1. INTRODUCTION
Prebiotic sugars such as polysaccharides, oligosaccharides, and non-digestible carbohydrates have garnered great interest as functional food ingredients because they can alter the composition of the colonic microbiota in the human gut (Rycroft, Jones, Gibson and Rastall, 2001). These sugars have diverse structures and compositions that affect their functional properties and have long been used to improve the texture, water retention, and stabilization of emulsions. Furthermore, they are increasingly incorporated into health foods due to their prebiotic effect and their role as dietary fibers and mimetic fats (Warrand, 2006). Till date, prebiotic sugars that satisfy this definition include fructo-oligosaccharides, inulin, galacto-oligosaccharides, and gluco-oligosaccharides (Macfarlane et al., 2006). Several non-digestible food ingredients that are emerging as prebiotic sugar candidates include lactulose, galactans, fructans, xylo-oligosaccharides, β-glucans, or arabinoxylans (Sabater-Molina et al., 2009).
Recently, prebiotic sugars from various foods such as pomegranate peel (Zhu et al., 2015), Spirulina platensis (Kurd and Samavati, 2015), Clematis huchouensis Tamura (Zhang et al., 2014), longan pulp (Zhong and Wang, 2010), cereal (Michida et al., 2006), mushrooms (Aida et al., 2009), herbs (Guo et al., 2004), chicory root (Roberfroid, 2000), citrus fruits (Sendra et al., 2008), soybean (Crittendan and Playne, 1996), and potatoes (MacFarlane et al., 2006) have gained attention as alternative food supplements.
Moreover, lignocellulosic biomass seems to be a potential source for prebiotic sugars, since it contains carbohydrates such as cellulose (glucan) and hemicelluloses (arabinan, xylan, mannan, and galactan) (Linares-Pasten et al., 2014).
Meanwhile, steam explosion is a simple, low-cost, and environmentally friendly technology that causes hemicellulose depolymerization into soluble oligomers and lignin transformation, taking advantage of its high temperature profile (Lee et al., 2010). During steam explosion, a part of the lignocellulosic biomass becomes solubilized as polymers or monomers, while the other part becomes degraded or transformed into new components.
Recently, numerous reports have described the use of the response surface methodology (RSM) for the optimization of the extraction of polysaccharides from lignocellulosic biomasses, followed by the determination of the antioxidant properties of the extracted polysaccharides (Jung et al., 2012; Kim et al., 2015). RSM is a collection of statistical and mathematical techniques useful for the development, improvement, and optimization of processes. The main advantage of RSM is that it reduces the number of experimental trials needed to evaluate multiple variables and their interactions. However, to the best of our knowledge, there is no report on the use of RSM for the optimization of the conditions required for the extraction of a prebiotic-rich extract from P. densiflora or on the determination of their prebiotic activities. Although several studies have explored the steam explosion and extraction stages separately, optimizing the operating variables for each independent process, none of the studies have reported the simultaneous optimization of the two stages. Therefore, the aim of this study was to use RSM for the determination of the optimal operating factors in a two-stage process designed to obtain the highest yield of prebiotic sugars from P. densiflora extracts. We also investigated the prebiotic activity of the extracts.
2. MATERIALS and METHODS
Pinus densiflora was collected from the forest around the city of Hongcheon in South Korea. P. densiflora were chipped to a particle size of approximately 2 × 2 × 0.5 cm3 for steam explosion and stored at 20 °C at a moisture level below 10% ‒ 15%.
The chipped raw material was treated according to the conditions derived from the statistical model (Table 1). Each experimental condition was represented by a severity factor, which unifies time (t, min) and temperature (T, °C) in a single factor and allows to compare the different treatment conditions. The severity factor was calculated using equation (1) (Fernandez et al., 1999, Jung and Yang, 2016).
Temperature (°C) | Time (min) | Severity factor (Ro)a |
---|---|---|
160 | 5 | 2.47 |
180 | 5 | 3.05 |
10 | 3.36 | |
15 | 3.53 | |
200 | 5 | 3.64 |
10 | 3.94 | |
15 | 4.12 | |
220 | 5 | 4.23 |
10 | 4.53 | |
15 | 4.71 |
Steam temperatures of 160 °C to 220 °C and treatment times of 5 to 15 min were used to achieve the following values for the severity factor (Ro): 2.47, 3.05, 3.36, 3.53, 3.64, 3.94, 4.12, 4.23, 4.53, and 4.71 (Table 1).
