1. INTRODUCTION
In Indonesia, the forestry sector has an essential role in many aspects. The Indonesian forestry sector had relied on wood industries especially plywood, sawn timber, and others from 1970 to 1990. At that time, Indonesia was well known as the biggest plywood producer and exporter. However, since the last two decades, wood industries were replaced by pulp and paper industries. According to Statistics Indonesia (BPS 2014a; 2014b; 2015; 2016; 2017), the Indonesian export volume of pulp and paper increased significantly from 4.8 to 6.0 million ton during the last five years, while the productivity of timber industries decreased.
Pulp and paper industries in Indonesia highly depend on wood from plantation forests in the last twenty years. The forest was established either by local people or corporation using fast-grown tree species, especially Mangium (Acacia mangium), Sengon (Falcataria moluccana), and Eucalyptus (Eucalyptus spp.). Though pulpwood from plantation forests increases every year, some species from the natural rainforest are still needed to improve the characteristic of pulp and paper produced. However, the suitability of the species has not been proven scientifically. Wood characteristics, especially in anatomical structure and fiber quality, have to be properly investigated to improve the quality of pulp and paper (Hidayat et al., 2017).
According to Soerianegara et al. (1993) and Mandang (2013), there are approximately 4000 potential wood species in Indonesian tropical rainforest, and only less than 10% has been utilized. There is a lot of wood species that can be selected and used for the candidate. Benuang (Octomeles sumatrana), Duabanga (Duabanga moluccana), Pisang Merah (Horsfieldia hellwigii), and Terap (Artocarpus odoratissimus) which are categorized as lesser-used wood species are a prospective source because of its abundance in natural forests. Understanding of these species' characteristics will lead to the evaluation of its potential in wood markets. Some researchers have done a similar project. They characterized some lesser-known and lesser-used wood species to promote the utilization (Poku et al., 2001; Olson, 2003; Ishengoma et al., 2004; Shupe et al., 2005; Grünewald et al., 2009; Junior et al., 2009; Ali, 2011; Ogunwusi, 2012). These characteristics, which are varied among species as well as within and among the trees, can be used to predict an appropriate processing and proper utilization of the wood (Barnett and Jeronimidis, 2003; Wahyudi, 2013; Hidayati et al., 2017; Park et al., 2017; Yang et al., 2017; Park et al., 2018; Purusatama et al., 2018). Hence, it is important to characterize the properties of lesser-used wood species to predict the appropriate processing technique and proper utilization.
This study aimed to characterize the four lesser-used wood species, especially their anatomical structures and fiber quality to evaluate its suitability in pulp and paper manufacturing. An evaluation was conducted by comparing the characteristics of experimental species with general raw materials in pulp and paper manufacturing (Mangium, Sengon, and Eucalyptus).
2. MATERIALS and METHODS
The trees are large to very large, dioecious evergreen, 45‒60 m tall, and about 90‒250 cm in diameter. The trunk is straight, cylindrical, branchless up to 30 m, and buttressed 3‒6 m high. The outer bark is thick up to 5 mm, greyish brown color, and has shallow streaks. The natural distribution of this species ranges from the Philippine, Sumatera, Kalimantan, Sulawesi, Moluccas, and New Guinea. In Indonesia, it grows on low altitude riverside land up to 1000 m asl. (Martawijaya et al., 2005; ITTO’s site; Ogata et al., 2008). According to Martawijaya et al. (2005), Ogata et al. (2008), and Susila (2010), Benuang belongs to the fast-growing and pioneer species.
The genus of Duabanga consists of two species, D. molucanna in Kalimantan, Java, Timor, the Philippine, Sulawesi, the Moluccas, and New Guinea; and D. grandiflora from the Himalayas to Southern China, Andaman Islands, and Malaya. The trees are medium to fairly large, 35‒45 m tall, and up to 100 cm in diameter. The trunk is straight, cylindrical, without buttress (ITTO’s site; Ogata et al., 2008). According to ITTO’s site, the trees growing in the forest along streams at a low altitude up to 1200 m asl. and frequently present in regrowth and riverside areas.
