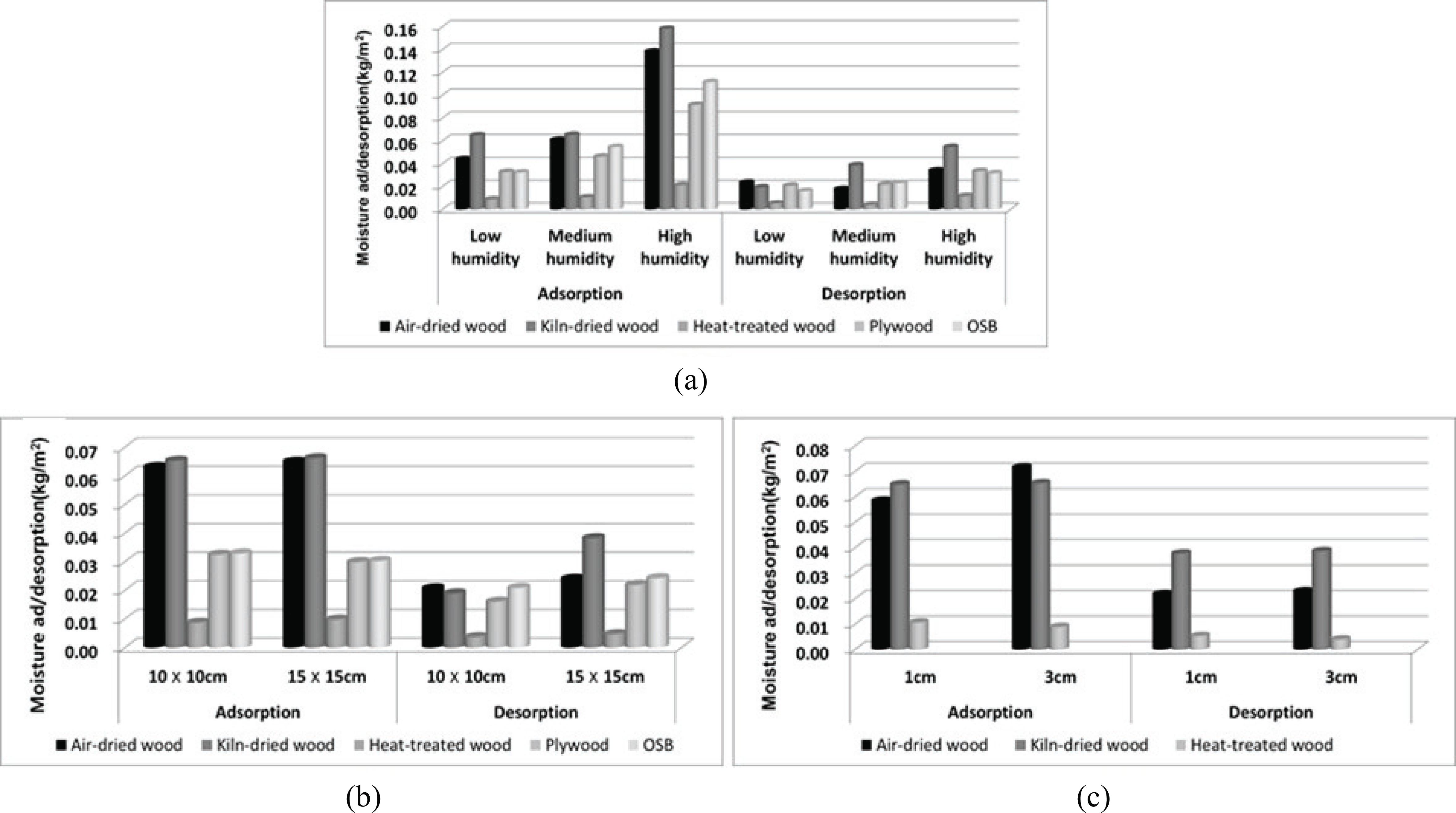
Due to insufficient time for equilibration of the moisture content between the surface layer and core layer of the specimen, the water molecule adsorbed in the surface layer moved rapidly to the core layer. Therefore, this adsorption is slightly affected by the surface area and thickness in finite time (24 h). These results corresponded to changing humidity conditions in the building during the day. The data is required for calculating the appropriate area and thickness of the wooden interior materials associated with a given size of space and changing humidity conditions in a building. These data were calculated based on 12-h cycles, but hourly measurements were required for determining the moisture ad/desorption rates. As Fig. 2 shows, the ad/desorption rate of each material was high initially, decreased sharply within 2 h, and then converged within 12 h.
