1. INTRODUCTION
Cross-laminated timber (CLT) is an innovative and sustainable engineered wood product manufactured by gluing together multiple layers of solid-sawn timber (laminas), with the grain of each layer oriented perpendicular to the adjacent layers (Ahn et al., 2021; Lee et al., 2022; Song and Kim, 2023; Trisatya et al., 2023). This material is widely applied in walls, floors, and roofs, where it offers various advantages, such as excellent load-bearing capacity and thermal insulation (Albert and Liew, 2024; Kim et al., 2024; Song and Kim, 2023; Trisatya et al., 2023). Nonetheless, the limited supply of medium-to-heavy hardwoods, which are traditionally used in the production of CLT, has become a significant concern (Albert and Liew, 2020, 2024). To address this issue, researchers have adopted timber densification, a technology that enhances the properties of low-density timber and improves the structural performance of this engineered wood product (Gan et al., 2019; Ha et al., 2023; Wang et al., 2023).
The densification process, which dates to the early 20th century, has produced densified wood products such as Staypak, renowned for their enhanced physical and mechanical properties (Albert and Liew, 2020). A previous study demonstrated significant improvements in the physicomechanical properties (e.g., flexural strength and hardness; Dodoo et al., 2022; Pelit et al., 2018). However, in the context of construction, where the incidence of building fires has been increasing, understanding the effect of densification on the fire performance of CLT is critical (Wimmers, 2017). This is especially important to ensure that the end products meet the standard requirements, with fire safety being the major priority (Wimmers, 2017). Therefore, a comprehensive assessment of the fire performance of timber and engineered wood products, such as CLT, is essential (Gan et al., 2019). Generally, fire tests such as cone calorimeter and open burning tests are conducted according to standards to assess the fire performance of materials, where there tests evaluate flammability properties, such as the ignition time, heat release rate, and oxygen consumption (Albert and Liew, 2024). In addition, the temperature profile, or dynamic temperature variations, is also an essential factor used to characterize the thermal profile of wood during combustion as the material progresses through several stages of thermal degradation (Wen et al., 2015).
The moisture content is a vital factor that affects the ignition time and intensity of the fire (Albert and Liew, 2024). Moisture delays ignition and restricts the temperature increase during the early stages of combustion (Gan et al., 2019). After being compressed, densified wood can exhibit altered moisture dynamics, influencing its temperature reactivity (Gan et al., 2019). A previous study suggested that as the timber is compressed, the heat transfer from the hot-press plates reduces the moisture content but maintains a desirable moisture level (10%–12%; Albert and Liew, 2020). A high moisture level (> 15%) helps to inhibit fire spread but weakens the structural integrity of the timber, whereas low moisture (< 10%) might enhance heat release, making the timber prone to rupture (Albert and Liew, 2024; Dodoo et al., 2022). The density and structure of the wood are other essential factors that influence the fire performance of the timber (Albert and Liew, 2024). Densified timber can have slower combustion rates because densification compacts the internal structures in wood, prohibiting oxygen diffusion (Gan et al., 2019). Furthermore, the arrangement of wood fibers and the presence of lignin, cellulose, and hemicellulose elements influence the thermal behavior of the material, where the densification process may influence these characteristics, affecting how the material reacts to heat (Emberley et al., 2017; Wen et al., 2015; Xu et al., 2015). Understanding these factors is essential for effectively determining the thermal behavior of CLT constructed from densified timber. The temperature profile of CLT made from densified Paraserianthes falcataria, a fast-growing timber species, was investigated in this study. The intention of this research was to provide insights into how short-duration densification affects temperature fluctuations during open burning, adding useful information for a more extensive understanding of the fire performance of CLT.
2. MATERIALS and METHODS
Kiln-dried P. falcataria laminas were cut into dimensions of 20 mm (t) × 50 mm (w) × 300 mm (l) and conditioned with a relative humidity of 65% and room temperature of 25°C to maintain the equilibrium moisture content of 12%.
