1. INTRODUCTION
Diabetes is one of chronic diseases that threaten human health along with obesity and increasing every year. In particular, that is a metabolic disease which insulin secretion is not controlled and blood glucose is not regulated. It is divided into type I diabetes, type II diabetes, and gestational diabetes. Type I is insulin-dependent diabetes, which is unable to produce insulin, and is unable to produce insulin naturally β-cells due to autoimmune disorders. Type II is caused by insulin resistance that cells do not respond to normal insulin action and is called non-insulin dependent (Eizirik and Mandrup-Poulsen, 2001; Miriam et al., 2005; choi et al., 2010). Currently diabetic patients have abnormally high level of glucose and insulin in the post prandial blood. However, they use various hypoglycemic agents to keep the blood glucose level within the normal range rather than the fundamental treatment. Due to the various side effects of synthetic hypoglycemic agents, recently researches on herbal medicines has been actively conducted (Heo et al., 2009; Standl and Schnell, 2012).
Free radicals and active oxygen are known to be detrimental to human health by damaging lipids, proteins, and nucleic acids, by causing oxidative stress, and by leading to aging and many geriatric diseases. Antioxidants remove free radicals through electron donation function (Aviram, 2000; Nono and Parola, 2008; Jung, et al., 2017; Jeong, et al., 2017; Kim, et al., 2017). Antioxidant activity is a very important part of antidiabetic efficacy because the oxidative stress is cause insulin secretion disorder by increasing β-cell damage in type II diabetes (Evans et al., 2002; Kim and Son, 2006).
Korean red pine (Pinus densiflora) belongs to the Pinaceae family and is widely distributed in Korea and in the other areas of East Asia (Kim, 1996). Needles, cones, bark, and pollen of Korean red pine have been used for medicine and safe food raw materials (Kim et al., 2012). Although many studies on the chemical components and biological activities have been reported on the extracts of Korean red pine leaves and branches, but the researches on the bark extracts have still plenty of room to be conducted. Also the antioxidative and antidiabetic activity on the active components of the pine bark have not been studied yet.
Therefore, this study was carried out to investigate the chemical constituents of the extracts of Korean red pine inner bark and to confirm the potential for functional application by the evaluation of antidiabetic activity of the extracts.
2. MATERIALS and METHODS
Korean red pine inner bark (2.11 kg), collected at the experimental forest of Kangwon National University, was air-dried in the laboratory for about two weeks, ground to fine powder, extracted three times with 70 % aqueous ethanol at room temperature, and then concentrated under vacuum pressure. The concentrated crude was sequentially fractionated with n-hexane, chloroform (CHCl3), ethyl acetate (EtOAc) and H2O using a separatory funnel (Table 1). A portion of EtOAc soluble (5 g) of those fractions was repeatedly chromatographed on a Sephadex LH-20 column using various kinds of eluting solvents, several aqueous MeOH-H2O, MeOH-CHCl3, and EtOH-hexane, to afford 5 isolated compounds. The structures of isolated compounds were characterized with NMR data using methanol-d4(CD3OD) NMR solvent and a Bruker Avance DPX 600 MHz spectroscopy at the central laboratory of Kangwon National University and then elucidated by NMR analysis and by comparison with the authentic literature data (Shinn et al., 2019; Foo and Karchesy, 1989; Harbone and Mabry, 1982; Ham and Bae, 1995; Ahn et al., 1996).
Then the crude, EtOAc soluble, H2O soluble, and the isolated compounds were treated to evaluate the antidiabetic activities. Each of the samples was dissolved in dimethy sulfoxide (DMSO, Sigma-Aldrich Co.) to prepare a 100 mg/mL stock solution and then added into the cell culture medium according to the preset treating concentration for evaluation of antidiabetic activities.
HIT-T15 hamster pancreatic β-cells were purchased from the Korean Cell Bank (Seoul, Korea). HIT-T15 cells were cultured in Ham’s F12K medium supplemented with 100 mL/L horse serum, 25 mL/L fetal bovine serum (FBS), 100,000 U/L penicillin, and 100 mg/L streptomycin at 37°C in a humidified atmosphere of 5% CO2. When cells were 80% confluent, they were subcultured using 0.25% trypsin-2.65 mM EDTA, and the medium was changed every 2 days.
Cell viability in HIT-T15 cells were estimated by the MTT assay (Denizot and Lang, 1986). The cytotoxicities of Korean red pine bark extracts on HIT-T15 cells were evaluated by MTT assay using the concentration of 0, 1, 5, 10, 25, 50 and 100 μg/ml. HIT-T15 cells were plated at a density of 2 × 104 cells/well in a 96-well plate and incubated for 24 h. After incubation for 24 h, cells were treated with various concentrations of test samples for 24 h. At the end of the treatment period, the media were removed and 1 mg/mL 3-(4,5-dimethyl thiazol-2-yl)-2,5-diphenyl tetrazolium bromide (MTT, Amresco, Solon, OH, USA) solution was added, and the cells were incubated at 37°C for 2 h. After incubation for 2 h, MTT solution was removed, 0.1 mL of isoporpanol was added to dissolve formazan crystals, and the absorbance was measured at 570 nm with a microplate reader (Molecular Devices, Sunnyvale, CA, USA).