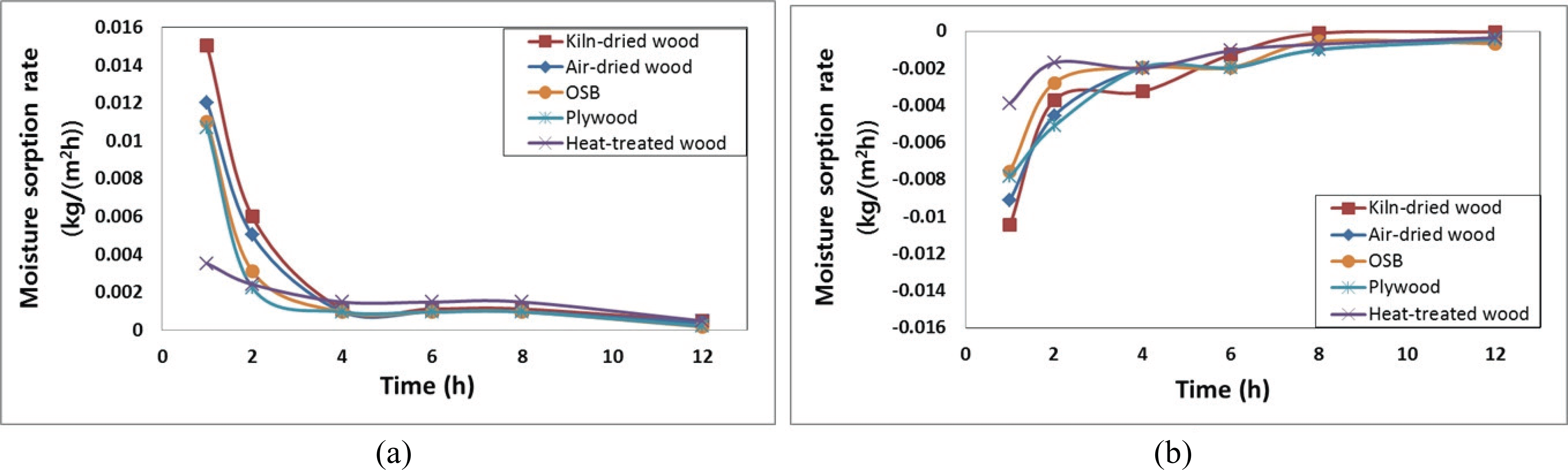
The rates of moisture ad/desorption indicate the degree of moisture ad/desorption of materials per unit surface area and time, and high values are indicative of an efficient response to rapid changes in humidity. The ad/desorption rates of the HT wood specimens were lower than those of the other wood specimens. This resulted from the hydrophobicity of the wood surface that renders adsorption of water molecules difficult. Moreover, the rates of moisture ad/desorption on the KD wood were higher than that of the AD wood. Vapor and moisture transfer on the former were easier than that of the latter because the number of pores in the wood increased with volatilization of wood extractives during KD wood. Plywood and OSB exhibited similar moisture ad/desorption rates, and the rapid gradient observed indicated that those approached the equilibrium state more rapidly than the rates of other materials (2 h). That is, compared with that of the other hygroscopic materials, the hydrophobicity of the wooden resin render the adsorption of water molecules difficult.