Each steam-exploded wood sample (severity factor (Ro) = 2.47 ‒ 4.71, 1 g) was treated in a 250-mL flat-bottomed beaker with the determined extraction ratio of water to raw material (10 ‒ 50, mL/g) at an extraction temperature of 60 °C to 100 °C for an extraction time of 30 to 300 min. After the water extraction step, the resulting extracts were collected by centrifugation at 4,000 rpm for 15 min.
A three-variable Box-Behnken design (BBD) was used to optimize the two-stage process for the extraction of prebiotic sugars from P. densiflora. The selected variables were, namely, the severity factor (Ro) of steam explosion and temperature (°C) and the ratio of water to material (mL/g) for water extraction, the ranges for which were defined as 3.64 ‒4.12 for the severity factor, 80 °C ‒ 100 °C for the temperature, and 30 ‒ 50 for the ratio of water to material (mL/g). The levels of the variables were determined from a single-factor experimental analysis. The three variables were X1 (severity factor), X2 (extraction temperature), and X3 (ratio of water to material), and the three levels were coded 1, 0, and -1 for high, intermediate, and low levels, respectively. Table 2 lists the BBD matrix of the experiment with 17 trials and the response values used to develop the model. The experiments were performed in a random order to minimize the effects of external factors without taking the experimental design into account.
On the basis of the experimental data, a second-order polynomial model was established, which correlated the association between prebiotic sugar content and steam explosion treatment (severity factor, Ro) and the variables of the water extraction method (extraction temperature in °C, and ratio of water to material (mL/g)). The second-order polynomial model was given by equation (2):
where X1, X2, and X3 are the independent variables affecting the responses of Y, and β0, βi (i = 1, 2, and 3), βii (i = 1, 2, and 3), and βij (i = 1, 2, and 3) are the regression coefficients for the intercept, linear, quadratic, and cross-product terms, respectively (Jung et al., 2017).
The carbohydrate compositions of the raw material and steam-exploded wood (solid and liquid fractions) were determined according to the procedures of the American Society for Testing and Materials (ASTM). Thus, the carbohydrate composition was determined on the basis of the monomer content, which was measured after a two-step acid hydrolysis procedure for the fractionation of the fibers. The first step involved adding 72% H2SO4 at 30 °C for 60 min. In the second step, the reaction mixture was diluted with 4% H2SO4 and autoclaved at 121 °C for 1 h. The sugar content of this hydrolysate was then analyzed by gas chromatography (GC) following sulfuric acid hydrolysis and conversion to alditol acetates. The analysis was performed using a gas chromatograph system (YL6100 GC, Young Lin Ins. Co., Ltd. Korea) equipped with a DB-225 capillary column 15 m long with an inner diameter of 0.25 mm and a film thickness of 0.25 mm (Agilent Technologies, USA). Each sample (2 uL) was injected via a split injector (200 °C, split ratio of 30:1) into the DB-225 capillary column. The column temperature program was set as follows: The initial column temperature was 190 °C (held for 1 min), and then it was increased to 220 °C at 10 °C/min (held for 10 min) with a total run time of 17 min. The carrier gas was nitrogen (flow rate of 40 cm/s) and the detector was set at 70 eV. The standard curve for each monosaccharide, using myo-inositol as the internal standard, was determined by plotting the concentration ratio against its area ratio.
The prebiotic sugar content was estimated using the phenol-sulfuric acid colorimetric method (Dubois et al., 1956). The samples (0.6 mL) and the phenol solution (0.3mL) were placed in screw-capped tubes (13×100 mm), which were then capped and vortex-stirred. Following this, 1.5 mL of concentrated H2SO4 was added either directly against the liquid surface over 2 or 10 s or slowly down the side of each tube. The tubes were then closed, vortex-stirred for 5 s, and incubated for 15 min at 100 °C. All the tubes were allowed to cool to room temperature before the absorbance was measured at 490 nm, using distilled water as the blank, in a UVvisible spectrophotometer (HITACHI U-3000, Tokyo, Japan); the final quantification was based on a glucose calibration curve.