HIT-T15 cells were plated at a density of 5 × 104 cells/well in a 48-well plate and incubated for 48 h. After incubation for 48 h, cells were incubated in 1 mL Krebs-Ringer Bicarbonate Buffer (KRB buffer: 10 mM D-glucose, 0.49 mM MgCl2, 119.78 mM NaCl, 4.56 mM KCl, 0.7 mM Na2HPO4, 1.3 mM NaH2PO4, 14.99 mM NaHCO3) supplemented with various concentration of test samples for 30 min. Media conditioned for 30 min were collected and centrifuged at 12,000 rpm for 10 min. The supernatant was collected and the levels of insulin in supernatant were assayed using the insulin ELISA kit (Shibayagi Co., Gunma, Japan) according to the manufacturer’s instructions.
L6 rat skeletal muscle cells were purchased from the Korean Cell Bank (Seoul, Korea). L6 cells were cultured in Dulbecco Modified Eagle Medium (DMEM) supplemented with 100 mL/L FBS, 100,000 U/L penicillin, and 100 mg/L streptomycin at 37°C in a humidified atmosphere of 5% CO2. When cells were 80% confluent, they were subcultured using 0.25% trypsin-2.65 mM EDTA, and the medium was changed every 2 days.
L6 cells were plated at a density of 5 x 104 cells in a 48-well plate and incubated for 24 h. After incubation for 24 h, cells were treated with various concentrations of test samples for 24 h. Cell viability were measured by MTT assay, as described above.
L6 cells were plated at a density of 1 x 104 cells/well in a 96-well plate and incubated for 24 h. Cells were then cultured in DMEM containing 20 mL/L FBS for 4 days to induce myotube differentiation. Following differentiation induction, cells were treated with the indicated concentrations of test samples for 2 h. Glucose uptake was estimated using a glucose uptake colorimetric assay kit (Biovision, Milpitas, CA, USA) according to the manufacture’s instructions.