Fig. 3 shows FT-IR spectra obtained for the AD/KD/HT yellow poplar wood. The moisture adsorption point of the wood corresponded to the OH group from cellulose and hemicellulose, and was related to the hygroscopic property of the wood. For the wood subjected to the high-temperature treatment, the peaks occurring at 3400 cm-1 and 2900 cm-1 represent the OH group and the C-H group associated with symmetric stretching in aliphatic methyl, respectively. This indicates that combinations of the alkyl group, aromatic compound, and carboxylic acid were broken, owing to the dehydration resulting from the heat treatment.
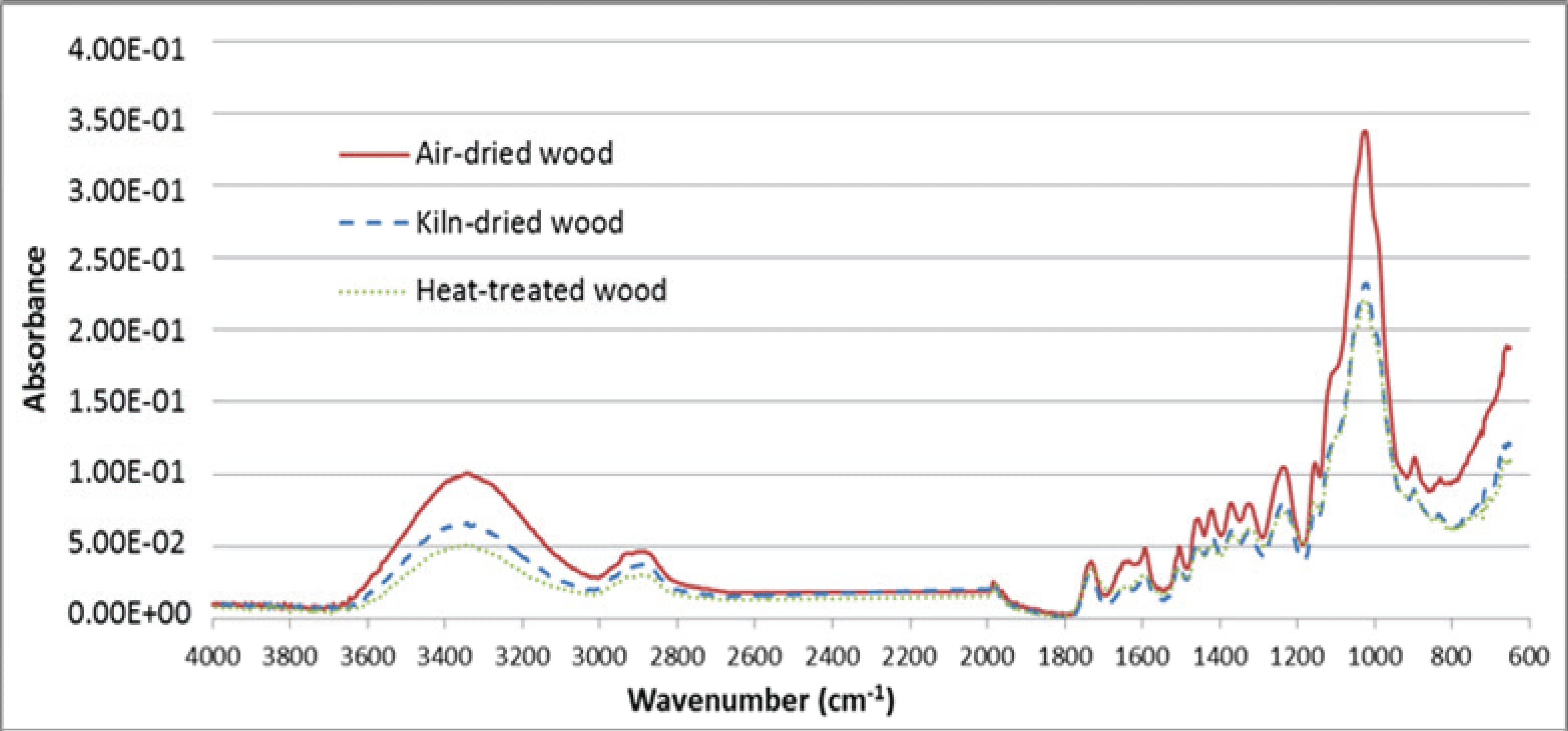
The band occurring at 1510 cm-1 is characteristic of the C=C stretching vibration in the aromatic skeletal of lignin. The heat treatment led to a reduction in the absorbance associated with this characteristic band of lignin (Nuopponen et al., 2003). In the KD wood, the peaks at 3400 cm-1 resulted