The probiotic strains used in this study included Bifidobacterium animalis subsp. animalis, Bifidobacterium breve, Bifidobacterium longum, Bifidobacterium bifidum, Lactobacillus casei, Lactobacillus acidophilus, Lactobacillus delbrueckii subsp. bulgaricus, Enterococcus faecium, Enterococcus faecalis, Bacillus subtilis, Bacillus coagulans, Lactococcus gasseri, and Streptococcus salivarius subsp. thermophilus. The probiotic activity of the strains in the presence of the prebiotic sugars extracted from P. densiflora, at the optimal two-stage process conditions, was determined by measuring the optical density using a UV-visible spectrophotometer (HITACHI U-3000, Tokyo, Japan). In this method, the change in OD at 600 nm was measured, which indicated turbidity and, thus, growth of the probiotic microorganisms. The number of cells required for an initial OD value of 0.1 at 600 nm were harvested and inoculated in de Man, Rogosa, and Sharpe (MRS) medium without sugar. The positive control was incubated in MRS medium, and the samples were incubated at 37 °C for 48 h; the OD was measured at 600 nm after mixing for 15 seconds.
Regression analysis and ANOVA were performed using Design-Expert, version 10 to assess the combined effect of the variables on the responses according to the full quadratic polynomial model with a confidence level of 95% (p = 0.05). All the experiments were performed in triplicate, and the mean values were reported as responses in the RSM model.
3. RESULTS and DISCUSSION
The carbohydrate composition of the solid fraction obtained after each steam explosion treatment is shown in Fig. 1, which also shows the expected decrease in the carbohydrate content of the steam-exploded P. densiflora solid fraction as the severity factor increased. This result was mainly observed due to the removal of hemicelluloses, as some authors have shown previously (Martin-Sampedro et al., 2014). The proportion of the sugars that were not detected in the solid fraction after treatment at a severity factor of 4.12-4.71 depended on the type of the hemicellulosic sugar (i,e., arabinose, xylose, mannose, or galactose). Generally, hemicellulose undergoes considerable decomposition at temperatures ranging from 200 °C to 300 °C, and some small phenolic fragments are released from lignin within the temperature range of steam explosion (Tumuluru et al., 2011). The carbohydrate composition of the liquid fraction obtained after water extraction of the steamexploded P. densiflora is shown in Fig. 1. The carbohydrate