3. RESULTS and DISCUSSION
The isolated compounds were elucidated as (+)- catehin, (-)-epicatechin, taxifolin, taxifolin-3′-O-β-D- (+)-glucose and ρ-courmaric acid (Fig. 1) and the weight of the isolated compounds was indicated in Table 2.
Compounds | Weight |
---|---|
(+)-catechin | 469.8 mg |
(-)-epicatechin | 2.2 mg |
taxifolin | 15.4 mg |
taxifolin-3′-O-β-D-(+)-glucose | 321.2 mg |
ρ-courmaric acid | 18.5 mg |
The cytotoxicities of the fractions of Korean red pine extracts and the isolated compounds on HIT-T15 cells were tested by MTT assay.
The crude extract indicated 97 % of cell viability at 5 μg/mℓ of concentration, but the higher than 1 μg/mℓ showed cytotoxicity (Fig. 2). Kim et. al. (2018) reported that Korean red pine inner bark extracts indicated 100 % of cell viability at 10 μg/mℓ of concentration on neuron-like PC-12 cells and Lee et al. (2013) also reported that PineXol showed 100 % of cell viability up to 200 μg/mℓ of concentration on mature 3T3-L1 cells. Both of EtOAc and H2O soluble fractions gave 100 % of cell viability up to 50 μg/mℓ, but in the higher concentration, EtOAc soluble fraction indicated cytotoxicity (Fig. 3 and 4).
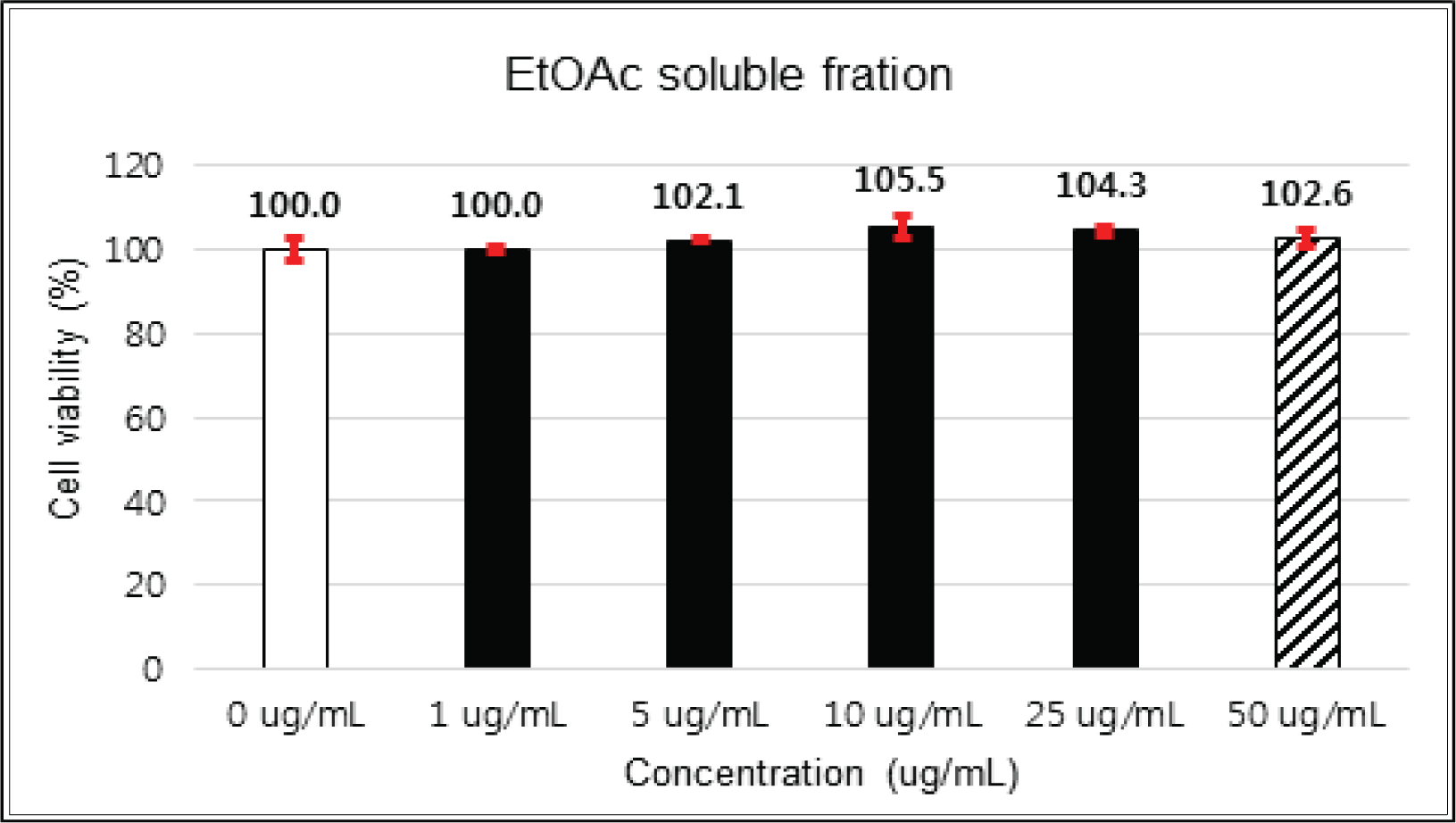
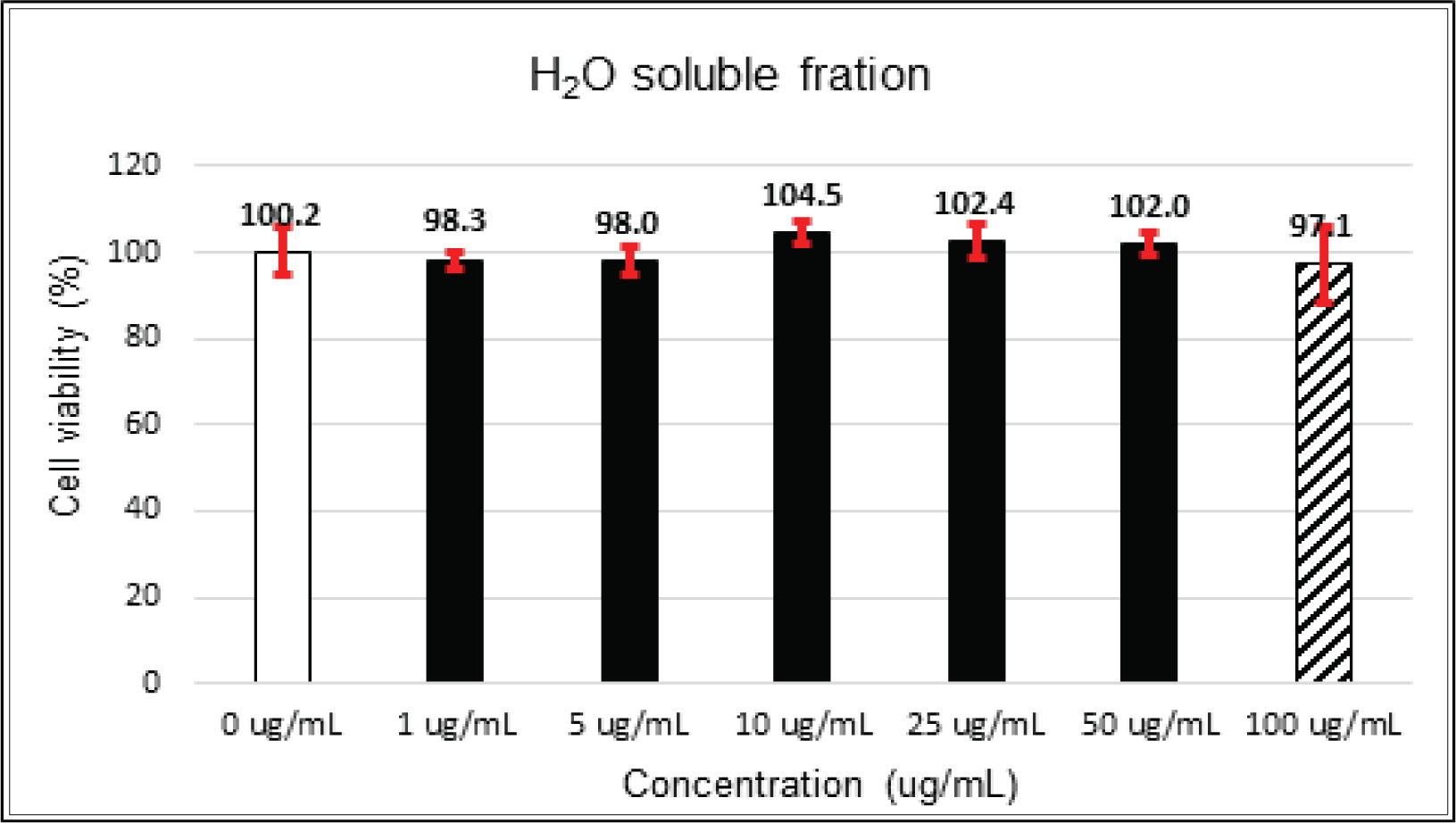
(+)-catechin showed 100 % of cell viability up to 100 μg/mℓ of concentration. (-)-epicatechin and taxifolin- 3′-O-β-D-(+)-glucose gave 93 % of cell viability at 5 μg/mℓ of concentration, but indicated cytotoxicity in the higher (Figs. 5, 6 and 8). Taxifolin and ρ-coumaric acid gave almost 100% of cell viability up to 25 μg/mℓ of concentration and more than 90 % of cell viability at 25 μg/mℓ of concentration (Figs. 7 and 9). Based on these results, the following tests were done within the range of nontoxic concentration on HIT-T15 cells.
