1. INTRODUCTION
Indonesia, a tropical country, is known for its incredibly diverse wood species—each exhibiting its unique growth behavior and distinct characteristics. Indonesian wood provides significant ecological, social, and economic benefits. Harnessing tropical forests for the benefit of society requires an understanding of tree growth to inform strategy development and ensure sustainable forest management in the future. Information regarding the existence of forest stands is closely related to tree growth, mortality, structure, and species composition. Accurate growth and yield conditions support forest management optimization (Nascimento et al., 2014; Rosli and Gang, 2013). Red jabon [Anthocephalus macrophyllus (Roxb.) Havil], a native species, is widely sought after by local communities and wood industry stakeholders due to its rapid growth, providing an alternative resource to meet local and national wood demands. Red jabon grows rapidly in diameter, reaching 5.05 cm annually (Mpapa, 2022). Therefore, extensive plantation forest development is necessary to meet these requirements. Knowledge of tree growth is required for red jabon plantation forest development and its wood production utilization. Growth involves the formation, development, and differentiation of cells and organs. At the cellular level, wood formation involves cell division, cell enlargement, cell wall thickening, and lignification (Brack, 2001; Fromm, 2013; Plomion et al., 2001). The growth state of tropical trees, such as red jabon, is usually measured based on dimensional changes in trunk circumference or diameter. Diameter is one of the tree measurements most often used as a growth parameter. Information on diameter increase can help model tree growth (Adame et al., 2014; Bowman et al., 2013; Roik et al., 2018).
Cambial tissue activities in trees cause the trunk to grow larger. Changes in stem diameter per unit of time can provide information on growth conditions. Diameter is one of the most frequently used tree dimensions as a growth parameter (Scolforo et al., 2017). An increase in stem diameter indicates wood formation from its constituent cells. Cells formed in the trunk exhibit complex physiological activities over a certain period. New cells resulting from cambium tissue division grow in size and develop, causing an increase in tree dimension or volume (Plomion et al., 2001). The increase in stem and root diameters will, in turn, increase nutrient and water transport and photosynthesis. This increase is critical in determining vascular cambium activity, which consists of fusiform cambium and ray cambium. The fusiform cambium forms cells parallel to the vertical direction of the trunk, whereas the ray cambium produces ray cells (transverse to the trunk; Vanclay, 1994). Cambium tissue activity is influenced by internal factors, particularly hormones, and external factors, particularly climate, such as sunlight duration (photoperiod), temperature, and rainfall. Auxin is the main growth hormone involved in cambium growth (Lachaud et al., 1999; Schrader et al., 2003; Uggla et al., 1998). The increase in cambium tissue activity, in turn, increases stem, branch, twig, and root diameters. The climate plays a crucial role in cambium growth and wood formation. Cambium zone thickness varies seasonally (Crang et al., 2018). Temperature and rainfall in a year correlate positively with cambium activity (Lee et al., 2022).
Wood mass increase in the radial direction of the stem, in combination with the pinning method, provides critical information, such as the ability to monitor wood formation precisely (Wolter, 1968). This method is extremely effective for measuring radial increases in tropical trees (Shiokura, 1989) and provides an almost perfect picture of the newly formed wood cells (Camp et al., 2017). The onset of wood formation from the onset of cambium activity is determined by the wounding method, observed in the cross-sectional and radial sections of the stem throughout the growing season (Seo et al., 2007). The depth at which the needle is inserted into the tree trunk varies, and the puncture must penetrate the cambium tissue (Kuroda and Shimaji, 1984; Schmitt et al., 2004; Seo et al., 2007; Yoshimura and Hayashi, 1981). The newly formed cells can be observed in cross and radial sections, or both (Larson, 1969). The pinning method has mainly been applied to trees in temperate regions (Bäucker et al., 1998; Kuroda and Shimaji, 1984; Mil et al., 2017; Nobuchi et al., 1995; Yoshimura and Hayashi, 1981). The pinning concept is based on cambium characteristics, which are highly responsive to external disturbances. When wounded, the cambium exhibits two different responses: stopping the formation of new cells and remaining active. This response enables wood formation monitoring over a certain period and an elucidation of the dynamics of tree growth (Larson, 1969). The response of cambium to wounds varies and depends on the tree species, shape and size of the wound, season, growth conditions, and needle size (Lachaud et al., 1999).
