1. INTRODUCTION
Mangium (Acacia mangium), which produces short-fibered wood, has come to dominate plantations in Indonesia because of the high demand for pulp and paper, which reached 410 million tons in 2017 (Hermes, 2018). Mangium bark is consequently an abundant waste byproduct in the Indonesia wood industries, particularly in the pulp and paper industry, and it is often used as firewood or as fuel for industry. Mangium bark contains a high level of tannin, composed of naturally occurring polyphenolic compounds that are soluble in water (Hoong et al., 2011). These compounds can be used in various applications, including the manufacture of wood adhesives.
Santoso et al. (2016) reported that merbau (Instia spp.) wood extracts contained flavonoid oligomers with up to six repeating units, and the extracts could be used in adhesives for glulam manufacturing, especially with medium-density woods. At present, the commercial and industrial tannin extracts usable in wood adhesives are mainly obtained from the bark of mimosa (Acacia mearnsii), quebracho (Schinopsis balansae), and pines, including Pinus merkusii (Fradinho et al., 2002; Panamgama, 2007; Vazquez et al., 2000) and Pinus radiata barks (Valenzuela et al., 2012). Previous research demonstrated that condensed tannin extracts can be successfully used as substitutes for phenol in the production of phenol–formaldehyde resins, resorcinol–formaldehyde resins, and phenol–resorcinol–formaldehyde (PRF) resins and that condensed tannin–formaldehyde wood adhesives can be used for interior and exterior wood bonding of products such as particleboard and plywood (Pizzi, 1983). These naturally occurring polyphenolic compounds can also be used as a raw material for adhesives in manufacturing of glued laminated timber (glulam) (Lestari et al., 2015) and laminated board (Hendrik et al., 2016).
Indonesian plantation forests are dominated by fast-growing tree species such as jabon (Anthocephalus cadamba), sengon (Falcataria moluccana), and mangium. These species have cutting cycles of 5–10 years, and a diameter of less than 30 cm, and their quality is inferior compared to logs from natural forests (Hadi et al., 2015). Glulam is one option for improving the quality of wood and its physical and mechanical properties, with no restriction on its dimensions. The objectives of the current study were to characterize mangium wood extract and to determine the physical and mechanical properties of glulams made from three fast-growing wood species and adhesive based on either tannin from the mangium extract or PRF. The physical and mechanical properties, shear strength, and delamination of the glulams were evaluated, and the results were compared to the Japanese Agricultural Standard (JAS) 234 (2003) for glued laminated timber.
2. MATERIALS and METHODS
Chipped mangium stem bark was oven-dried and underwent hot-water extraction (70 °C–80 °C), and the resultant liquid extract was then filtered. The filtered extract then underwent spray drying, which produced tannin powder. Tannin yield was calculated from the difference between the weight of the tannin powder and the weight of the oven-dried bark prior to extraction. The Stiasny number method, as described by Yazaki and Collins (1997), was used to determine reactivity of the polyphenol with formaldehyde. A total of 0.12 g of the tannin powder was added to a 150-mL flask containing 10 mL of water. Aqueous formaldehyde (37 %; 2 mL) and hydrochloric acid (10 N; 1 mL) were added to the solution, and the mixture was then heated to 100 °C for 30 min. The reaction mixture was filtered while it was still hot, and the precipitate was dried in an oven at 105 °C to constant weight. The Stiasny number was determined according to the following equation:
The tannin powder was analyzed by matrix-assisted laser desorption/ionization-time of flight (MALDITOF; Shimadzu Biotech Axima Performance 2.9.3.20110624), x-ray diffraction (XRD, Shimadzu, Kyoto, Japan), and by pyrolysis gas chromatography–mass spectrometry (GCMS; Py-GCMS-QPXP-2010, Shimadzu, Japan). Mangium tannin adhesive was made by mixing tannin powder and mangium extract liquid (1:4 m/m) to produce a tannin solution. Next, 9 g of resorcinol, 10 mL of formaldehyde, and 10 mL of sodium hydroxide 40 % were added for each 100 mL of the tannin solution. The mixture was stirred at room temperature for approximately 15 min.