from water-molecule loss and combination between the cellulose. This combination and loss occurred simultaneously with the formation of ester linkages during the heat treatment, thereby reducing the ratio of hemicellulose, which have many OH groups. However, the moisture ad/ desorption rates of the KD wood werehigher than those of the AD wood. Similarly, vapor and moisture transfer were easier because the number of micro-pores formed on the wood cell wall of the KD wood increased with drying-induced volatilization of the wood extractives.
The microstructural changes induced by heat treatment of the wood were evaluated via SEM. As Fig. 4 shows, the HT wood is characterized by many micro-cracks, shrinkage of the cell wall, and collapsed wood cells resulting from considerable water loss from the wood.
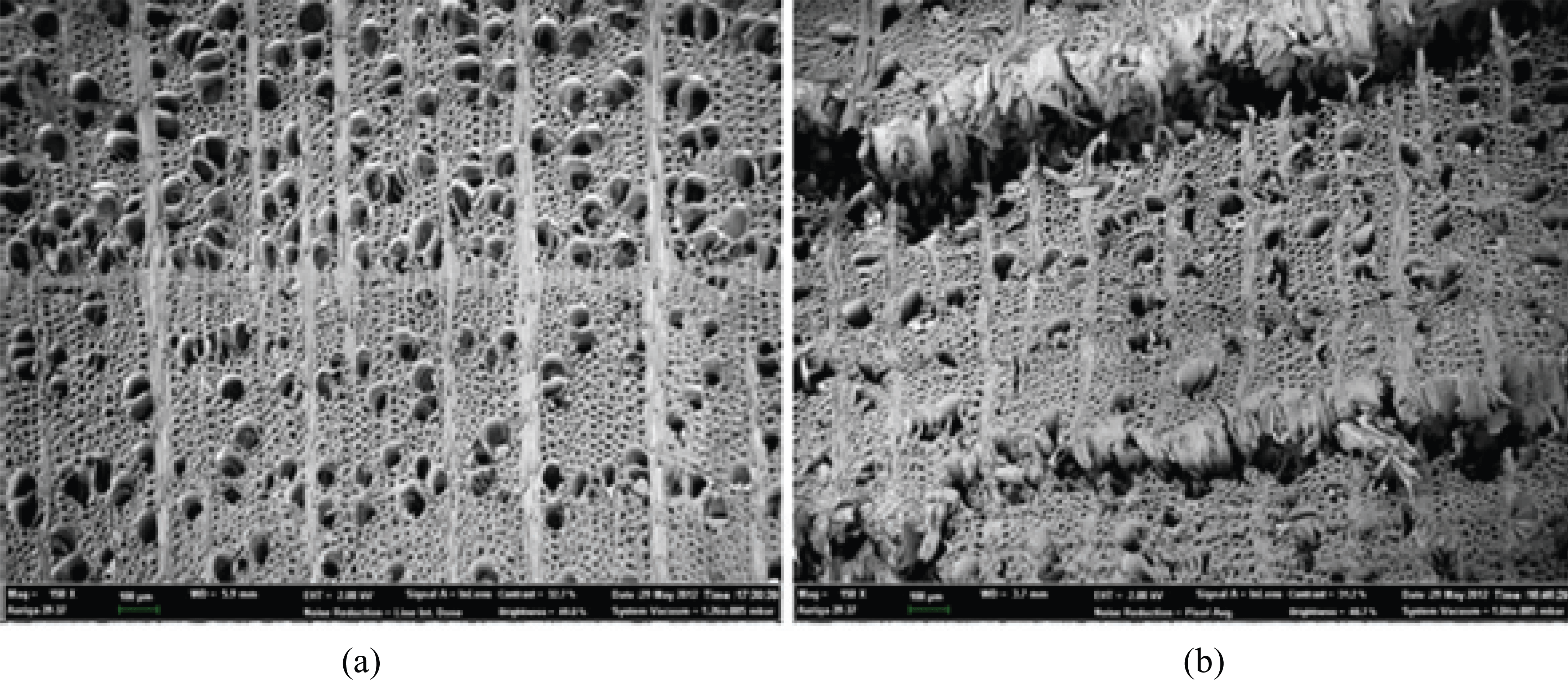