In Indonesia, studies have not been widely conducted on wood formation in fast-growing trees, such as red jabon, using the pinning method. Unlike trees in subtropical areas, where information is available for a long time (Bauch and Dunisch, 2000; Bäucker et al., 1998; Grotta, 2002; Mäkinen et al., 2008; Mil et al., 2017; Vieira et al., 2014), information on the dynamics of red jabon wood formation, affected by climatic factors, such as rainfall and temperature, remains extremely limited. In this study, we aimed to examine wood formation in red jabon, a potential local wood species, through anatomical observations after pinning. We also examined the effects of climate (rainfall and temperature) on wood formation. The information obtained in this study provides valuable insights and a precise perspective on wood formation and the application of silviculture (Marbun et al., 2019) to help improve growth, wood properties, and wood quality (Fadwati et al., 2023; Hidayati et al., 2019) using the pinning method.
2. MATERIALS and METHODS
Red jabon stands were planted in 2009 in the Banggai plantation forest in Bunga Village, North Luwuk District, Banggai Regency, Central Sulawesi Province (00°52’26.23” South Latitude and 122°54’04.19” East Longitude), altitude 304 m above sea level [Fig. 1(a)]. Three red jabon trees were used for wood formation observations [Fig. 1(b)]. Based on climate data from the Luwuk station of the Meteorology and Geophysics Agency, during 2021, the amount of rainfall was 1,194.6 mm and the average temperature was 28.19°C (Table 1). During the 10 years (2012–2021) of the study period, the amount of rainfall ranged from 1,004.4 to 1,848 mm, and the average temperature ranged from 27.7°C–28.7°C. The climate in Banggai Regency shows a varying trend every year [Fig. 1(c)].