content initially increased with the severity factor (Ro) for steam explosion, and then slowly decreased. This trend can be ascribed to a balance between depolymerization of the oligomeric sugars and secondary degradation of the monomeric products. The increase in the content of arabinose, xylose, and galactose after steam explosion, albeit still significant, was lower than that for mannose and glucose. Arabinose, which is the least stable of the oligomeric sugars, was completely depolymerized during steam explosion, and in some cases, the yield of arabinose actually decreased because of secondary degradation.
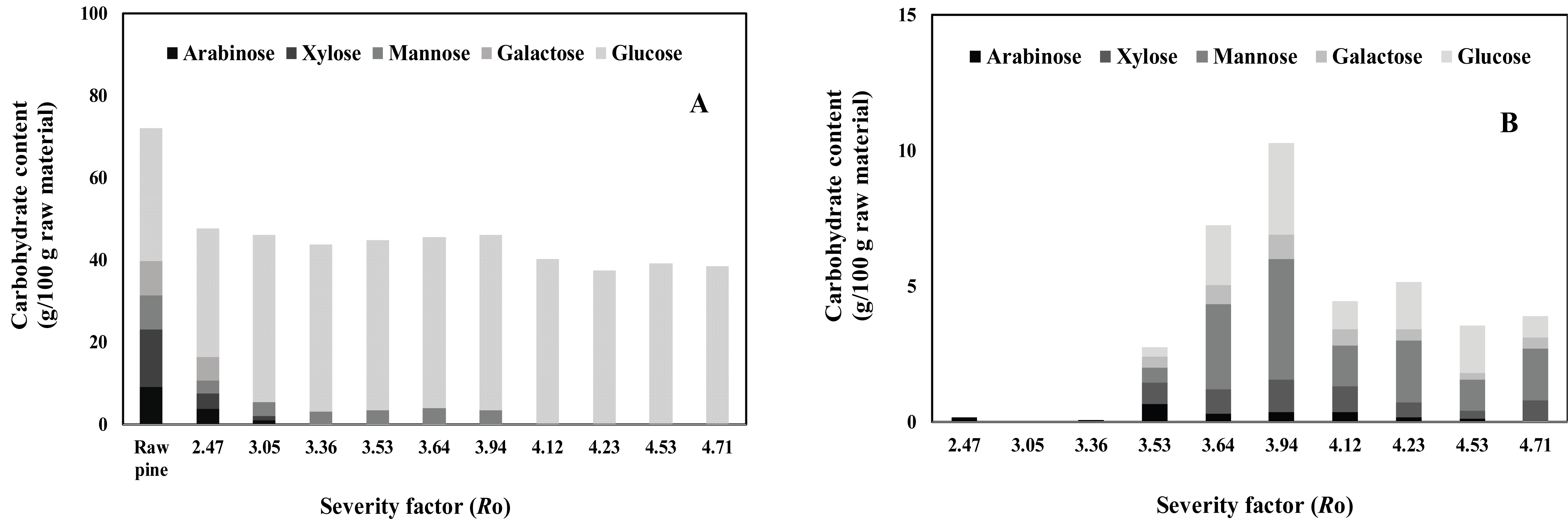
Cellulose and hemicellulose are among the polysaccharides that can undergo considerable changes at a critical temperature of 120 °C. Hemicellulose is more sensitive to temperature and is easier to degrade than cellulose. During steam explosion, cellulose and hemicellulose were degraded, and polysaccharides with low molecular weights were generated from the hemicellulose (Zhang et al., 2006).
To investigate the influence of different steam explosion conditions on the prebiotic sugar content of P. densiflora, steam explosion was performed using the following different severity factor (Ro) values: 2.47, 3.05, 3.36, 3.53, 3.64, 3.94, 4.12, 4.23, 4.53, and 4.71 (Fig. 2A). The extraction temperature, extraction time, and the ratio of water to the material were fixed at 60 °C, 60 min, and 30, respectively. As shown in Fig. 2A, the maximum prebiotic sugar content was observed when the severity factor (Ro) was 3.94. Therefore, severity factor (Ro) values in the range of 3.64 to 4.12 were evaluated in the present study.
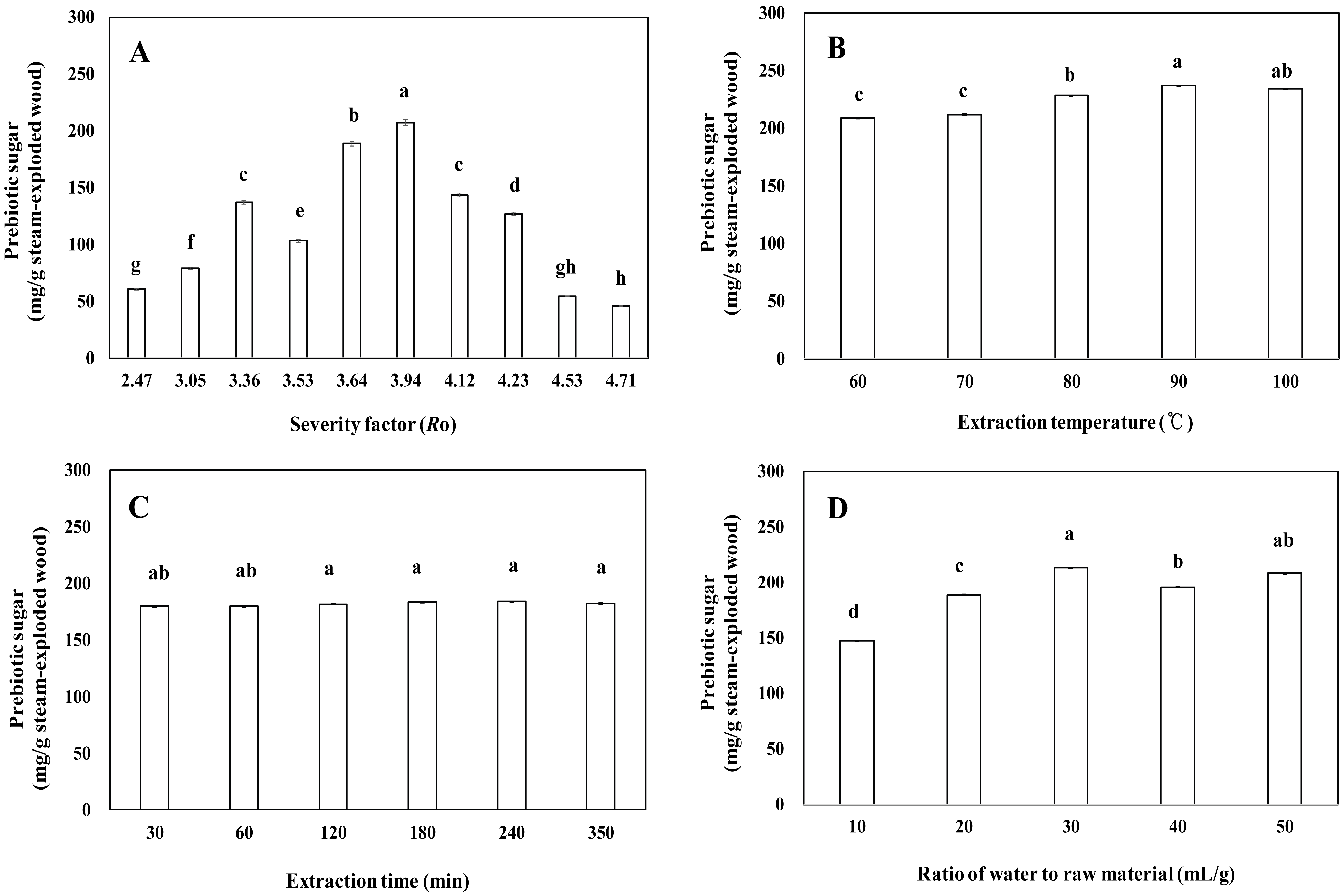
The extraction temperature is one of the most important variables affecting the extraction of prebiotic sugars; an optimum extraction temperature is required to assure the maximum prebiotic sugar extraction by water extraction. Other factors were set as follows: the severity factor (Ro) at 3.94, the extraction time at 60 min, and the ratio of water to material at 30. From the data, we observed that the prebiotic sugar content increased and reached a maximum at 90 °C, after which it declined slightly (Fig. 2B). Therefore, extraction temperatures ranging from 80 °C to 100 °C were investigated in the present work.