The laminas were compressed at various compression ratios (40%, 50%, 60%) using a hot-press machine (105°C, 6 MPa) equipped with a cooling mechanism (Fig. 1). This mechanism was implemented and improvised by referring to a previous study, which assisted in reducing the set-recovery rate (Albert and Liew, 2022).
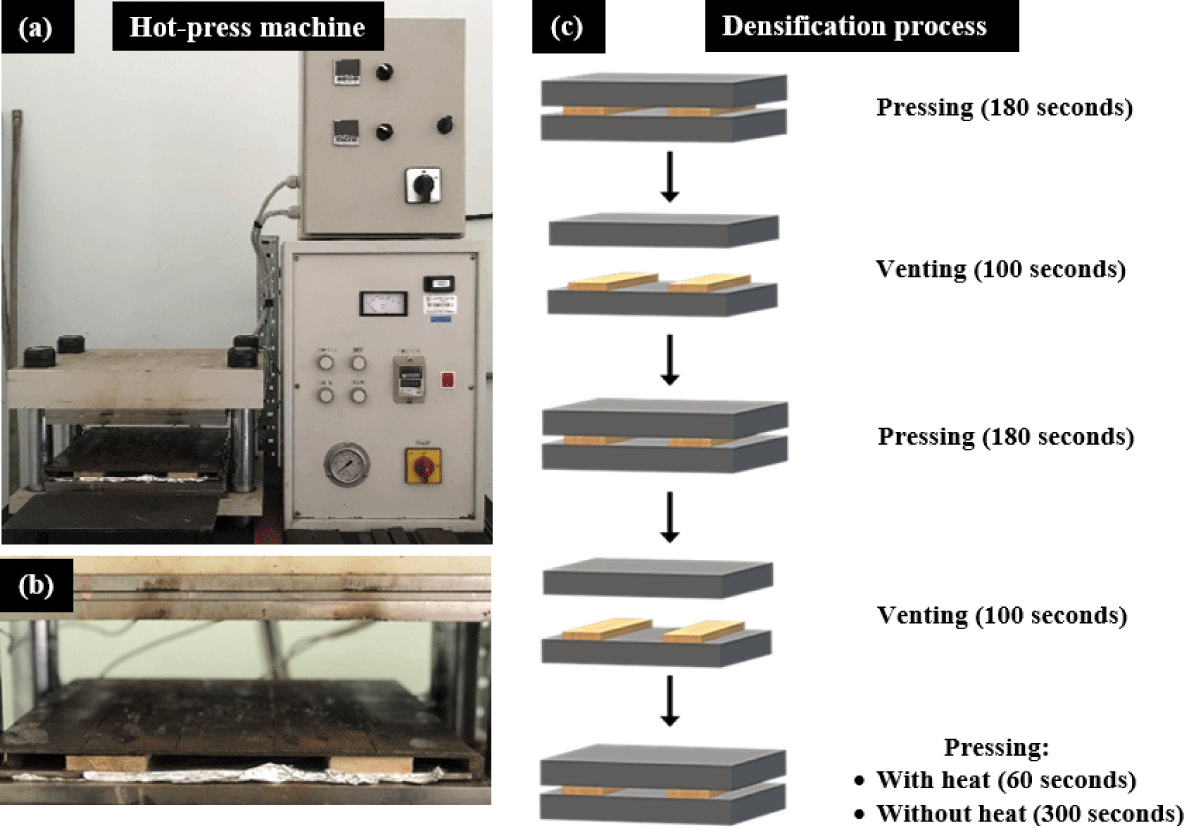
This short-duration densification approach required 900 seconds for completion. The venting phases were implemented to release the moisture trapped inside the laminas. The authors found that compressing the laminas at a temperature < 80°C during the final phase of the densification improved their dimensional stability. The compression ratio of the laminas was calculated according to Equation (1):
Where CR is the compression ratio (%), ti is the initial thickness (mm), and tf is the final thickness (mm). The final thicknesses of different compression ratios are illustrated in Table 1 and Fig. 2.