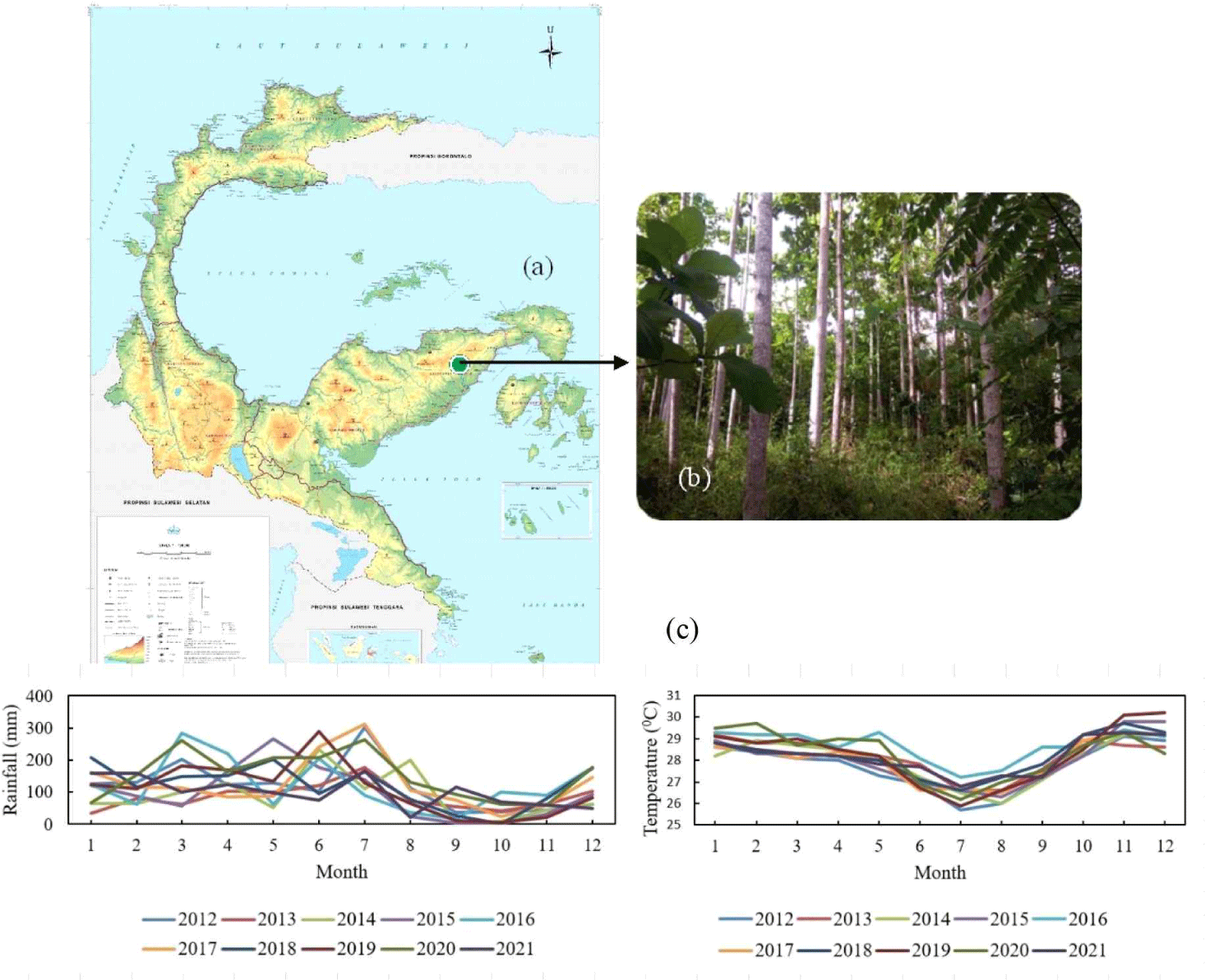
The red jabon tree trunk at breast height [Fig. 2(a)] was wounded using a pinning tool equipped with a 2 mm diameter needle [Fig. 2(b)] and inserted slowly by pinning perpendicular to the bark surface to the sapwood near the cambium [Fig. 2(c)]. Wounding was carried out periodically for 360 d in 2021 at 30 (A), 60 (B), 90 (C), 120 (D), 150 (E), 180 (F), 210 (G), 240 (H), 270 (I), 330 (K), and 360 d (L). The pinning method is useful for studying wood formation (Wolter, 1968). The trunk was pinned using a needle (Kuroda and Shimaji, 1984; Yoshimura and Hayashi, 1981).
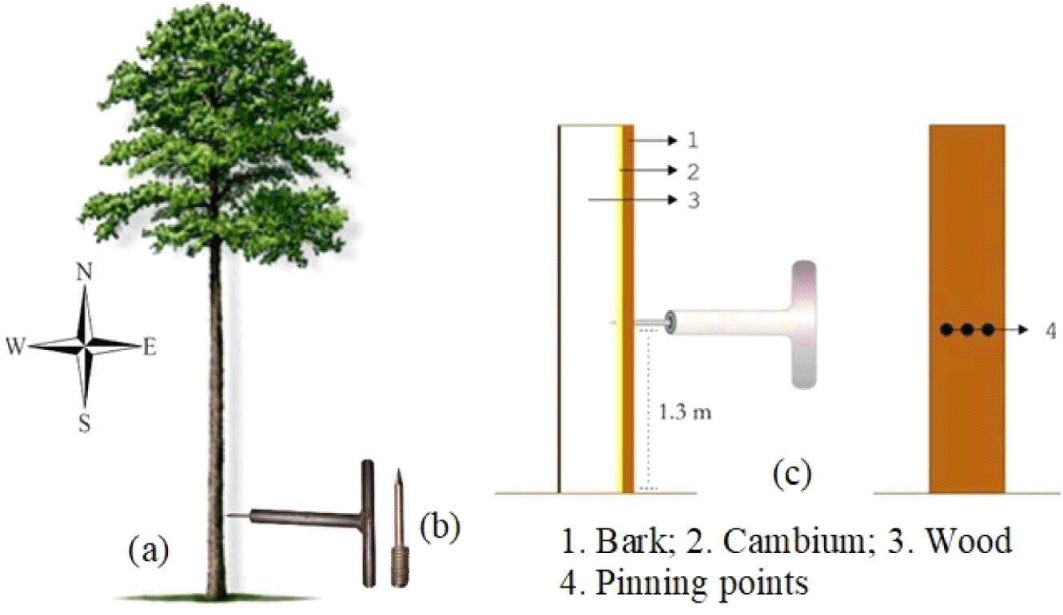
The red jabon tree sample was cut based on the criteria for a healthy tree, i.e., a disk with a thickness of ± 5 cm was sampled [Fig. 3(a)] and then cut into pieces to be used as a test sample unit [Fig. 3(b)]. Trees were sampled according to the A–L periods after pinning to retain pinning marks. The test sample was sanded, ensuring its surface was smooth and revealed pinning marks on its cross-section. The changes in anatomical structure were observed microscopically using wood tissue sections of 20–25 μm thickness, prepared using following standard procedures (Wheeler et al., 1989) on a microtome (HistoCore Biocut; Leica Biosystems, Deer Park, IL, USA) in the anatomy laboratory of the Bogor Sustainable Forest Management Instrument Standardization Center [Fig. 3(c)]. Microscopic observations were performed under an Olympus BX51 microscope, and images were recorded using a DP70 digital camera (International Equipment Trading, Mundelein, IL, USA).