The different extraction times were set at 30, 60, 120, 180, 240, and 300 min to evaluate the effect of the extraction time on the prebiotic sugar content, setting the other extraction conditions as follows: a severity factor of 3.94, an extraction temperature of 90 °C, and a ratio of water to material of 30 (Fig. 2C). Fig. 2C shows that the prebiotic sugar content was not correlated with an increase in extraction time, with the prebiotic sugar content being 181.7 mg/g at an extraction time of 120 min. Although the prebiotic sugar content was high when the extraction time was 180 min, increasing the extraction time would increase the cost of the extraction process from an industrial point of view. For this reason, an extraction time of 120 min was used in the present study.
The effect of changing the ratio of water to material (10, 20, 30, 40, and 50) on the prebiotic sugar content was also investigated, while maintaining the other three factors as follows: the severity factor (Ro) at 3.94, the water extraction temperature at 90 °C, and the extraction time at 120 min. Fig. 2D shows that the ratio of water to material had a significant effect on the prebiotic sugar content. When the ratio was increased from 10 to 30, the prebiotic sugar content increased significantly. However, the data also suggests that a higher ratio of water to material (40 and 50) may lead to a decrease in the prebiotic sugar content. Thus, a water to material ratio of 30-50 was used for further investigation. In summary, we set the following range of parameters for the response surface methodology experiments on the basis of these single-parameter studies: a steam explosion severity factor (Ro) of 3.94-4.12, a water extraction temperature ranging from 80 °C to 100 °C, and a ratio of water to material of 30 ‒ 50 (mL/g).
A box-Behnken design (BBD) is more efficient for conducting experiments compared with traditional methods, since it simplifies the complexity of the experimental trials needed to evaluate multiple variables and their interactions.
The main goal of the steam explosion step is to modify the structure of the lignocellulosic biomass to increase the yield of the main component. The effectiveness of the steam explosion method was evaluated by performing prebiotic sugar tests on the liquid fraction extracted from P. densiflora using RSM to optimize the three independent variables: severity factor, water extraction temperature, and ratio of water to material. The objective of the experimental design was to optimize the steam explosion (severity factor (Ro)) and water extraction conditions (extraction temperature (°C) and ratio of water to material (mL/g)) in order to maximize the prebiotic sugar content.