Glulam was made with wood from 5- to 7-year-old trees of three species, sengon, jabon, and mangium. The trees were harvested from Bogor, West Java, Indonesia. The lumber was processed into lamina sheets that were 1 cm × 6 cm × 120 cm in thickness, width, and length, respectively. The laminas were air dried and then kiln-dried to a moisture content of approximately 12 %. Lamina sorting was performed by modulus of elasticity (MOE) prediction using a nondestructive device (Panther version MPK-5, IPB, Bogor, Indonesia) for quality sorting. Laminas used for the face and back layers of glulam had higher MOE values than the core laminas. Each lamina was bonded using either mangium tannin adhesive, which had a solids content of 24.02 % and a viscosity of 95 cP, or PRF, a polymer consisting of a base resin and hardener. Glue was double spread at 280 g/m2, and the laminas were then pressed using a cold press machine at a pressure of 12 MPa for 4 h and then clamped for 20 h. The resultant five-layer homogeneous glulam measured 5 cm × 6 cm × 120 cm in thickness, width, and length, respectively. The glulam was cut to various sizes for different tests as shown in Fig. 1. For comparison purposes, solid wood representing a material without adhesive was used for control samples. The solid wood samples were the same size as the glulam samples. Five test specimens were used for each treatment.
The physical and mechanical properties of glulam were tested according to the standard for glued laminated timber (JAS 234-2003 (2003)). Physical properties were tested based on the moisture content, density, and width shrinkage, and mechanical properties were tested with a Universal Testing Machine (Universal Testing Machine, Shimadzu Corporation, Kyoto, Japan) to determine the modulus of rupture (MOR), MOE, and shear strength. For MOR and MOE tests, one-point loading was applied with a span of 70 cm and a load speed of 3 mm/min.
Hot water delamination tests were done by boiling each test specimen in water (100 °C) for 4 h, soaking it in water at room temperature for 1 h, and then placing it in an oven at 70 ± 3 °C for 18 h. The cold water delamination test was carried out by soaking test specimens in water at room temperature for 6 h and then putting them in the oven at a temperature of 40 ± 3 °C for 18 h. The delamination ratio was calculated using Eq. 2.
To test formaldehyde emissions, samples sized 2.5 cm × 2.5 cm × 5 cm were hung in bottles containing 25 mL of distilled water without coming into contact with the water. The bottles containing the samples were placed in an oven at 40 ± 2 °C for 24 h. Ten milliliters of water from each bottle was mixed with 10 mL of acetyl ammonium acetate reagent and the absorbance of the solution was measured with a spectrophotometer at λ = 412 nm to determine the formaldehyde concentration.
Data analysis was undertaken using a factorial design. The first factor was wood species (i.e., sengon, jabon, and mangium), and the second factor was the type of adhesive (i.e., solid or nonadhesive, tannin adhesive, and PRF). If the analysis of variance indicated that any treatment was significantly different (P ≤ 0.05), Duncan’s multi-range test was used for further analysis.
3. RESULTS and DISCUSSION
The Stiasny number indicates the reactivity of tannin with formaldehyde. The reactivity can be affected by the bark freshness, source of wood, tree habitat, and extraction method (Achmadi, 1990). Tannin extract accounted for 377.6 g of the 3 kg of mangium bark (12.59 % by weight) that underwent extraction, and it had a Stiasny number of 47.22 % (w/w).
The pyrolysis GCMS chromatograph (Fig. 2) indicated that the mangium tannin extract contained phenol, and its molecular weight was 866 g/mol, which was much lower than previously reported values for tannin from pine (3200 g/mol) and mimosa (1250 g/mol) (von Leyser and Pizzi, 1990). The pyrolysis GCMS showed that the mangium tannin extract was dominated by hydroxyl groups, aromatic groups, and ether groups. Pyrolysis GCMS revealed that the phenolic compounds accounted for 49.08 % (w/w) of the extract and were dominated by 1,3-benzenediol, similar to the results of Santoso et al. (2016), who found that liquid extract of merbau wood was dominated by 1,3-benzenediol.