Mburu et al. reported that the differences resulted from the presence of extractives deposited in the resin channels, which evaporated after heat treatment (Mburu et al., 2007). Fig. 5 reveals the presence of small cracks on the middle lamella and a significant decrease in the cell wall width. The expansion of the micromovement path resulted in a decrease in the vapor diffusion resistance and, consequently, an increase in the speed of ad/desorption. However, the effects of structural changes by heat treatment were less severe than those of chemical changes such as hydrophobicity and moisture ad/desorption (Awoymi and Jones 2011).
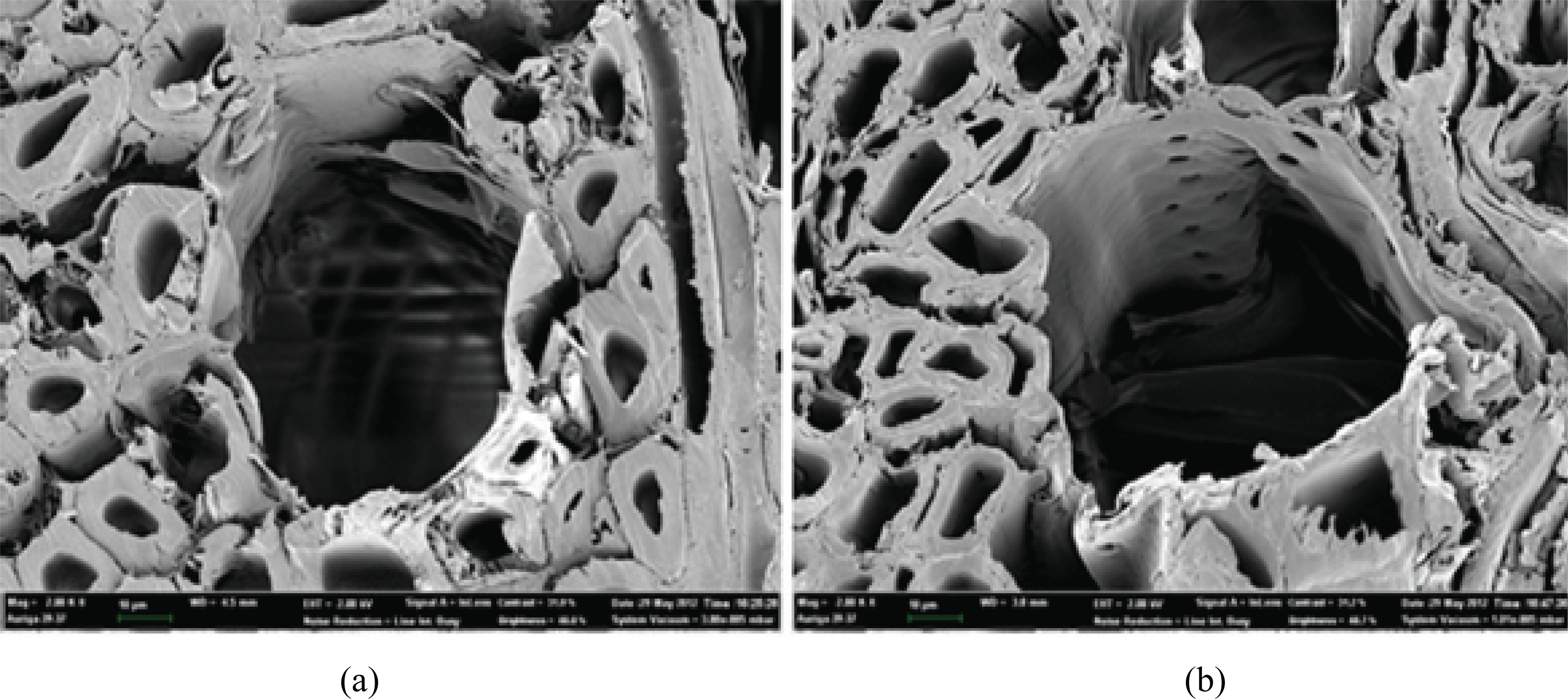
To elucidate the effect of heat treatment on hydrophobicity, the adsorption rate of HT wood was measured for samples prepared using 180-grit and 1000-grit sandpaper. The effect of heat treatment on the surface roughness of the wood was evaluated by considering four main roughness parameters which mean arithmetic deviation of the profile (Ra), root mean square roughness (Rq), maximum roughness (Rt), and mean peak-to-valley height (Rz).
For the HT wood ground by sandpaper, Ra: 8 μm, Rq: 12 μm at 180-grit and Ra: 4 μm, Rq: 5 μm at 1000-grit (Table 2).
Material | Roughness (µm) | |||
---|---|---|---|---|
Ra | Rq | Rt | Rz | |
180 grit | 8.09 | 11.83 | 45.85 | 31.95 |
1000 grit | 3.60 | 5.37 | 41.10 | 28.20 |
The moisture adsorption rate associated with the 180-grit sandpaper is higher than that of the 1000-grit paper, owing possibly to the fact that the high roughness increased the contact surface between the water molecules and the wood surface. The difference of initial rate of adsorption was 5 g/m2h in both cases, and the difference between the rates decreased gradually (Fig. 6). The surface roughness played a major role in the initial stage of adsorption, because water molecules were adsorbed on the surface of the wood. However, this role decreased due to gradual diffusion of water molecules into the wood cell wall.