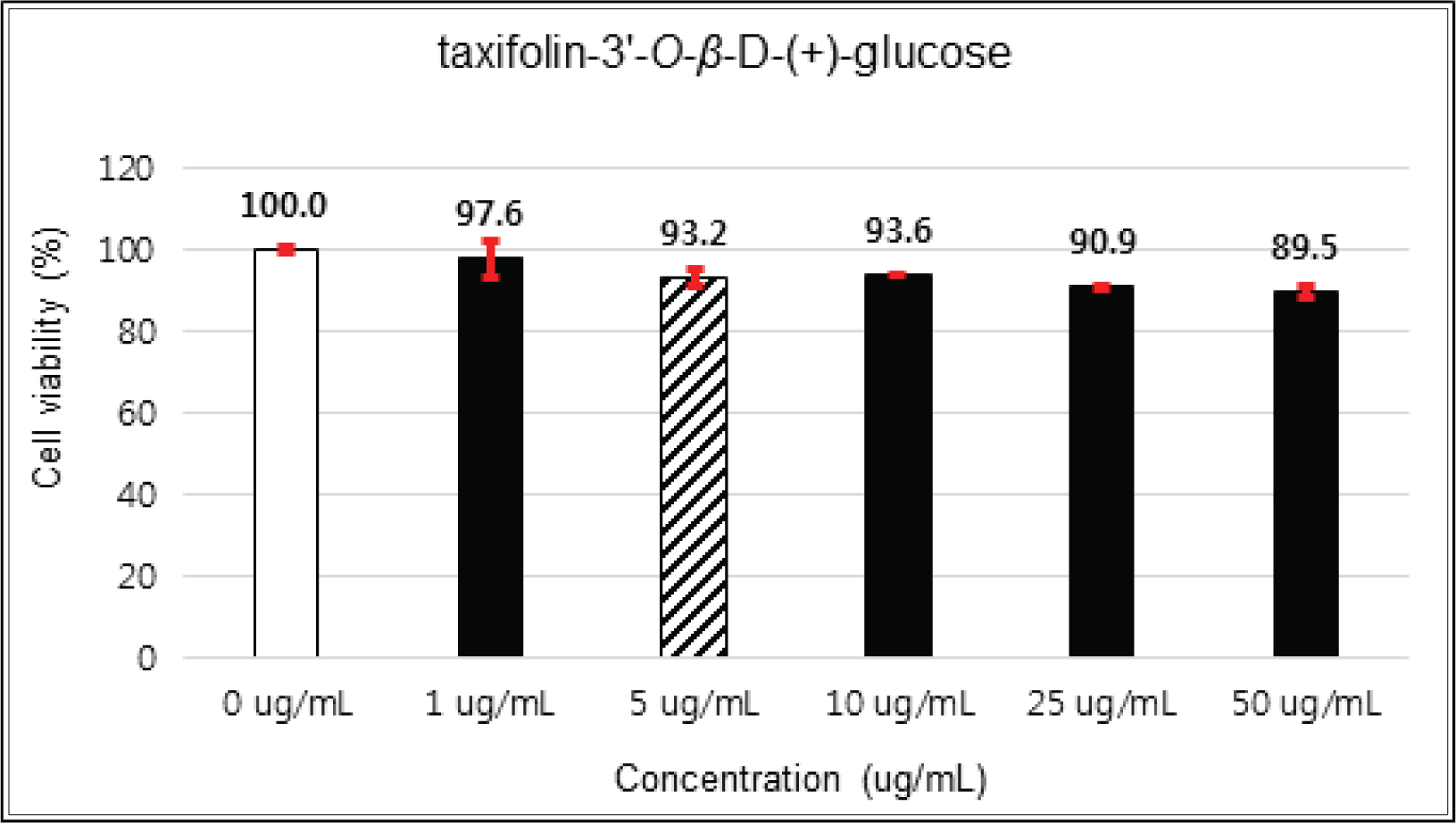
The variations of β-cells insulin secretion were checked by the addition concentrations of the crude, fractions and isolated compounds of Korean red pine inner bark extracts.