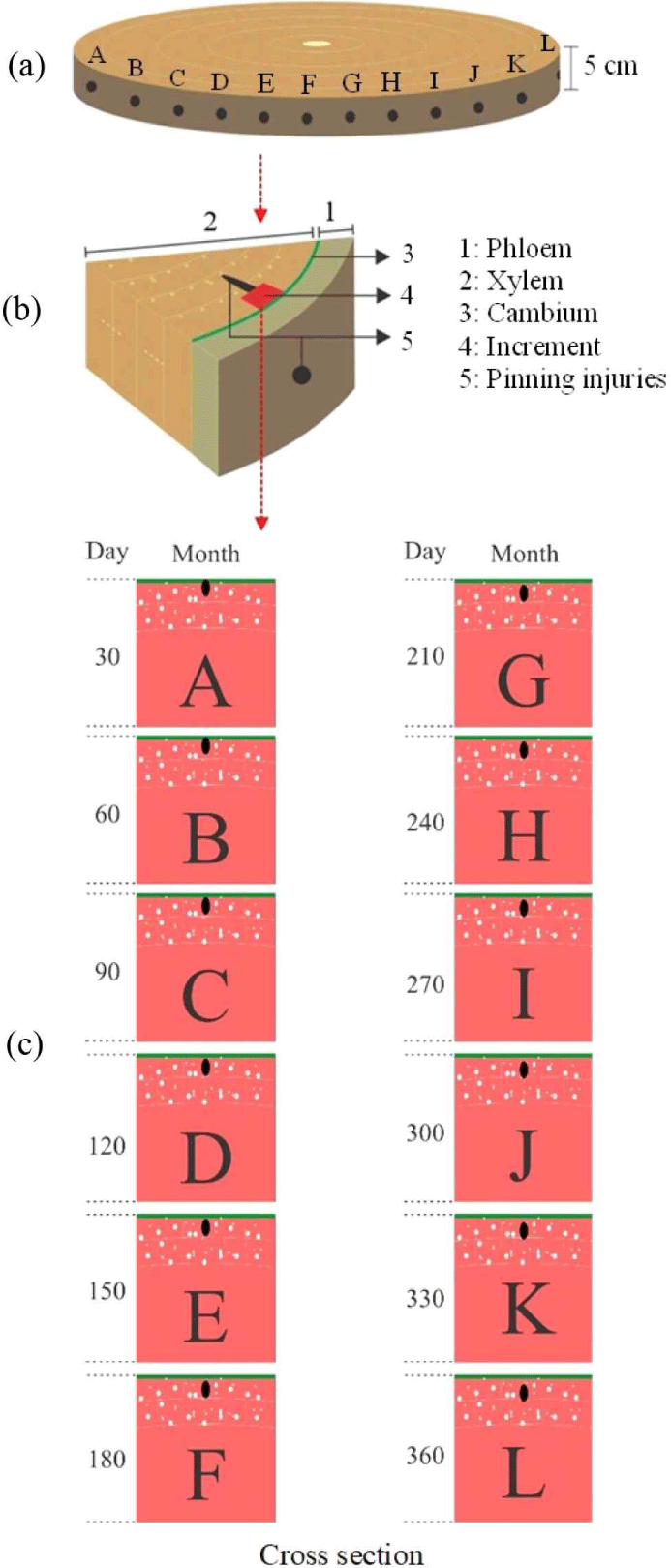
We estimated the time required for wound healing caused by the pinning of red jabon trees based on observations made of samples A–L after pinning. Hardwood wound healing can be observed at a certain time (Wood, 1980). The width of the newly formed wood in red jabon trees was measured using the ImageJ software to observe new cell additions.