According to Krisdianto et al. (2013), and ITTO’s site, the trees are small to medium-large, 30 m tall, and about 60 cm in diameter. The trunk is straight, cylindrical, and occasionally has small buttress. The bark is smooth and greyish white. The natural distribution of this species is from the Malay Peninsula, Kalimantan, Sumatera, and Java to New Guinea. According to Ogata et al. (2008), the trees growing scattered in lowlands, lower montane, or rarely montane rain forests up to 1200 m asl.
The trees are medium to large and 35‒43 m tall. The trunk is straight, cylindrical, without buttress, and often about 65 cm in diameter. The bark is brown to greyish brown and dense woolly. The natural distribution of this species ranges from India to Southeast Asia and Western Pacific. In Indonesia the tree growing on lowland evergreen forest to 750 m asl. (Abdurrohim et al., 2004; ITTO’s site; Ogata et al., 2008).
The main materials were 5 cm thick wood discs of Benuang, Duabanga, Pisang Merah, and Terap from tropical rainforest in Kalimantan, Indonesia. Information on the experimental materials is presented in Table 1.
A small block of 2 cm (width) by 5 cm (thick) by 5 cm (length) was prepared from wood disc representing three locations, i.e. near the pith (1st segment), middle (2nd segment), and near the bark (3rd segment). Wood blocks were then divided again into four parts of 2 cm by 2 cm by 2 cm for each parameter studied (Fig. 1).
Macroscopic characteristics, namely wood color, odor, texture, grain, luster, and heartwood percentage, were directly observed using loupe of 15 times magnification. Microscopic characteristics observation was carried out through the thin microtome specimens by light microscope (Zeiss Axio Imager. A1m). The specimen of 25 μm was produced by sliding microtome using safranin as a dying agent. The IAWA Hardwood List of IAWA Committee (Wheeler et al., 1989) was used as a reference for anatomical characteristics observation.
Fiber dimensions, namely fiber length, fiber diameter, and lumen diameter, were measured through macerated specimens using the same light microscope equipped with a camera. Cell wall thickness was half of the difference between fiber diameter and lumen diameter. Maceration process was based on the modification of Franklin’s method (Rulliaty, 1994). A total of 50 individual fibers were measured for each segment. The derived values of fiber dimension namely Runkel ratio (RR), felting power (FP), Muhlsteph ratio (MR), flexibility ratio (FR), and coefficient of rigidity (CR) were calculated following the equations:
Where,
w = fiber wall thickness
L = fiber length
l = diameter lumen
d = diameter fiber
The derived values were then used to determine fiber quality class following Rachman and Siagian (1976), as described in Table 2.
3. RESULTS and DISCUSSION
Results showed that heartwood is yellowish-brown but quite easy to be differentiated from sapwood (Fig. 2A). Wood texture is medium and even, wood grain is straight to interlocked (Fig. 2A&B) while growth ring indistinct (Fig. 2C). The wood surface is dull and has no specific odor. From the measurement, it was found that the heartwood portion is 63.38%.
Results also showed, that wood is diffuse-porous and there is no distinct difference in pore size between earlywood and the latewood (Fig. 3A). Most vessels are solitary and in radial arrangement, partly multiple 2 cells (Fig 3A: arrows). Average lumen diameter is 182.29 (141.90–231.89) μm and vessel frequency is 5 (4–6) cells per mm2. The solitary vessel is rounded in transversal section. Perforation plate is simple, while inter-vessel pitting is alternate with a polygonal shape (Fig. 3D). Gum, tyloses, and other deposits are not present.
Fibers are septate and non-septate (Fig. 3D: arrows), the average length of 1565.61 (844.75–2561.5) μm, very thin-walled (2.93 μm), with simple to minutely bordered pits.
Axial parenchyma is rare, narrow to slightly wide and short; 1–4 cells width; and have >8 cells per strand. (Fig. 3A&B). Apotracheal axial parenchyma is diffuse, while paratracheal axial parenchyma is scanty (Fig. 3A: P1), and the sheath is narrow (Fig. 3A: P2).
Rays' width is 1 to 3 cells (Fig. 3C), average height is 738.10 (309.91–1414.47) μm, and have 5 (4–6) cells per mm. The Body ray consists of procumbent cells with 2-4 rows of the square marginal cells (Fig. 3B).