Parameter | Compression ratios (CRs; %) | Initial thickness (mm) | Final thickness (mm) | Density (kg/m3) |
---|---|---|---|---|
Control | Control | 20 | N/A | 300.60 |
40CR | 40 | 20 | 12 | 512.87 |
50CR | 50 | 20 | 10 | 614.70 |
60CR | 60 | 20 | 8 | 689.62 |
The densified laminas were edge- and face-glued using polyvinyl acetate with a constant glue spread of 400 g/m2 to form three-layered CLTs (Fig. 3) with dimensions of 60/36/30/24 mm (t) × 300 mm (w) × 300 mm (l).
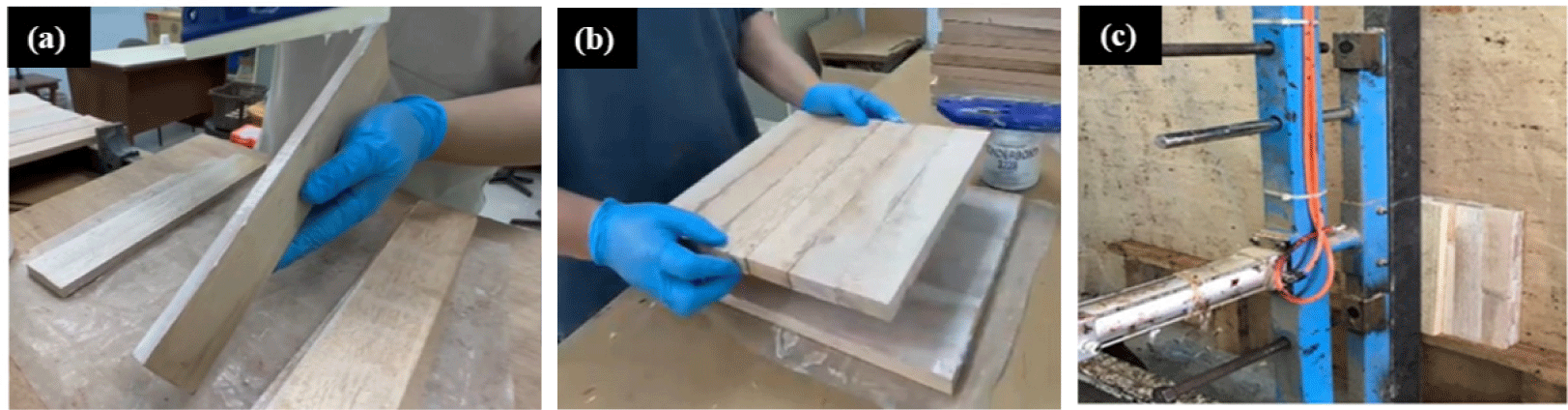
The test was performed by referring to the NIST Voluntary Product Standard PS 1-09 (Locke and Gallagher, 2010). In total, three test pieces (moisture content: 10%–12%), with dimensions of 60/36/30/24mm (t) × 146 mm (w) × 224 mm (l), were prepared from each parameter. The experimental setup is illustrated in Figs. 4 and 5, where the height of the blue flame (850°C–900°C) was set to 38.1 mm. Eight holes with a 40 mm distance from each other were drilled to insert Type-K thermocouples, which were connected to a data logger for dynamic temperature measurements. The placement configuration was set to avoid lining up multiple thermocouples that could influence the thermal properties of the test pieces (e.g., charring rate). Each test was performed for 120 minutes.