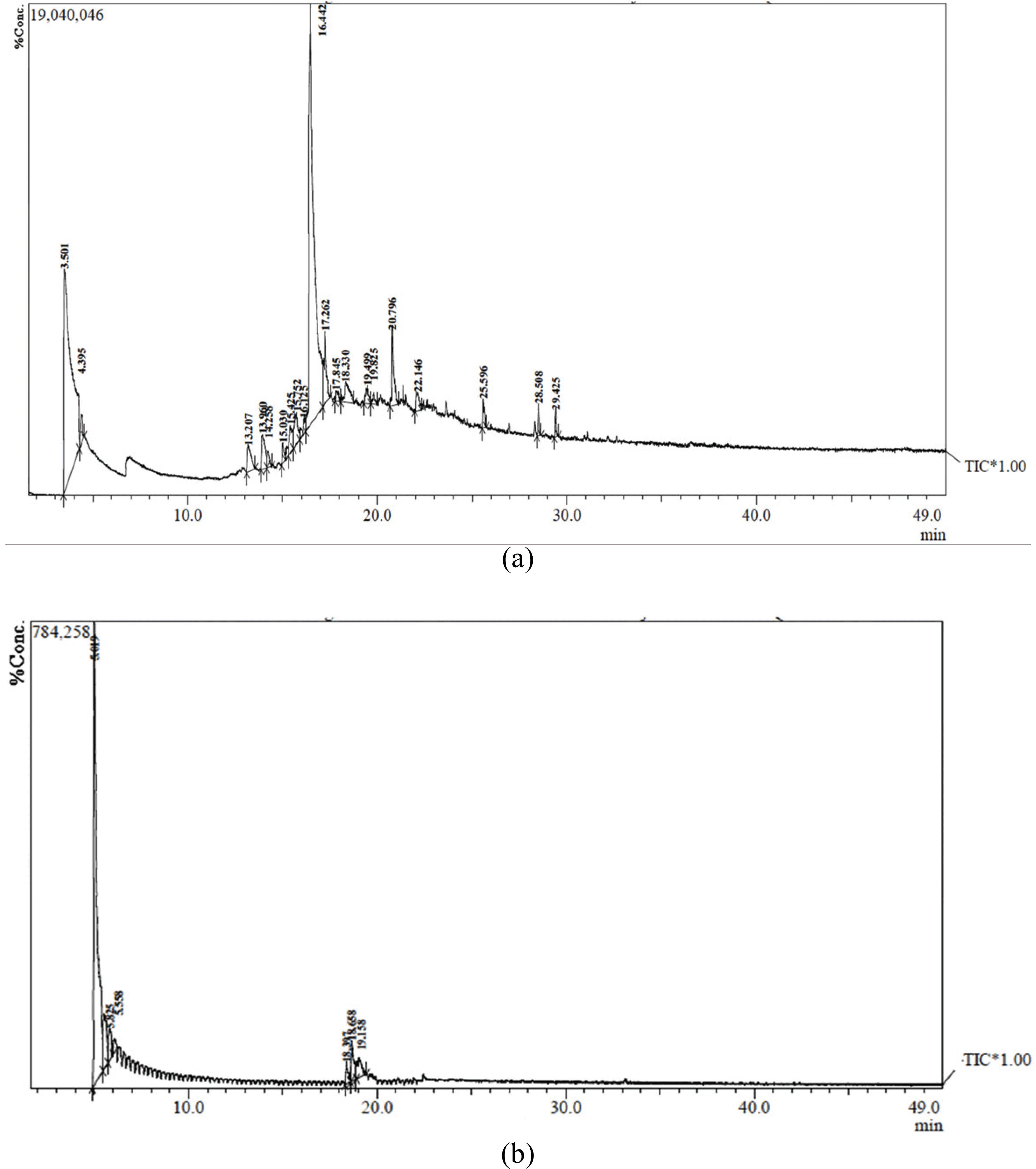
The pyrolysis GCMS chromatograph (Fig. 2a) indicated that the mangium tannin contained phenol and many other peaks. Therefore, in Table 1 the total phenolic content was decreased in tannin resorcinol formaldehyde (TRF), due to reaction between mangium tannin and resorcinol formaldehyde forming a new chemical component. Based on the retention times obtained, mangium tannin appeared to be able to react with resorcinol and formaldehyde.