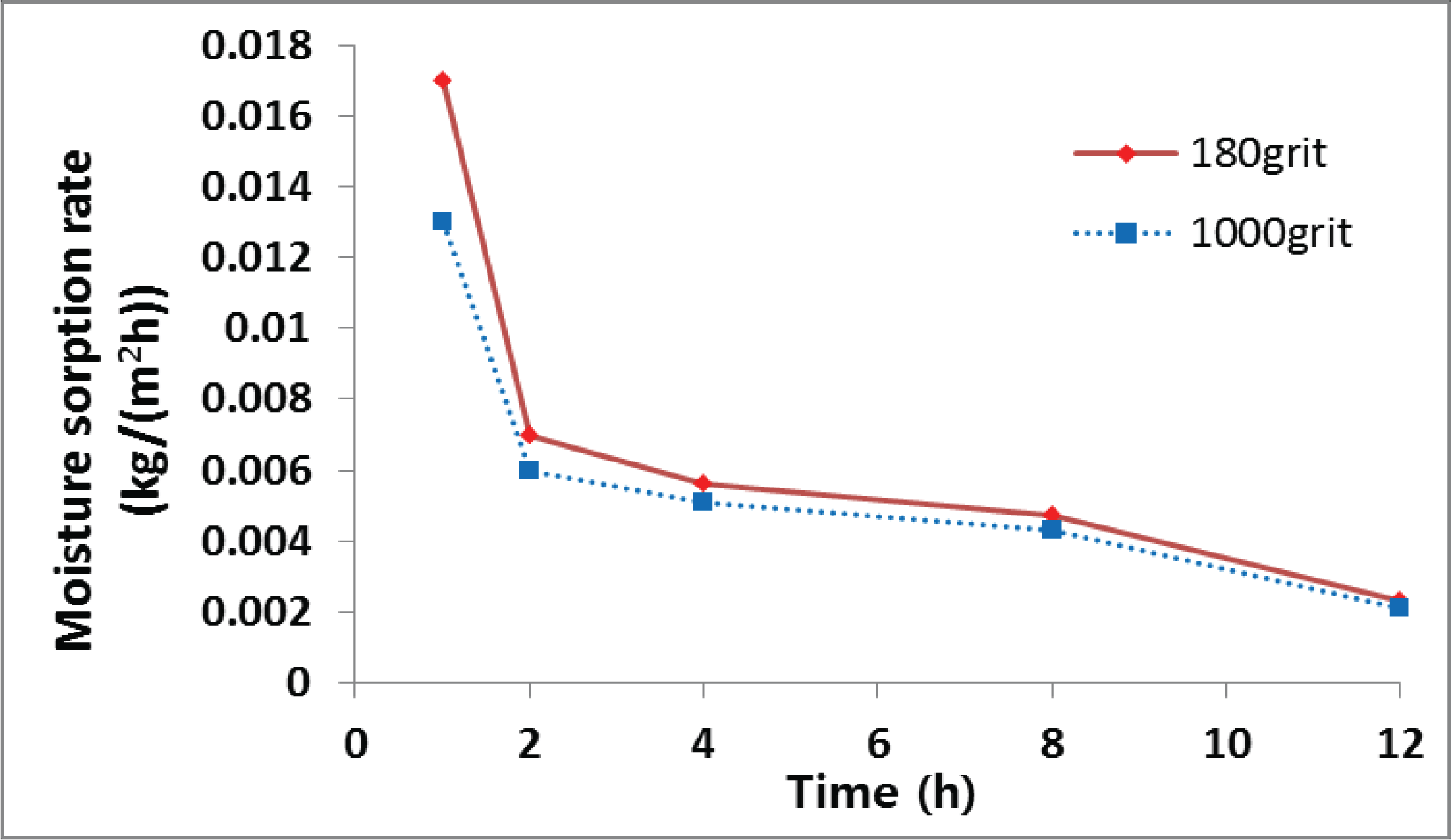
4. CONCLUSION
This study was aimed at changing the hygroscopic property of yellow poplar by means of heat treatment. The moisture ad/desorption content of the KD wood was higher than that of the AD wood. These results were attributed to changes in the microstructure, surface roughness, and functional groups. The roughness was considered a major factor in the initial ad/desorption. Moreover, the highest rate of ad/desorption was observed for the KD wood indicating the satisfactory hygroscopic performance of this wood, even with significant changes in the humidity condition. Heat treatment performed at appropriate temperatures will contribute to changed hygroscopic performance of wood. Although the hygroscopic property of the HT wood was inferior to that of the KD wood, this wood exhibited high dimensional stability and decay resistance for use in wooden products.
Therefore, this type of wood is used as interior material in spaces under continuous high RH conditions. In addition, a substance exhibiting outstanding hygroscopic property may have been injected into the extended numerous pores of the HT wood to change the hygroscopic performance. The results of this study provide basic data for quantifying the hygroscopic property of residential spaces on wooden houses.