These findings, especially the vessel diameter and vessel frequency, are following Ogata et al. (2008), but not for fiber length, cell wall thickness, type of parenchyma, type of fiber, the existence of crystal and silica. The fiber in our study is shorter, thicker, and septated; the axial parenchyma is scanty and sheath narrow (theirs are vasicentric and aliform). Furthermore, silica was observed in our study, but not the crystal.
Compared to Martawijaya et al. (2005), the similarity is found in vessel distribution, vessel diameter, vessel frequency, fiber length, cell wall thickness, type of parenchyma, and ray width, while un-similarity is found in tyloses, ray height, and ray frequency. Tyloses were absent in our study, but the ray cell is taller and has a higher frequency.
The results showed that heartwood is pale yellowish-brown and challenging to differentiate from sapwood (Fig. 4A). Wood texture is moderate to coarse, wood grain is interlocked (Fig. 4A&B), while growth ring boundaries are indistinct (Fig. 4C). The wood surface is dull and have no specific odor.
The result showed that wood is diffuse-porous, and there is no clear distinction in pore size between earlywood to latewood (Fig. 5A). Most of the vessels are solitary, but sometimes the radial multiple 2 cells and clustering up to 3 cells (Fig. 5A: CL). The average diameter of the lumen was 279.51 (196.55–383.51) μm and 3 (2–4) cells per mm2. The solitary vessel is rounded in transversal section. Perforation plate is simple, while inter-vessel pitting is alternate with a polygonal shape (Fig. 5C: arrows). Gum, tyloses, and other deposits are not present.
Fibers are septate and non-septate (Fig. 5D: arrows), the average length of 1599.39 (763.00–2670.50) μm, very thin-walled (3.47 μm) with simple to minutely bordered pits.
Axial parenchyma is wide and long (Fig. 5B&F) and mostly more than three cells in width and eight cells per strand. The apotracheal axial parenchyma is diffuse, while paratracheal axial parenchyma is vasicentric either sheath broad (Fig. 5A: SB), sheath narrow (Fig. 5A: SN), complete circular to oval sheath (Fig. 5B: PK) or incomplete (Fig. 5B: PA), as well as confluent (Fig. 5B: PK).
Rays exclusively uniseriate (Fig. 5D), the average length of 210.81 (79.03–336.29) μm, and 5 (4–6) cells per mm. Rays composition varies, square cells with 1–2 rows of upright marginal cells (Fig. 5E: J1); procumbent cells with 1–2 rows of upright marginal cells (Fig. 5E: J2); and procumbent, square, and upright cells mixed throughout the ray (Fig. 5F).
Furthermore, Duabanga wood, especially xylem area near to the bark, has loads of crystals in ray and axial parenchyma. In one chamber, there is one or more crystal. Prismatic crystals (Fig. 5G: KP), small cubic crystals (Fig. 5G: KK), and crystal sand (Fig. 5G: KPa) are found in axial parenchyma; while prismatic crystals (Fig. 5H: KP) and small cubic crystals (Fig. 5H: KK) are found in ray parenchyma. Silica was also found in ray parenchyma (Fig. 5H: SI).
These findings especially vessel distribution, vessel frequency, vessel diameter, ray wide, fiber length, type of axial parenchyma, and the existence of crystal are in accordance with Ogata et al. (2008), but not for the cell wall thickness, ray height, type of fiber, and existence of tyloses and silica. When compare to Ogata et al. (2008), the cell wall of Duabanga wood is thicker, but the ray is shorter. Furthermore, septate fiber and silica exist, but tyloses absent.
The results showed that heartwood is reddish-brown but slightly challenging to distinguish from the sapwood (Fig. 6A). The wood texture is medium and even, wood grain is straight (Fig. 6B), while growth ring boundaries slightly distinct to indistinct (Fig. 6C). The wood surface is slightly luster and without specific odor.