Fig. 3 shows a representative MALDI-TOF spectrum of mangium tannin, with a molecular weight ranging from 30 to 2000 Da. The molecular weight of the predominant repeating unit was 272 Da, indicating that this tannin was a profisetinidin. The MALDI peaks indicated the presence of oligomers in the tannin, with the largest being heptamers (2075 Da), and the distribution is shown in Table 2. The flavonoid repeating units present in this tannin extract were types A for recorsinol-katekol (274 Da), B for recorsinol-pyrogallol (290 Da), and C for floroglusinoll-pyrogallol (306 Da) (Fig. 4). Table 2 shows that many valid combinations of different repeating units are possible.
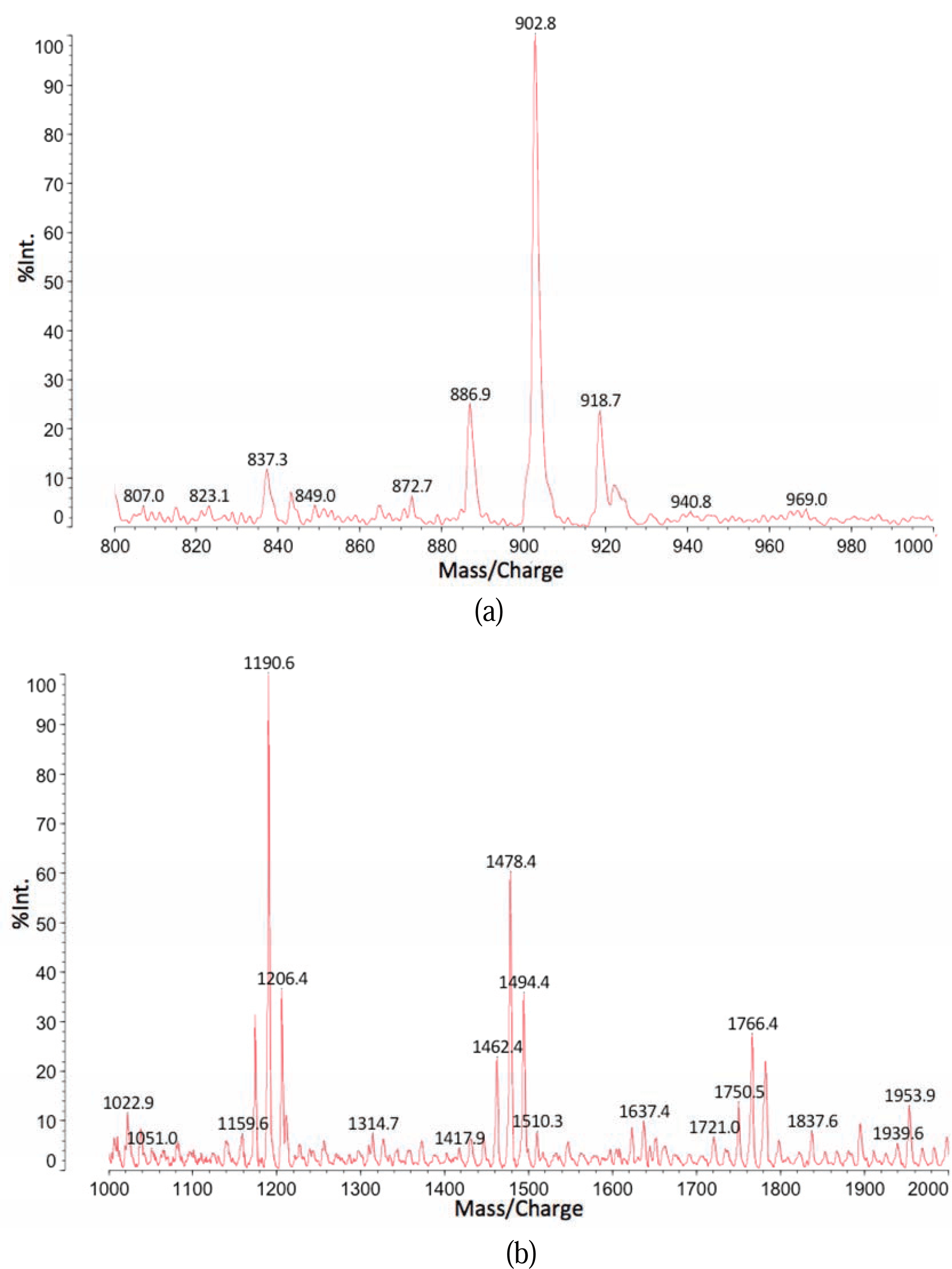
Copolymerization reactions may lead to changes in the molecular and crystalline structure of the mangium tannin, and an understanding of its molecular and crystalline structures and the changes would provide valuable information regarding bonding performance. The XRD patterns of mangium extract and TRF adhesive are shown in Fig. 5 the y-axis represents the intensity. The results indicate that the degree of crystallinity of the mangium tannin powder increased from 14.8 % to 22.34 % when it copolymerized with resorcinol and formaldehyde.
The physical properties of glulam and solid wood are shown in Table 3. Glulam and mangium solid wood had higher densities (0.66 ± 0.02 g/cm3) than glulam and jabon solid wood (0.34 ± 0.01 g/cm3) and sengon wood (0.33 ± 0.02 g/cm3). The analysis of variance showed that sengon, jabon, and mangium woods had significantly different densities (Table 4). However, the type of adhesive did not significantly affect the density, and the three types of product (solid wood, glulam bonded by TRF, and glulam bonded by PRF) had the same density values.