3. RESULTS and DISCUSSION
Microscopically, at all observation times from A to L after pinning, wounds were visible on the cells (Fig. 4). During periods A and B, the canal remained open and slowly closed from periods C to L. There was a visible cell bending that looked like a line on the side of the wound canal. The presence of wounds and cell bending on the sides provides a unique sign used to determine the annual growth ring boundaries in red jabon wood, which is known to be a fast-growing type with unclear growth rings. The formation of new cells during periods A and B was concentrated on both sides of the canal. During periods C–L, the formation of new cells was observed in the radial direction of the red jabon trunk. Tree injury during tree growth is believed to give rise to callus tissue (a group of parenchymal cells) that develops on the wound surface. The callus forms first in response to injury by the cambium and sapwood cells.
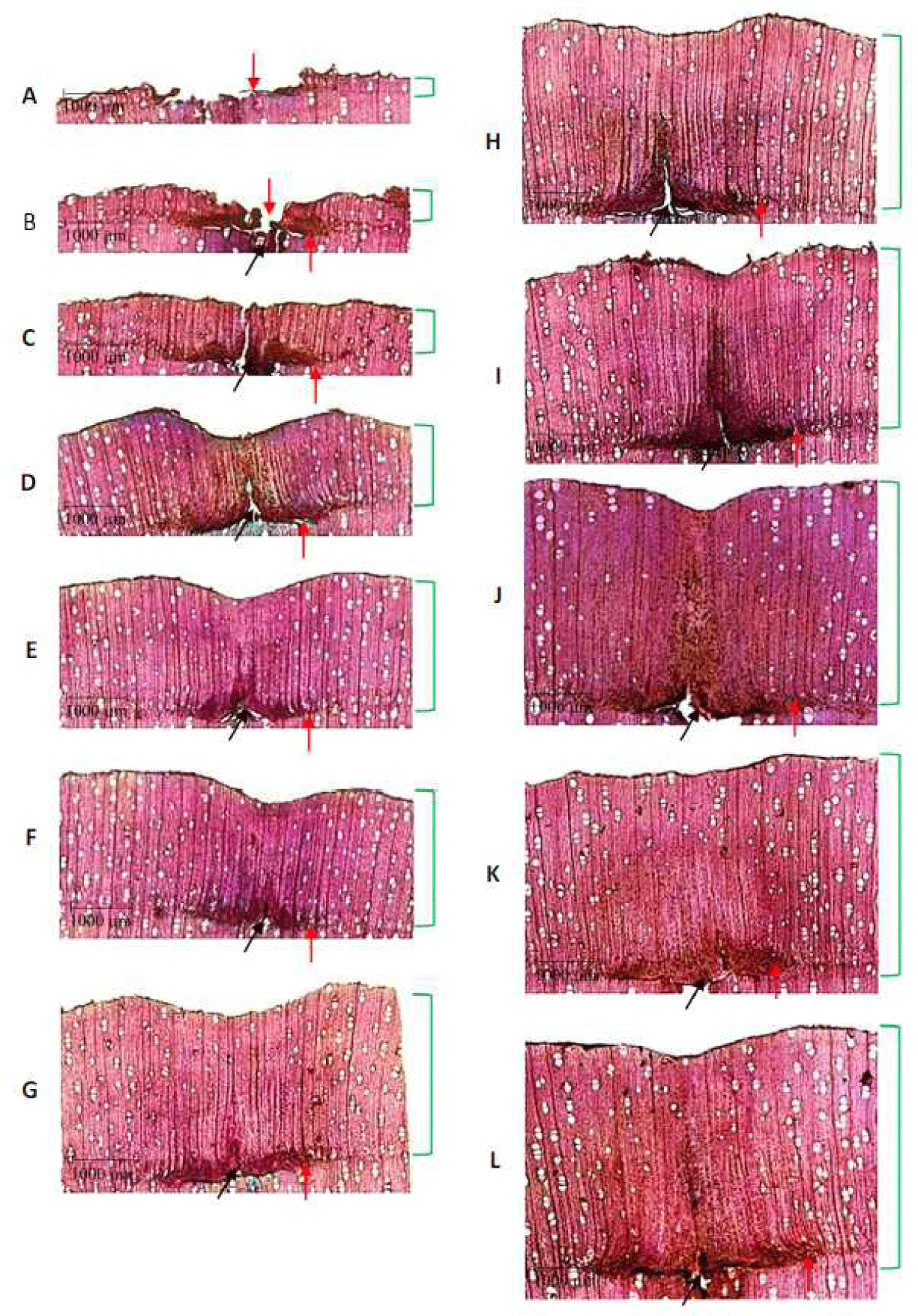
When the callus develops and grows, it quickly and efficiently covers the open tissue. The rate of wound healing varies significantly among tree species. The recovery speed is also impacted by environmental conditions and tree health (Purcell, 2020; Stobbe et al., 2002). During the observation period, a zone was observed around the wound because of pinning. Cells near the cambium died. Moreover, cells located a few micrometers from the side of the puncture canal appeared healthy, which indicates compartmentalization and cambium necrosis. Both caused the formation of a zone around the wound. Compartmentation occurs because of injured wood cells, and a boundary between the two parts is visible. Cambial necrosis is a form of vascular cambium that causes death (Dickinson and Johnson, 2004; Purcell, 2020; Shigo and Shortle, 1984). It is becoming increasingly clear that pinning can accurately determine the beginning of red jabon wood formation, as seen in the anatomical cross-section throughout the observation period. According to Gričar et al. (2007), wood formation begins by distinguishing between necrotic remains of cambium cells and xylem cells that extend radially. New cells, such as vessels, fibers, parenchyma, and ray cells were formed during the observation period. Vessel distribution exhibited a circular pattern, whereas vessel arrangement was solitary, with vessels commonly distributed in radial multiples of ≥ 4. The axial parenchyma was diffuse, fiber cells were clear, ray cells appeared as bands in rows, whereas growth ring boundaries were either indistinct or absent.
During the observation period, red jabon wood formation was visible in the cross-section of the trunk, which was characterized by the formation of new cells after pinning (Fig. 4). The new cells differed significantly in terms of their number and dimensions. The results obtained in this study confirmed that the pinning method could be used to monitor wood formation in each sampling period, particularly for fast-growing species, such as red jabon. Wood cells continue to expand in the radial direction of the trunk; therefore, the wood mass continues to increase over time, resulting in a larger red jabon trunk. The width of the newly formed xylem (wood) after pinning exhibited an increasing trend (Table 1).