Results showed that wood is diffuse-porous and there is no significant difference in pore size between earlywood to the latewood (Fig. 7A). Most vessels are radial multiples of 2‒4 cells, partly solitary and clustering up to 3 cells (Fig. 7A: CL). The average lumen diameter is 152.80 (72–219.60) μm, and 5 (4–7) cells per mm2. The solitary vessel is round in the transverse section. Perforation plate is simple, while inter-vessel pitting is alternate with a polygonal shape (Fig. 7C: arrows). Gum, tyloses, and other deposits are not present.
Fibers are non-septate, the average length of 1691.86 (1062.75–2507) μm, very thin-walled (3.46 μm) with simple to minutely bordered pits.
Axial parenchyma is narrow to slightly wide and long (Fig. 7A&E), consisted of 2–5 cells width and more than eight cells per strand. Apotracheal axial parenchyma is diffuse-in-aggregates, which is grouped into short discontinuous tangential line (Fig. 7A: DA). Paratracheal axial parenchyma is scanty (Fig. 7B: P1) and sheath narrow (Fig. 7A: P2). Banded marginal parenchyma (Fig. 7A: DA) was also detected both in narrow (1–3 cells) (Fig. 7A: BP1) and wide bands (>3 cells) (Fig. 7A: BP2).
Rays wide is 1 to 3 cells (Fig. 7D), the average height of 449.89 (140.63–862.12) μm, and have 9 (6–12) cells per mm. Body ray consists of procumbent cells with 1 to 2 rows of square marginal cells (Fig. 7E&F). The diffuse silica bodies present in ray cells (Fig. 7B&F), while oil and/or mucilage cells associated with ray parenchyma (Fig. 7C, SM).
Compared to Ogata et al. (2008), the similarity was found on the fiber type (septate), axial parenchyma type, without crystal, and the occurrence of oil cell. Un-similarities are found in vessel distribution (clustering is observed in our study), fiber length (ours is shorter), and the existence of silica (was not observed by them). Compared to Abdurrohim et al. (2004), the similarity was found in vessel distribution, vessel frequency, type of axial parenchyma, cell wall thickness, without crystal, and also tyloses. The unsimilarity was found in vessel diameter (our vessel is bigger), fiber length (ours is longer), ray width (theirs is wider), ray height (theirs is taller), ray frequency (ours is greater), and the occurrence of oil cell and silica (were not observed in their study).
The result showed that heartwood is brown to reddish-brown and easy to differentiate from the sapwood (Fig. 8A). Wood texture is medium and even, the wood grain is interlocked (Fig. 8B), while the growth of ring boundaries are indistinct (Fig. 8C). The wood surface is slightly luster and has a distinctive odor. The measurement showed that the heartwood portion is 46.29%.
Results also showed, that wood is diffuse-porous and there is no distinct difference in pore size between earlywood and the latewood. Vessels are mostly solitary but radial multiples of 2–4 cells are sometimes present (Fig. 9A). Average lumen diameter is 156.22 (61.06–261.78) μm and 4 (2–8) cells per mm2. The solitary vessel is rounded in transversal section. Perforation plate is simple, while inter-vessel pitting is alternate with a polygonal shape (Fig. 9B: arrows). Gum, tyloses, and other deposits are present in the heartwood vessels (Fig. 9C: arrows).
Fibers are septate and non-septate (Fig. 9D: arrows), average length of 1709.66 (1199–2452.5) μm, very thin-walled (3.55 μm), and with simple to minutely bordered pits.
Axial parenchyma is wide and long (Fig. 9A&E), mostly more than three cells in width and eight cells per strand. Apotracheal axial parenchyma is diffuse, while paratracheal axial parenchyma is aliform (Fig. 9A: P1), and confluent (Fig. 9A: P29A: P2).
Rays exclusively uniseriate (Fig. 9F), sometimes 1 to 3 cells width, the average height of 423 (210–854.56) μm, and 5 (4–6) cells per mm. Body ray consists of procumbent cells with one row of square marginal cells. Silica bodies present in ray cells (Fig. 9G).