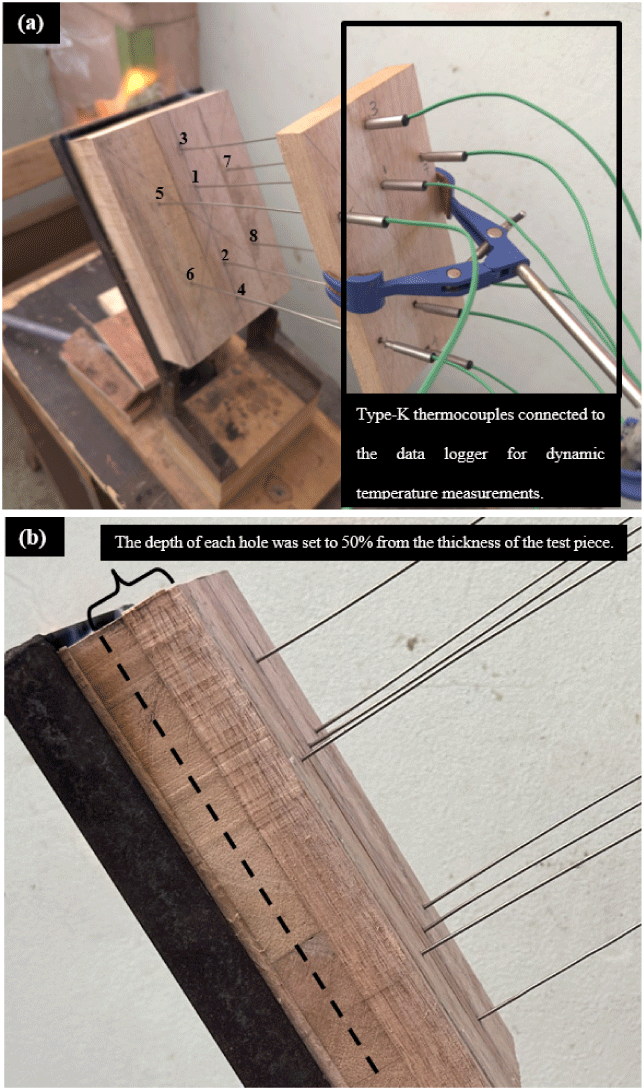
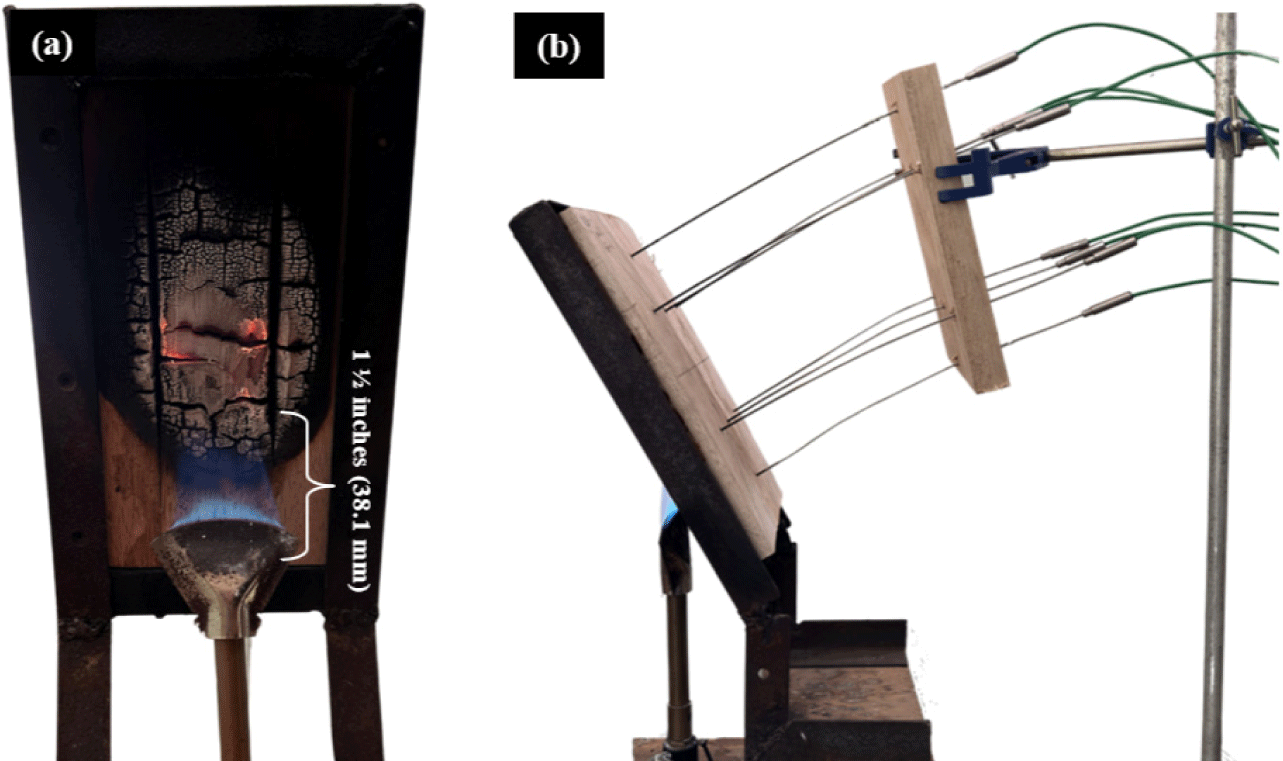
The front and side images of the test pieces were analyzed (Fig. 6) using image analysis software (ImageJ) to determine the charred area and depth.
The charring rate was calculated according to Equation (2), as follows:
Where β0 is the charring rate (mm/min), Dchar is the char depth (mm), and t is the duration of exposure (min).
3. RESULTS and DISCUSSION
The control (Fig. 7) obtained the highest charring rate (0.2186 mm/min), whereas 50CR had the lowest value (0.1717 mm/min). Statistical analysis indicated that there was no significant difference between the control and 40CR, whereas 50CR and 60CR were significantly