The formation of red jabon wood is a complex process involving metabolic activity in the roots, stems, and crowns of trees. Cambium activity is central to this process (Chaffey, 2002). Cambium is a stem cell system that participates in wood cell production and substantially regulates xylem development dynamics and cell differentiation over time to support tree body formation (Deslauriers et al., 2003; Mizrachi and Myburg, 2016). The width of the red jabon wood formation in each observation period was also determined by the intra-annual cambium activity (Hidayat et al., 2017; Park et al., 2015).
The mass of the red jabon wood in the radial direction of the stems continued to increase throughout the observation period. All sample trees exhibited xylem widths greater than 2 cm (after conversion). This condition may be related to both internal and external factors, such as the number of cells produced by the vascular cambium and temperature and soil conditions at the research location (Martel et al., 2008). In addition, the biological process responsible for the formation of red jabon wood is correlated with the components of genetic transcription and tree metabolism. The population of stem cells in the meristematic tissue at different locations in the plant body provides a dynamic growth potential. In the lateral position, the presence of a secondary meristem contributed to stem thickening. Stem cells from the vascular cambium of the secondary lateral meristem produce the secondary xylem (wood; Fischer et al., 2019). Pinning causes an area of dead cells, and over time, a healthy cell area, consisting of vessel cells, fibers, parenchyma, and rays, is observed, which indicates the existence of a development stage from cambium to cell death in xylem cell life (Samuels et al., 2006). The xylem has thick cell walls and is rich in cellulose, hemicellulose, and lignin. Fiber and vessel cells, a part of the xylem, provide mechanical support and transport water and minerals. The fusiform and ray initials are morphologically different meristematic cells in the cambium. Fusiform initials (> 90% of the vascular cambium) are oriented longitudinally to the stem and undergo periclinal division to produce phloem and xylem mother cells (Fischer et al., 2019; Mauseth, 2016). Ray initials produce radially oriented cells for transport and storage (Wang et al., 2021). Cell production varies based on tree species and size (Kang Han et al., 2013; Kohyama et al., 2003; Mil et al., 2017; Nabeshima et al., 2010). Fast-growing species, such as red jabon, exhibit adequate wood mass addition within 360 days after pinning.