The values of the response prebiotic sugar content (Y) under the different experimental combinations are shown in Table 3. The prebiotic sugar content varied considerably depending on the extraction conditions. The actual prebiotic sugar content ranged from 142.7 to 330 mg/g, whereas the predicted value ranged from 159.8 to 314.2 mg/g.
Among the 17 experiments (actual values), experiment 17 (severity factor of 3.94, extraction temperature of 90 °C, and ratio water to raw material of 30) yielded the highest prebiotic sugar content (330.0 mg/g dry steam-exploded wood), whereas experiment 9 (severity factor of 3.64, extraction temperature of 90 °C, and ratio water to raw material of 30) afforded the lowest prebiotic sugar content (142.7 mg/g dry steam-exploded wood).
The response surface shown in Fig. 3A-3C reveals the effect of extraction time and extraction temperature on the prebiotic sugar content.
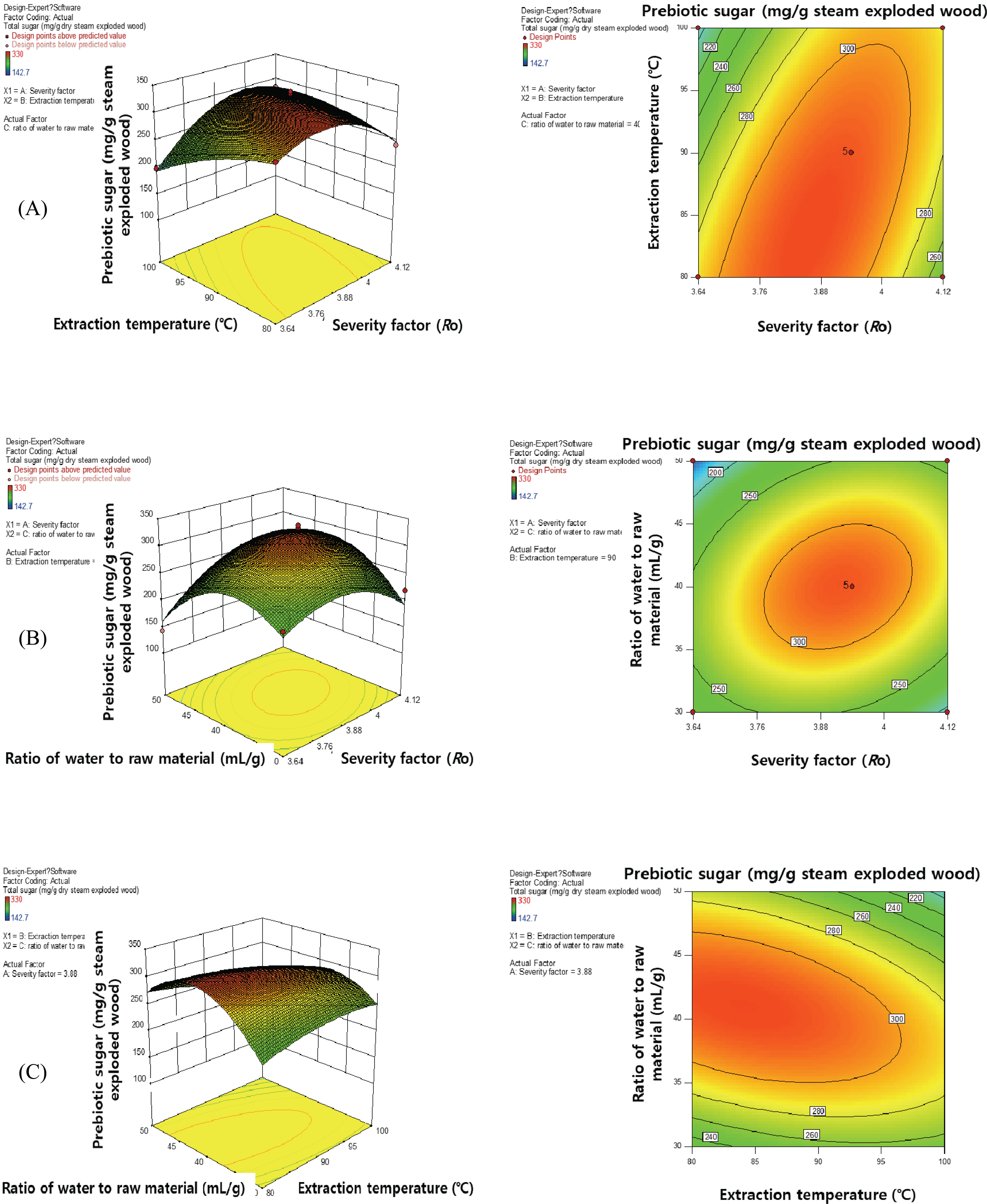
Three factors at three levels of BBD were used in this study to investigate the influence of operational variables such as severity factor (Ro = 3.64 ‒ 4.12), extraction temperature (80 °C ‒ 100 °C), and the ratio of water to material (30 ‒ 50) on the prebiotic sugar content from P. densiflora. From the model developed, a three-dimensional response surface and contour plots were constructed to illustrate the main and interactive effects of the independent variables on the response variable. These graphs, which are easy to understand and draw, were obtained by maintaining one factor constant (in turn at its central level), while varying the other two factors to understand the main and interactive effects of the constant factor on the dependent variables. They can also be used to determine the optimum conditions. As shown in Fig. 3B, the prebiotic sugar content increased with increasing the severity factor up to 3.86, resulting in a maximum prebiotic sugar content. However, further increase in the severity factor resulted in a decrease in the prebiotic sugar content.