The crude showed 116 % of β-cells insulin secretion at 5 μg/mℓ of concentration, but EtOAc and H2O soluble fractions did not indicate any significant difference (Fig. 10, 11 and 12). (+)-catechin indicated 98.3 % at 25 μg/mℓ, 102 % at 50 μg/mℓ, and 99 % at 100 μg/mℓ without any variations by the concentration change (Fig. 13). (-)-epicatechin showed 110 % at 1.25 μg/mℓ and 113 % at 2.5 μg/mℓ (Fig. 14). Taxifolin gave 109 % at 1.25 μg/mℓ and 128 % at 25 μg/mℓ, but decreased to 118 % at 50 μg/mℓ (Fig. 15). In taxifolin-3′-O-β -D-(+)-glucose, β-cells insulin secretion was 107 % at 1.25 μg/mℓ and 2.5 μg/mℓ, but had no significant difference compare to the control (Fig. 16). ρ-coumaric acid showed 117 % at 12.5 μg/mℓ and 112 % at 25 μg/mℓ (Fig. 17). Based on the above results, the Korean pine inner bark extracts did not improve the insulin secretion of pancreatic cells.
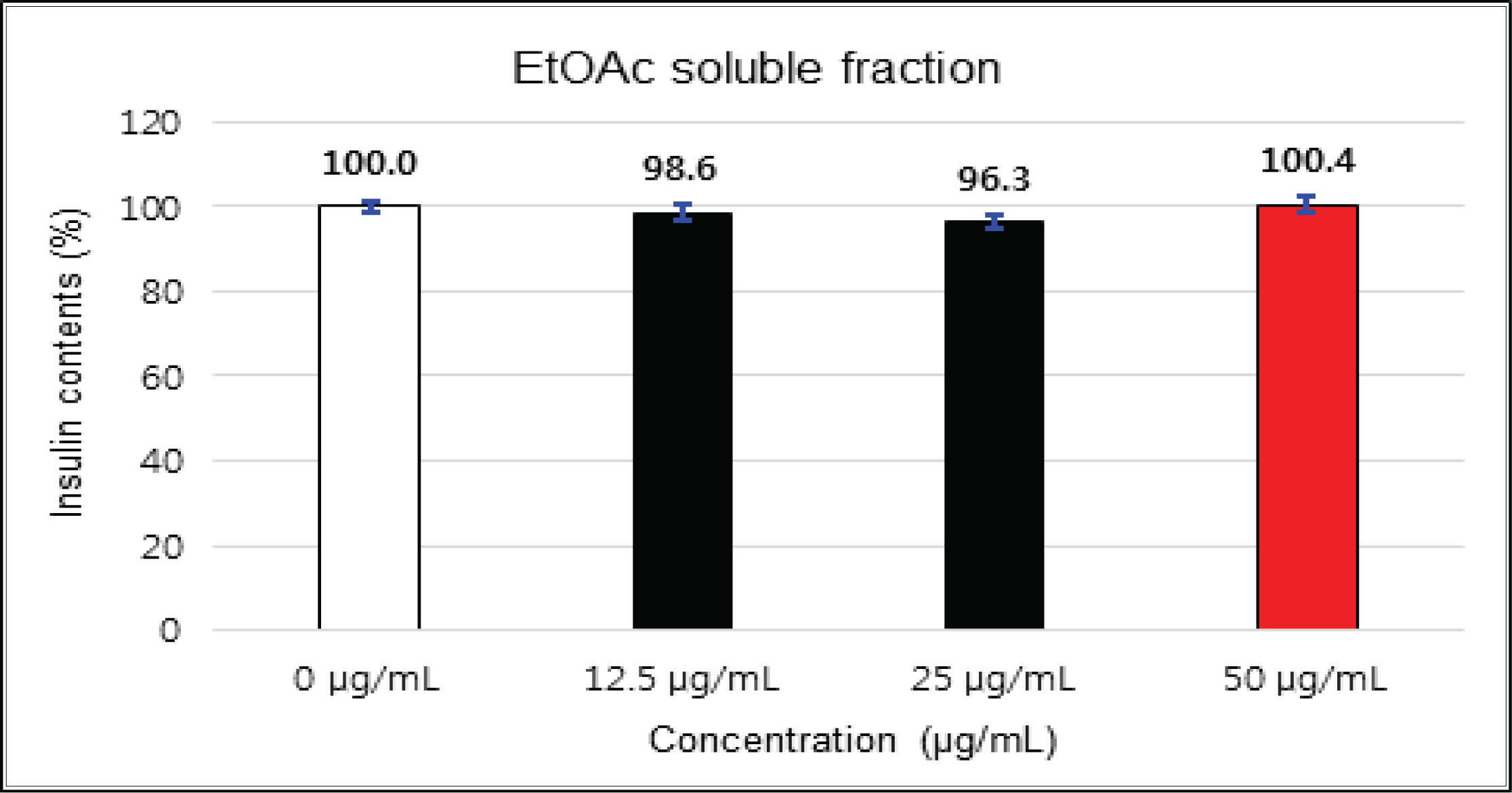
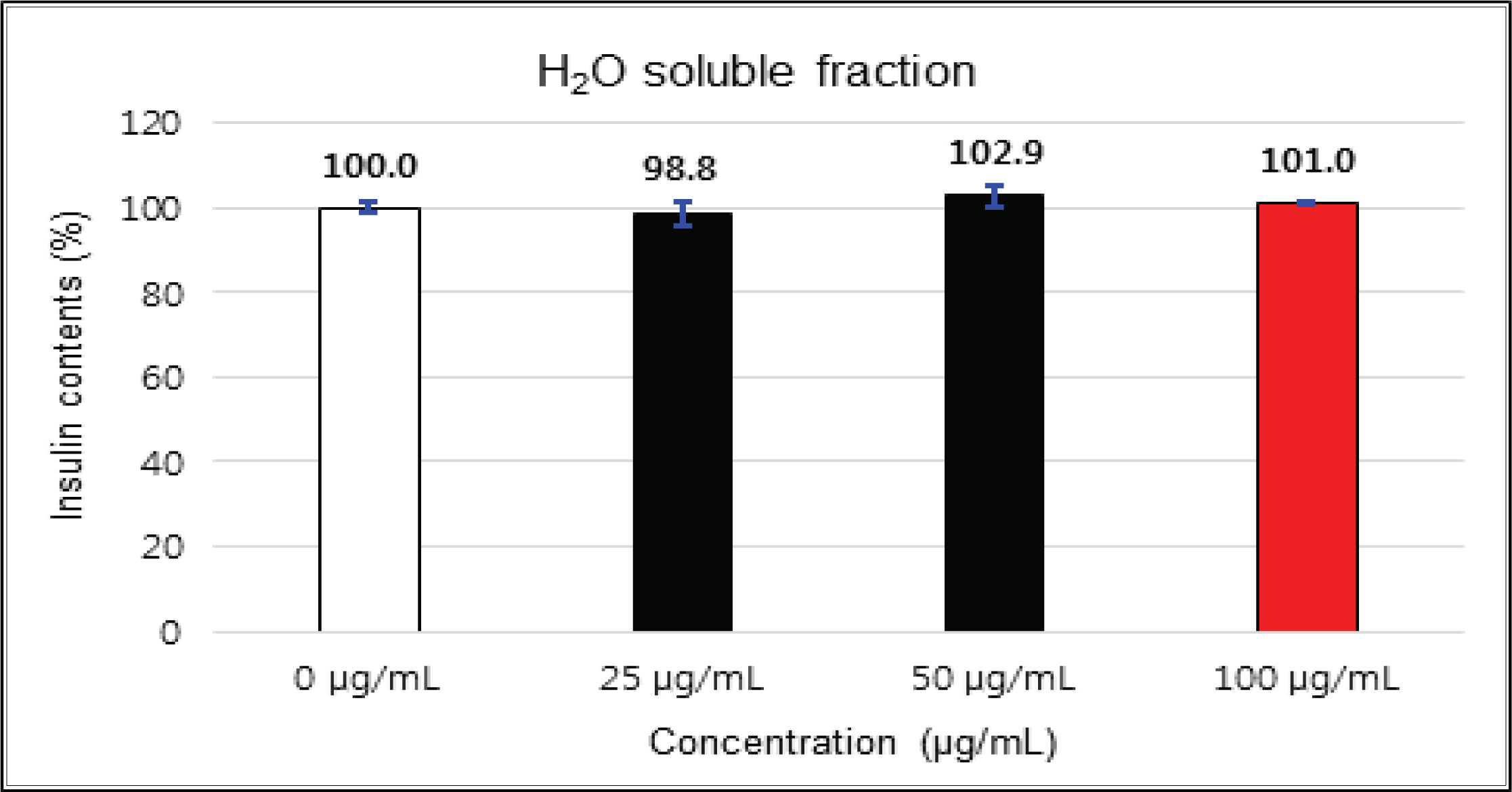
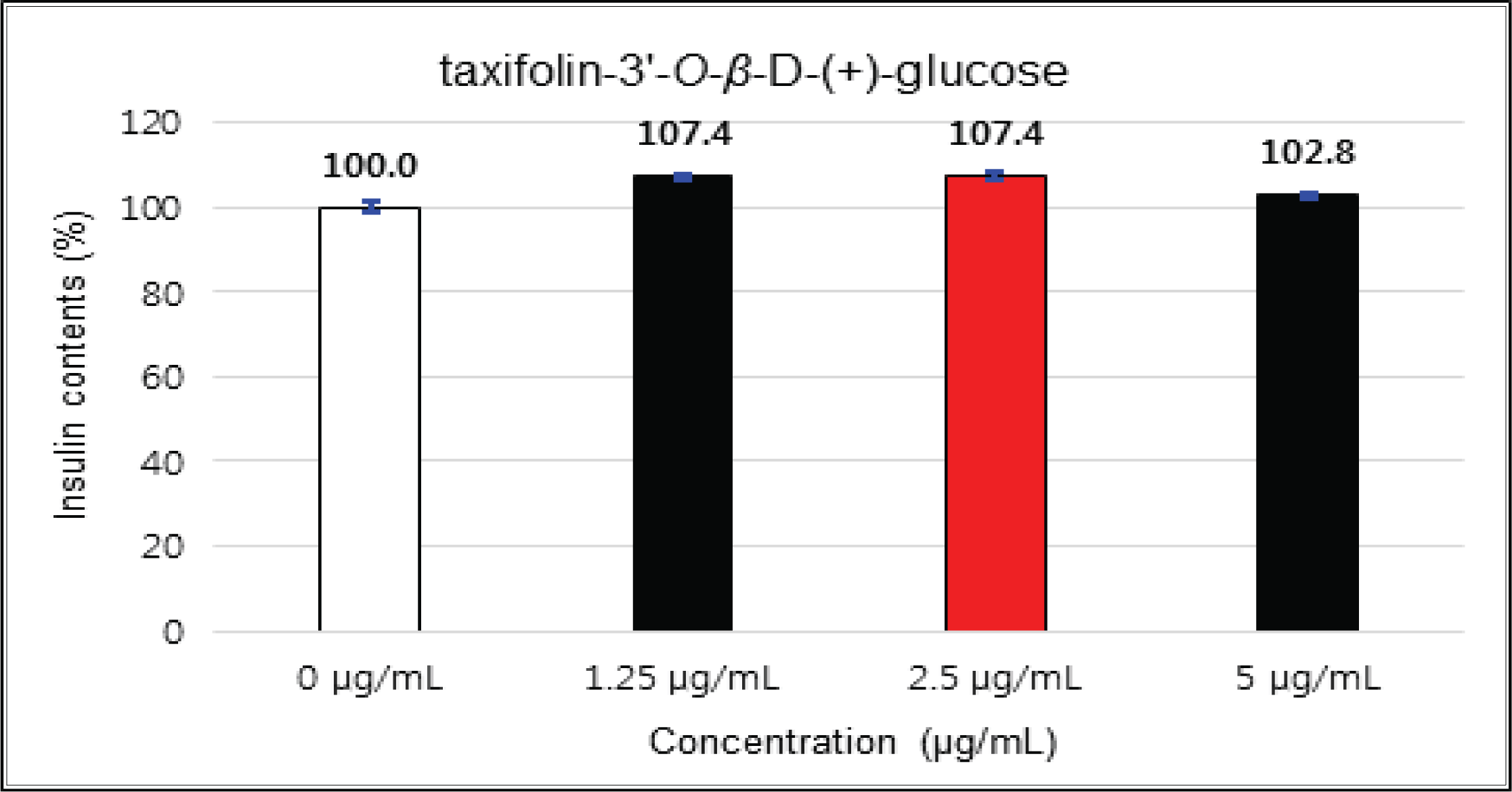
The cytotoxicities of the fractions of Korean red pine extracts and the isolated