Results showed that rainfall during the observation period amounted to 1,194.6 mm, with a range of 21.7–166.8 mm (Table 1). The temperature was in the range of 26.8°C–29.3°C. It is estimated that rainfall and temperature at the study location support red jabon wood formation. The amount of heat received is crucial in determining tree growth and cambium activity in the trunk (Rossi et al., 2006; Schmitt et al., 2004; Seo et al., 2008). The red jabon type is estimated to be a type that can survive when rainfall is below 100 mm per year. The amount of rainfall is correlated with cambium activity (Marcati et al., 2006), influencing the physiological process in the red jabon tree trunk in the form of xylem formation to continue during the observation period.
Local temperature conditions are believed to remain within the optimum range for the growth and formation of new cells; therefore, increasing the width of the newly formed red jabon wood during the observation period. Temperature affects cambium activity and xylem differentiation in the stems (Begum et al., 2017). Temperature can also affect stem mass and radial growth (Thomas et al., 2007). However, the activity of the cambium, which produces xylem, is not always influenced by seasonal temperature variations or annual temperature trends (Rossi et al., 2008; Zhang et al., 2008). Different tree species respond differently to climate, affecting their physiological activity within the stems. According to Bauman et al. (2022), the sensitivity of trees to climate varies among species. Additionally, the relationship between climate and wood formation is specific and inconsistent over time (Gauli et al., 2022).
During the observation periods of A–L, climate dynamics (rainfall and temperature) affected red jabon wood thickness formation in one year (360 days). Climate variations from year to year influenced the quantity of the formed wood. The biological and physiological aspects that regulate cambium activity in trees are crucial. Seasonal cambium activity plays an important role in wood formation and reflects the ability of trees to adapt to their environments. In temperate and cold climates, cambium is dormant during winter and active during spring and summer. Low temperatures and decreased photoperiods in winter trigger trees to enter a dormant state (Begum et al., 2017; Rossi et al., 2012). The approach of the heating unit to the biological process of wood formation begins as soon as a certain amount of heat accumulates over time. The amount of incident heat determines the onset of cambium activity (Seo et al., 2007). Temperature and rainfall play key roles in plant growth and determine the timing of wood formation. However, the specific effects of climatic factors can vary depending on local conditions (Pumijumnong et al., 2023; Sass et al., 1995; Silvestro et al., 2023), as evident from a study by Rahman et al. (2019), who investigated cambium activity in four tropical woods of Indonesia: Acacia mangium, Eucalyptus urophylla, Neolamarkia cadamba, and Tectona grandis. The absence of or low rainfall for 3–4 months is reported to cause a pause in cambium activity. Conversely, cambium reactivation continued throughout the year in the four tree species if rainfall was continuous. In contrast to the red jabon species at the study location, xylem formation continued when rainfall was low during periods E, F, H, J, K, and L, which indicates a positive correlation with rainfall. Furthermore, Buajan et al. (2023) studied xylem production in teak trees in Mae Sariang, Mae Hong Son Province, Thailand, and discovered that the width of the xylem differentiation zone positively and significantly correlated with rainfall and temperature. The findings of this study indicate that rainfall and climate play critical roles in red jabon wood formation. These findings can be used as a foundation for similar studies in the future, either on similar or other wood types, at different locations, and under different environmental stressors.
Microscopically, pinning caused wounds on the red jabon wood cell section at sampling periods of A-L. This wound provides a unique mark on the cell sections of red jabon wood. During periods A and B, canals were created due to visible pinning marks. These canals began to close through the formation of new cells from periods C to L. Complete healing (disappearance of early signs of injury) due to pinning is estimated to occur over the next few years, where intensive observations are required. The rate of wound healing is significantly impacted by tree health and environmental conditions (Luley, 2015; Purcell, 2020; Stobbe et al., 2002). In addition, applying intensive silviculture is believed to help accelerate wound healing caused by pinning and maintain the quality of red jabon trees.
4. CONCLUSIONS
The findings of this study confirmed pinning effectiveness in monitoring red jabon wood formation dynamics during the A–L observation periods after pinning. Wounds formed by pinning throughout the observation period provided important information regarding cambium tissue activity and the formation of new cells. In addition, climate parameters, such as rainfall and temperature, supported cambium activity, influencing the thickness of wood formation during the observation period. Rainfall and temperature variations have been shown to modulate the dynamics of red jabon wood formation. These findings demonstrated that utilizing the pinning method and considering climate influences can guide effective tree growth planning strategies, while also providing opportunities for further research on red jabon and other tropical wood species.