An analysis of variance (ANOVA) was performed to evaluate the predictive model and the variables. The analysis of variance was used to determine the coefficient of determination, and the significance of the linear, quadratic, and interaction effects of the independent variables on the response.
The significance of regression coefficient was determined using the p value in Table 4. The corresponding variables become more effective as the p value becomes smaller. Moreover, the p value can be used to evaluate the interaction strength between the independent factors (Muralidhar et al., 2001).As shown for the prebiotic sugar content (Y), it can be seen that the value of the linear coefficient (X1, X3), the quadratic term coefficient (X12 and X22), and the cross-product coefficient (X2 × X3) were significant, with very small p values (p < 0.05). On the other hand, the values of other coefficients (X2, X32, X1 × X2, and X1 × X3) were found to be insignificant (p > 0.05). We observed that the variable with the greatest effect on the prebiotic sugar content was the linear coefficient of the ratio of water to material (X3). The R2 and adjusted R2 values were 0.9130 and 0.8012 for prebiotic sugar content, respectively, which indicates that the highest variation (80%) of the prebiotic sugar content could be predicted by the models.
Applying the methodology to the desired function, the optimum values for various parameters were obtained as follows: 3.86 for the severity factor (Ro), 89.66 °C for the extraction temperature, and 39.20 for the ratio of water to material (mL/g).
Under these optimum conditions, the prebiotic sugar content was determined to be 332.45 mg/g. In addition, the prebiotic activity (Bifidobacterium animalis subsp. animalis, Bifidobacterium breve, Bifidobacterium longum, Bifidobacterium bifidum, Lactobacillus casei, Lactobacillus acidophilus, Lactobacillus delbrueckii subsp. bulgaricus, Enterococcus faecium, Enterococcus faecalis, Bacillus subtilis, Bacillus coagulans, Lactococcus gasseri, and Streptococcus salivarius subsp. thermophilus) was evaluated using the P. densiflora extract prepared under optimal conditions (Fig. 4). The prebiotic activities were found to be more than 50% (excluding Bifidobacterium animalis subsp. animalis, Lactobacillus delbrueckii subsp. bulgaricus, and Bacillus coagulans). It is worth noting that the activities of Enterococcus faecium and Enterococcus faecalis were higher than 90%. These data suggest that the extract obtained from P. densiflora using the two-stage process can be considered to be a prebiotic sugar-rich extract. Recently, it has been shown that the addition of probiotics containing Enterococcus faecium to broiler diets increased the length of the jejunal and ileal villi. Moreover, increased intestinal villi heights were reported after the addition of Bacillus subtilis in combination with prebiotics.