Table 3 shows that the moisture content of the sengon (14.37± 0.36 %) and jabon glulam (14.1± 0.39 %) were higher than mangium glulam 8.55 ± 0.80%. The moisture content fulfilled the JAS 234 (2003) standard, which sets the allowable moisture content at less than 15 %. According to the analysis of variance shown in Table 4, the moisture content of glulam was affected by the wood species, the type of adhesive, and the interaction between wood species and type of adhesive. Based on Duncan’s multi range test sengon and jabon were not different from one another (Table 5), but the glulam was different from the solid wood (Table 6).
The MOE values of solid wood and glulam are shown in Table 7. Only mangium glulams (107,318 ± 4,674 kgf/cm2) met the standard JAS 234 (2003), which sets the allowable MOE at more than 75,000 kgf/cm2. The MOE values of glulam made from sengon and jabon wood bonded by tannin adhesive and PRF were lower than those of solid wood. Compare the glulam from jabon wood glued with TRF (47,114 kgf/cm2) had a lower value than glulam 3-layers glued with isocyanate (56,084 kgf/cm2) (Lestari et al., 2018). According to the analysis of variance shown in Table 4, the MOE of glulam was not affected by the type of adhesive, but it was affected by wood species and the interaction between wood species and type of adhesive.
The MOR values for glulam and solid wood are shown in Table 7. All samples made with TRF adhesive had lower MOR values than those made with PRF and the solid wood. However, all glulams had an MOR that met JAS 234 (2003), which sets the allowable MOR at more than 300 kgf/cm2. Compared to larix glulam which was manufactured with resorcinol formaldehyde adhesive using glue spread 300 g/m2 reaching MOR 392 kgf/cm2 (Lee and Hong, 2016), the MOR of mangium glulam using TRF was 379 kgf/cm2 or a little bit lower of larix glulam, but if using PRF the MOR was 724 kgf/cm2 or much higher than larix glulam.
Based on the analysis of variance presented in Table 4, MOR values were significantly different based on the species of wood, the type adhesive, and their interactions. Based on Duncan’s test (Table 5), sengon and jabon were not significantly different.