compounds on L6 cells were tested by MTT assay using 0, 1, 5, 10, 25, 50 and 100 μg/ml of treating concentration. (Fig. 18, 19, 20, 21).
The cytotoxicities of the fractions of Korean red pine extracts and the isolated compounds on L6 cells were tested by MTT assay using 0, 1, 5, 10, 25, 50 and 100 μg/ml of treating concentration. (Fig. 22, 23, 24, 25).
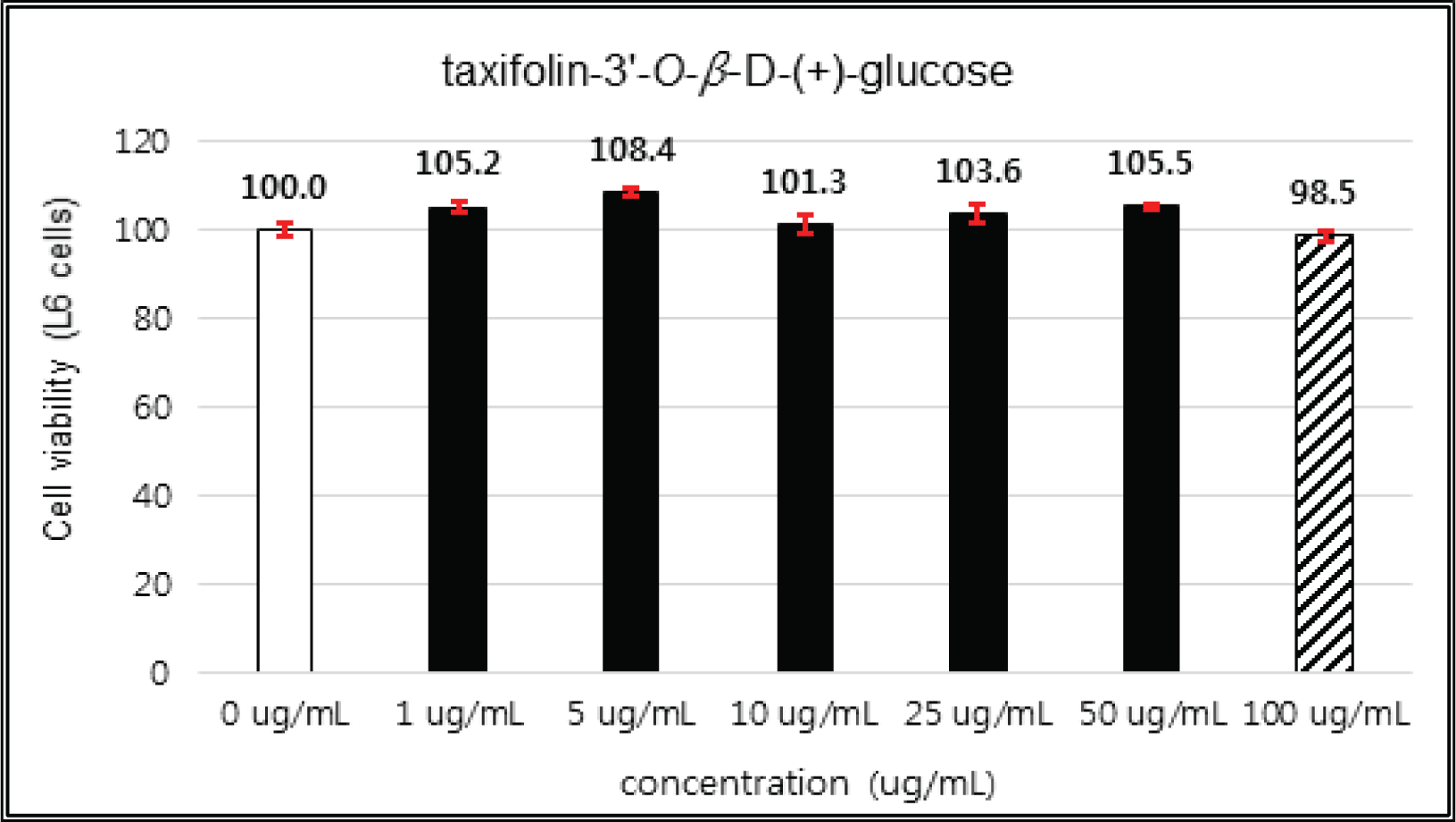
The glucose uptake activities were checked by the concentrations of the crude, fractions and isolated compounds of Korean red pine inner bark extracts.