These findings have similarity and un-similarity with the previous study (Abdurrohim et al., 2004; Ogata et al., 2008). Compared to Ogata et al. (2008), the similarity was found in fiber length and fiber wall thickness; while the un-similarities are found in pore frequency (our findings is greater), type of axial parenchyma (vasicentric was not observed in our study), and type of fiber (septate fibers were not found by them). Compared to Abdurrohim et al. (2004), the similarity was found in vessel distribution, vessel frequency, type of axial parenchyma, cell wall thickness, and the presence of septate fibers; while the unsimilarity was found in vessel diameter (ours is smaller), fiber length (ours is longer), type of ray parenchyma (uniseriate in our sample, multi seriates in them), existence of crystal (was not observed in ours), and existence of silica (was observed in our study but was not observed by them).
The fiber length of the four lesser-used species studied and their derived values, are tabulated in Table 3. It was confirmed that wood fiber of all species was classified into Quality Class of II. It means that the resulting pulp and paper would have good quality, especially in tensile, folding, and tear strengths. According to Rahman and Siagian (1976), the fiber of Quality Class II is easily beaten, and fiber bonding is particularly strong.
Even though in general they were categorized in the same quality class, however, some variation in the strength of pulp and paper and fiber length among the species also exists. In each wood species, fiber length tended to increase from pith (1st segment) to the bark (3rd segment) (Fig. 10). The latter phenomenon is correlated with the presence of juvenile wood (Tsuchiya and Furukawa, 2009; Tanabe et al., 2016).
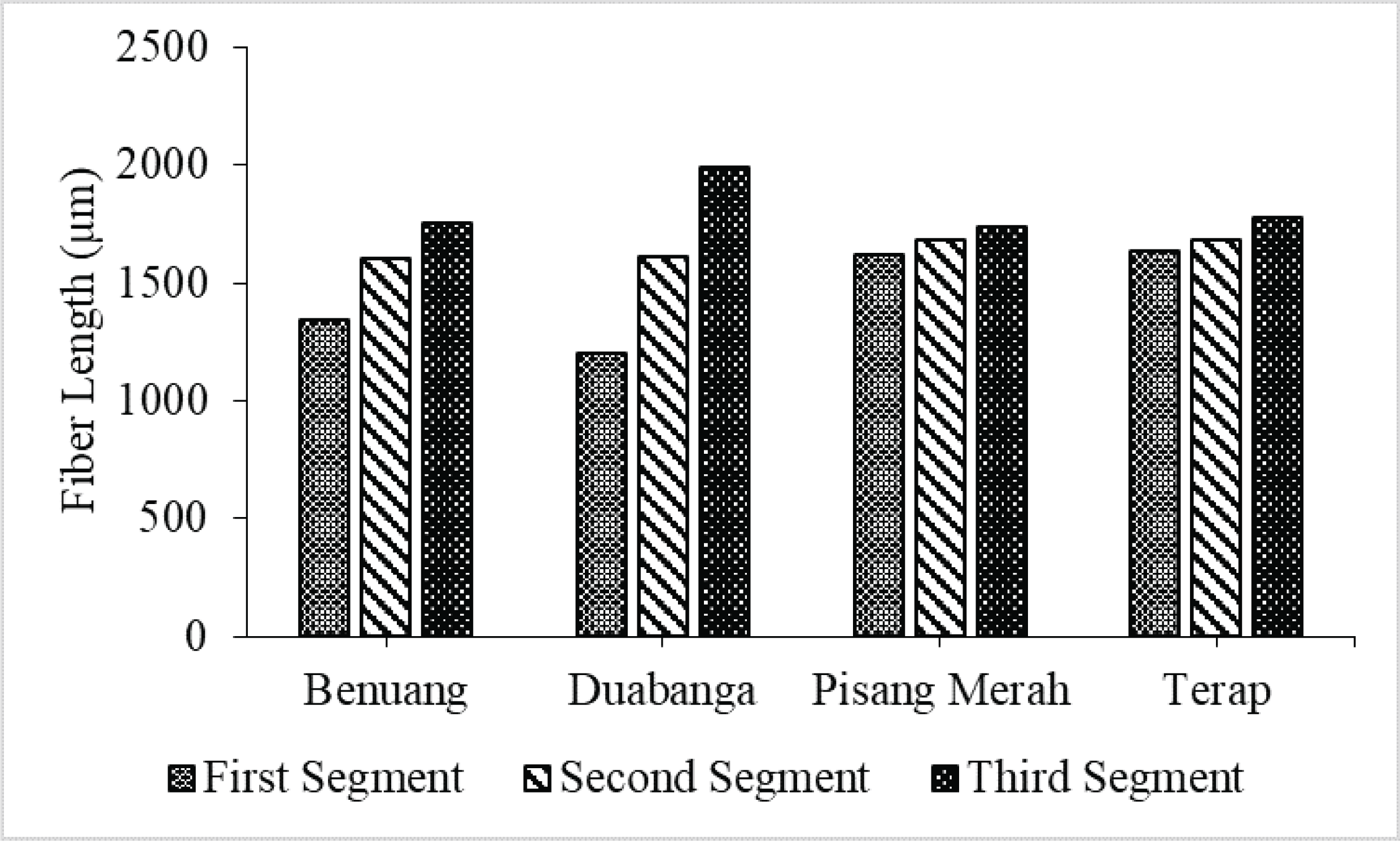
Variation in the strength of pulp and paper in this study is more correlated to the derived value of the fibers since fiber length of the four wood species is almost similar (1560 to 1700 μm). Table 3 shown that Benuang wood has the lowest Runkel Ratio (RR), Terap wood has the highest Felting Power (FP), while Duabanga wood has the lowest Muhlstep Ratio (MR). All fibers have a similar Coefficient of Rigidity (CR) and Flexibility Ratio (FR). CR has a positive correlation with pulp yield and density, while FR has correlations: positive with tensile strength, folding resistance, and density; negative with the porosity of pulp and paper (Adi et al., 2014; Supartini and Dewi, 2010; Supartini et al., 2013).