Shear strength tests were carried out under dry and wet conditions. Table 7 shows that glulam (29.49 ± 11.07 %) had lower shear strength values than solid wood (54.45 ± 10.61 %). All glulams failed to meet the JAS standard of more than 54 kgf/cm2 for shear strength. Compared to larch cross-laminated timber with resorcinol adhesive reaching shear strength 39 kgf/cm2 (Song and Hong, 2016), the shear strength of jabon glulam using TRF was 32 kgf/cm2 or a little bit lower of larch CLT, but if using PRF the shear strength was 41 kgf/cm2 or much higher than larch CLT.
According to an analysis of variance (Table 6), the species of wood and the type of adhesive affected the shear strength of glulam. Based on the results of Duncan’s test (Table 6), TRF adhesive was associated with lower shear strength values compared with those associated with PRF and solid wood. Under dry conditions, sengon and jabon were significantly different, but jabon and mangium were not. Under wet conditions, sengon and jabon were not significantly different from each other, but both were significantly different from mangium. This outcome was due to some mangium sample bonds having failed at the glue line.
The results of cold-water delamination (Table 8) indicate that mangium glulam bonded by tannin had the highest ratio of delamination (23.80 %). This result was likely due to mangium having a high density, which prevented the adhesive penetrating deeply into the wood before the curing process. Based on JAS 234 (2003), only sengon and jabon glulams bonded with TRF adhesive met the Japanese standard of less than 5 % delamination. The delamination of both species had a good performance as glulam made from Japanese larch, Korean pine, and Japanese cedar using melamine urea formaldehyde adhesive with delamination values less than 5 % as mentioned by Hong et al. (2017).
In the hot-water delamination test, glulam made from mangium presented delamination, but the extent of it depended on the adhesive used. Mangium glulam bonded with TRF adhesive had the highest delamination value (67.80 %), but mangium bonded by PRF met the JAS 234 (2003) requirement of less than 10% delamination. As in the cold-water test, sengon, jabon, and mangium-PRF glulam fulfilled the JAS standard for both cold-water (maximum 5 %) and hot-water conditions (maximum 10 %). According to the analysis of variance (Table 4), the outcome of delamination tests was affected by wood species, type of adhesive, and their interactions. Duncan’s test (Table 5) showed the same results for the delamination tests in hot and cold water; sengon and jabon were not different from each other, but both were different from mangium.
Table 9 shows that the formaldehyde emissions of sengon, jabon, and mangium glulams can be classified as F****, which is the lowest level of emission and the best class according to JAS 234 (2003). Tannin from bark extracts is rich in phenolic compounds, and thus it may be able to absorb free formaldehyde and at the same time provide strength to the bond (Hoong et al., 2012). The formaldehyde emission of this research reached 0.04 to 0.05 mg/L and these values were lower than wood-based composite panels with different surface lamination materials, which reached 0.525 to 1.148 mg/L (Park et al., 2016).
Wood species | Formaldehyde emissions (mg/L) | Grade* |
---|---|---|
Jabon | 0.05 | F**** |
Sengon | 0.04 | F**** |
Mangium | 0.04 | F**** |
Categorized by standard JAS 234 (2003)
4. CONCLUSION
-
The condensed tannin extract from mangium bark was 49.08 % phenolic compounds and had a molecular weight of 4745. The degree of crystallinity was 14.8 %, and the Stiasny number was 47.22 %.
-
The density and moisture content of the glulams differed from those of the solid wood. Mangium had the lowest moisture content (9.58 %) and the highest density (0.66 g/cm3).
-
The MOE of the glulam made with TRF adhesive was not different from those of solid wood and PRF glulam, although none of the glulams fulfilled the JAS 234 (2003) standard. Tannin glulam had lower MOR and shear strength than solid wood and PRF glulam. The MOR of all glulams met the standard, but the glulams did not met the standard for shear strength in all conditions.
-
Mangium glulam with tannin adhesive had the highest delamination (67.80%).
-
All glulams had low formaldehyde emissions. Sengon, jabon, and mangium glulams were classified as F**** for formaldehyde emissions according to JAS 234 (2003).
-
In future work, the purity of tannin should be increased to improve the reactivity of the phenolic compounds with formaldehyde. Greater reactivity could increase the shear strength value of the glulam.