The activities of crude and H2O soluble fraction were increased as the concentration increases. The crude and H2O soluble fraction showed the greatest uptake 221 % at 5 μg/mℓ and 266 % at 100 μg/mℓ, respectively. These uptake activities are higher than 1 μM insulin and similar to 2 mM Metformin, one of commercial agents. EtOAc soluble fraction showed higher activities in all concentrations compare to 1 μM insulin and was better than 2 mM Metformin as 267 % at 10 μg/mℓ (Fig. 26, 27 and 28).
The uptake activities of (+)-catechin and (-)-epicatehin were increased as the concentration increases. (+)-catechin was the highest as 264 % at 100 μg/mℓ and (-)-epicatechin was 260 and 276 % at 50 μg/mℓ and 100 μg/mℓ, respectively, indicating the higher potentials compare to 1 μM insulin and 2 mM Metformin (Fig. 29 and 30). These results are very similar to the report by Ho et al. (2017) that the glucose uptake activities of (+)-catechin and (-)-epicatehin on human myotubes and HepG2-cells were dependent on the concentration and that (-)-epicatehin was better than (+)-catechin. Taxifolin gave the glucose uptake activities 122, 150, and 207 % at 12.5 μg/mℓ, 25 μg/mℓ, and 50 μg/mℓ, respectively, similar to 1 μM insulin (Fig. 31). Taxifolin-3′-O-β-D-(+)-glucose showed lower activity than 1 μM insulin up to 50 μg/mℓ of concentration, but indicated better potential than 2 mM Metformin as 270 % at 100 μg/mℓ (Fig. 32). Finally ρ-coumaric acid showed higher glucose uptake activity than 270 % in all concentrations, indicating the higher than 1 μM insulin and 2 mM Metformin (Fig. 33).
4. CONCLUSION
β-cells insulin secretion and glucose uptake activity in muscle cells for the evaluation of antidiabetic activity were checked on the crude, EtOAc and H2O soluble fraction of Korean red pine inner bark extracts, including 5 compounds isolated by column chromatography.
The cytotoxicity of the fractions of Korean red pine extracts and the isolated compounds on HIT-T15 and L6 cells were tested by MTT assay using 0, 1, 5, 10, 25, 50 and 100 μg/ml of treating concentration. Based on the cytotoxic results, the following tests were done within the range of nontoxic concentration on HIT-T15 and L6 cells.
The crude showed β-cells insulin secretion of 116 % at 5 μg/mℓ of concentration, but EtOAc and H2O soluble fractions did not indicate any significant difference. (+)-catechin did not indicate any significant variations by the concentration change. (-)-epicatechin was 113 % at 2.5 μg/mℓ and taxifolin gave 128 % at 25 μg/mℓ, but decreased to 118 % at 50 μg/mℓ. Taxifolin-3′-O-β-D-(+)-glucose had no significant variations by the concentration change. ρ-coumaric acid indicated 117 % at 12.5 μg/mℓ and 112 % at 25 μg/mg.
The glucose uptake activities were checked by the concentrations of the crude, fractions and isolated compounds of Korean red pine inner bark extracts.
The glucose uptake activities were 221 % at 5 μg/mℓ in the crude and 266 % at 100 μg/mℓ in H2O soluble fraction. These values are higher than 1 μM insulin and similar to 2 mM Metformin, one of commercial agents. EtOAc soluble fraction showed higher activities in all concentrations compare to 1 μM insulin and was better than 2 mM Metformin as 267 % at 10 μg/mℓ.
(+)-catechin was 264 % at 100 μg/mℓ and (-)-epicatechin was 260 and 276 % at 50 μg/mℓ and 100 μg/mℓ, respectively, indicating the higher potentials compare to 1 μM insulin and 2 mM Metformin. Taxifolin gave the activity of 207 % at 50 μg/mℓ, similar to 1 μM insulin. Taxifolin-3′-O-β-D-(+)-glucose indicated better potential than 2 mM Metformin as 270 % at 100 μg/mℓ. ρ-coumaric acid showed higher glucose uptake activity than 270 % in all concentrations, indicating the better activities than 1 μM insulin and 2 mM Metformin.
Based on the above results, the crude, EtOAc soluble fraction, H2O soluble fraction, and isolated compounds of Korean red pine extracts did not show any significant results on the insulin secretion of pancreas β-cells except taxifolin, but indicated the significant differences on the glucose uptake activities. Korean pine inner bark extracts had a good potential to control blood glucose using glucose uptake activity. Therefore, Korean red pine inner bark extracts can be one of useful natural resources to substitute a synthetic hypoglycemic agent in the future.