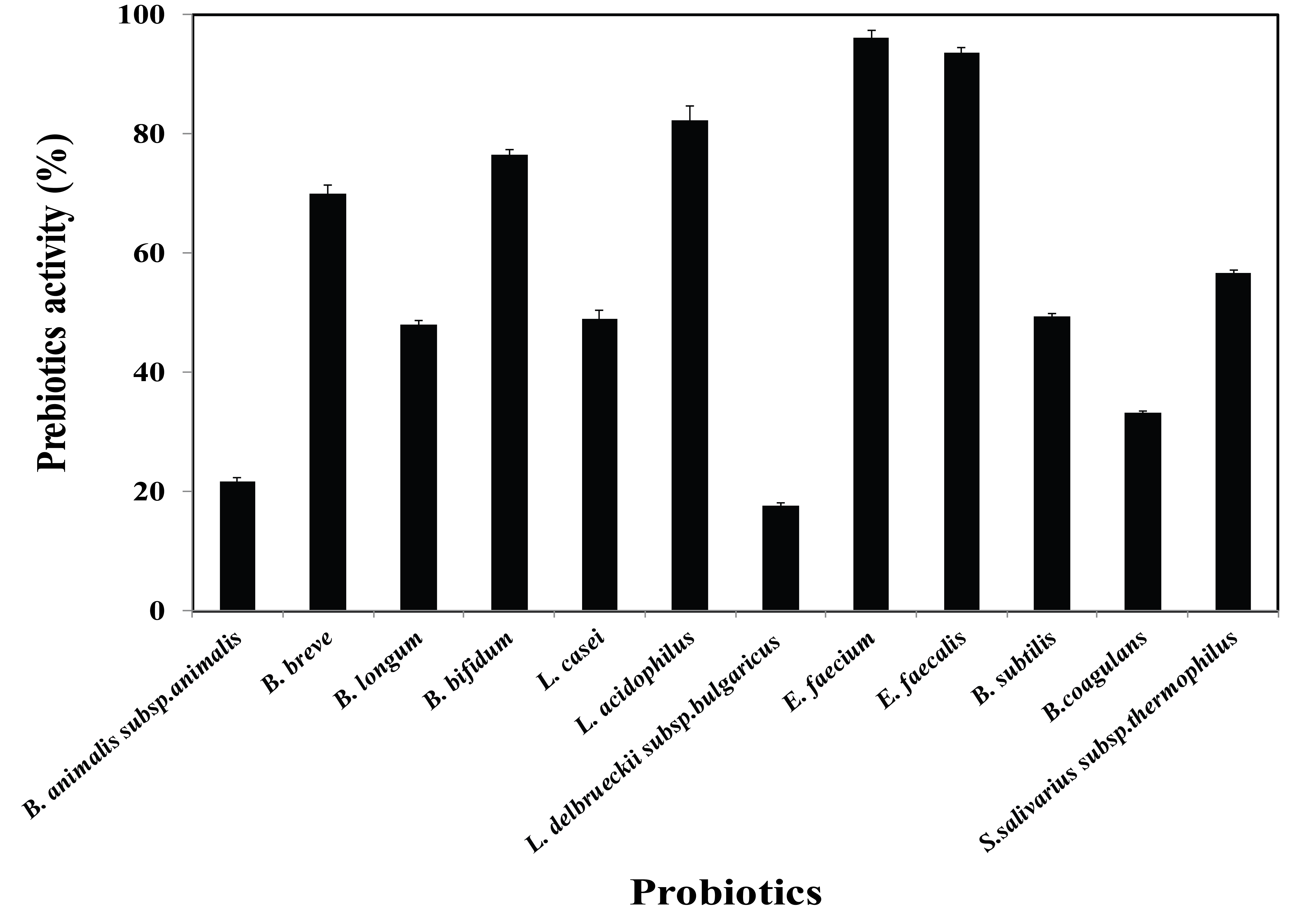
4. CONCLUSION
In this study, a two-stage process including steam explosion and water extraction was performed to efficiently release prebiotic sugars from P. densiflora. The simultaneous optimization of the process parameters was carried out by using BBD and RSM, resulting in an optimal severity factor (Ro) of 3.64 ‒ 4.12, a water extraction temperature between 80 °C and 90 °C, and a ratio of water to material of 30 ‒ 50 (mL/g). The data showed that both severity factor (Ro) and the ratio of water to material (w/v) had a significant effect on the prebiotic sugar content. Under the optimal conditions of Ro = 3.86, a water extraction temperature of 89.66 °C, and a ratio of water to material of 39.20 (w/v), the prebiotic sugar content was 332.45 mg/g. Enterococcus spp. strains showed good sensitivity to the P. densiflora prebiotic-rich extract. In conclusion, this P. densiflora prebiotic-rich extract could be considered in the future as an alternative source of prebiotics.