According to Panshin and de Zeeuw (1980) and Adi et al. (2014), the lowest RR resulted in the highest tensile strength. Hence, pulp and paper made from Benuang wood will have the highest tensile strength. Felting power or slenderness ratio has a positive correlation with tear strength (Ververis et al., 2012). Hence, pulp and paper made from Terap wood will have the highest tear strength. A higher value of MR meant lower bonding and contact area between fibers. It means that pulp and paper with higher MR value made from Pisang Merah wood are not resistant to tear. With the lowest in MR value, therefore, pulp and paper made by Duabanga wood will have the highest tear and tensile strength.
Important quantitative of anatomical features and fiber dimensions of these four wood species are tabulated in Table 4. Data is then compared to the conventional woods namely Mangium (A. mangium), Sengon (F. moluccana), and Eucalyptus (Eucalyptus spp.) These are common raw material in pulp and paper manufacturing.
It showed that vessel diameter of Pisang Merah and Terap wood is smaller than the Mangium, Sengon, and Eucalyptus, while Benuang and Duabanga are equal to the three conventional pulpwood. For the vessel frequency, Duabanga wood has similar frequency to Sengon wood, while Benuang, Pisang Merah, and Terap similar to Mangium and Eucalyptus.
It also showed that Benuang wood has the tallest ray parenchyma compared the others, except for Eucalyptus. Ray parenchyma in Duabanga and Mangium woods is similar and categorized as the shortest.
In contrast to Mangium, Sengon, and Eucalyptus woods, the fiber length of the four wood species studied is longer. However, their cell wall is thicker. The latter phenomenon is a valid judgment to support the idea that pulp and paper from the fiber of Mangium, Sengon, and Eucalyptus woods are better than the four wood species’ studied. According to Rachman and Siagian (1976), fibers of Mangium, Sengon, and Eucalyptus woods belong to Quality Class of I.
4. CONCLUSION
Fiber length and fiber quality class of Benuang, Duabanga, Pisang Merah, and Terap wood are similar, but their anatomical structures varied. The similarity in anatomical structures are found in growth ring, porosity, vessel outline, ray width, and deposits; while un-similarity are seen on vessel diameter size, vessel grouping, vessel frequency, the presence of tyloses, type of axial parenchyma, and ray height.
These lesser-used wood species are potential to be utilized as raw material for pulp and paper manufacturing. Their fibers belong to the Quality Class of II. Tensile, folding, and tear strengths of pulp and paper produced from all species studied are good. To promote their utilization, silviculture aspect of these four wood species has to be well understood and developed.