different from the control. These outcomes suggest that densifying the laminas at 50% significantly delayed the charring rate of the CLT. However, further increasing the compression ratio to 60% did not enhance fire resistance. These results indicate that higher compression ratios produce a protective char layer that restricts the charring rate, improving the fire resistance of CLT (Gan et al., 2019). The thickness recovery of the densified lamina layers (Fig. 8) throughout the test could be a fire-resistance mechanism, as the expansion occurred concurrently with the creation of the char layer.
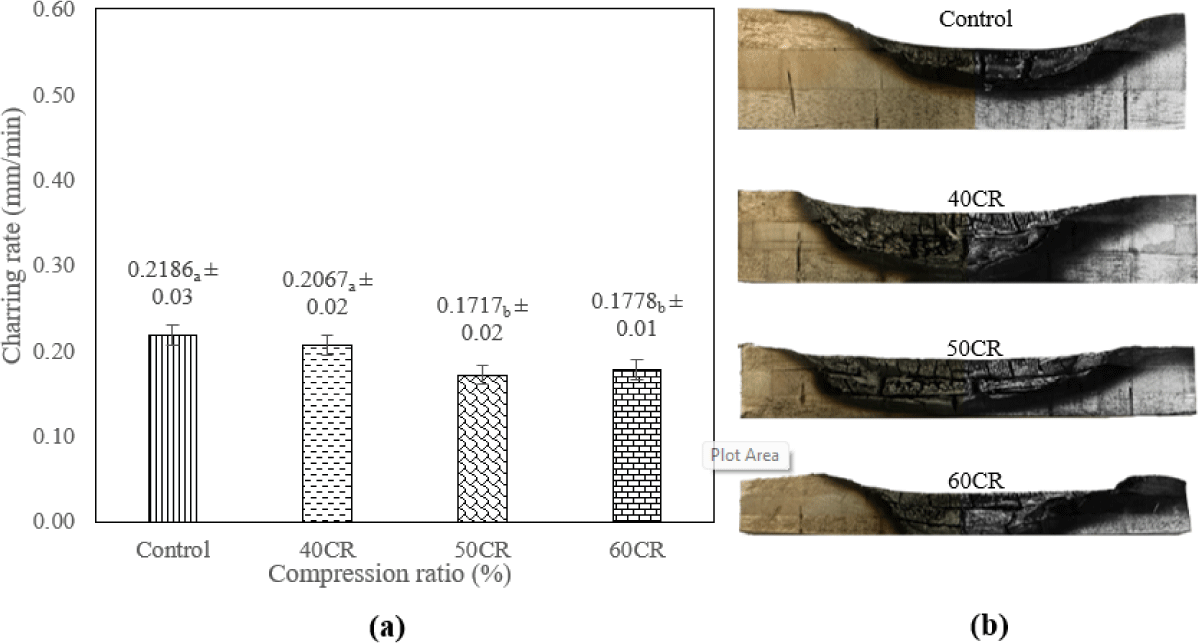
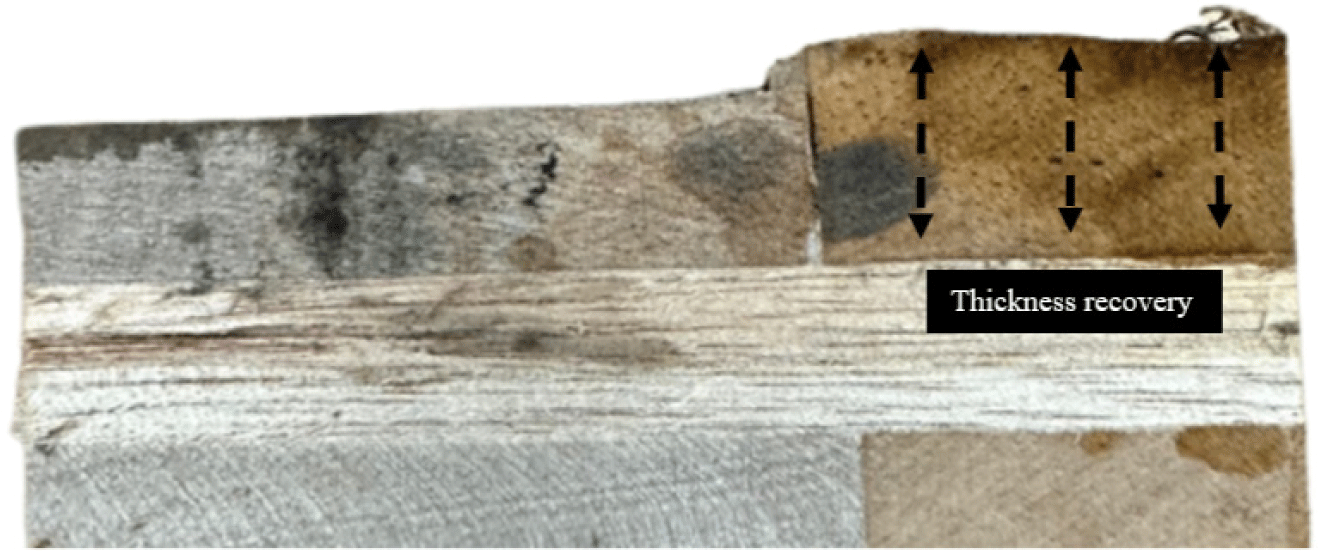
Understanding the temperature dynamics during the burning process is vital to assess the fire performance of CLTs (Albert and Liew, 2024). The dynamic temperature measurement (10-minute intervals), illustrated in Fig. 9, indicated that Channels 1 and 8 have the highest increases in temperature due to the placement of the Type-K thermocouples at the points with the highest heat concentration. Channel 4, in contrast, had the slowest temperature increase. The fire rapidly spread along the grain direction from the 0th to the 10th minute (Fig. 10), until the char layer started to accumulate. The temperature of the upper region of the test piece surface gradually increased, indicating that the fire spread was concentrated in that area.
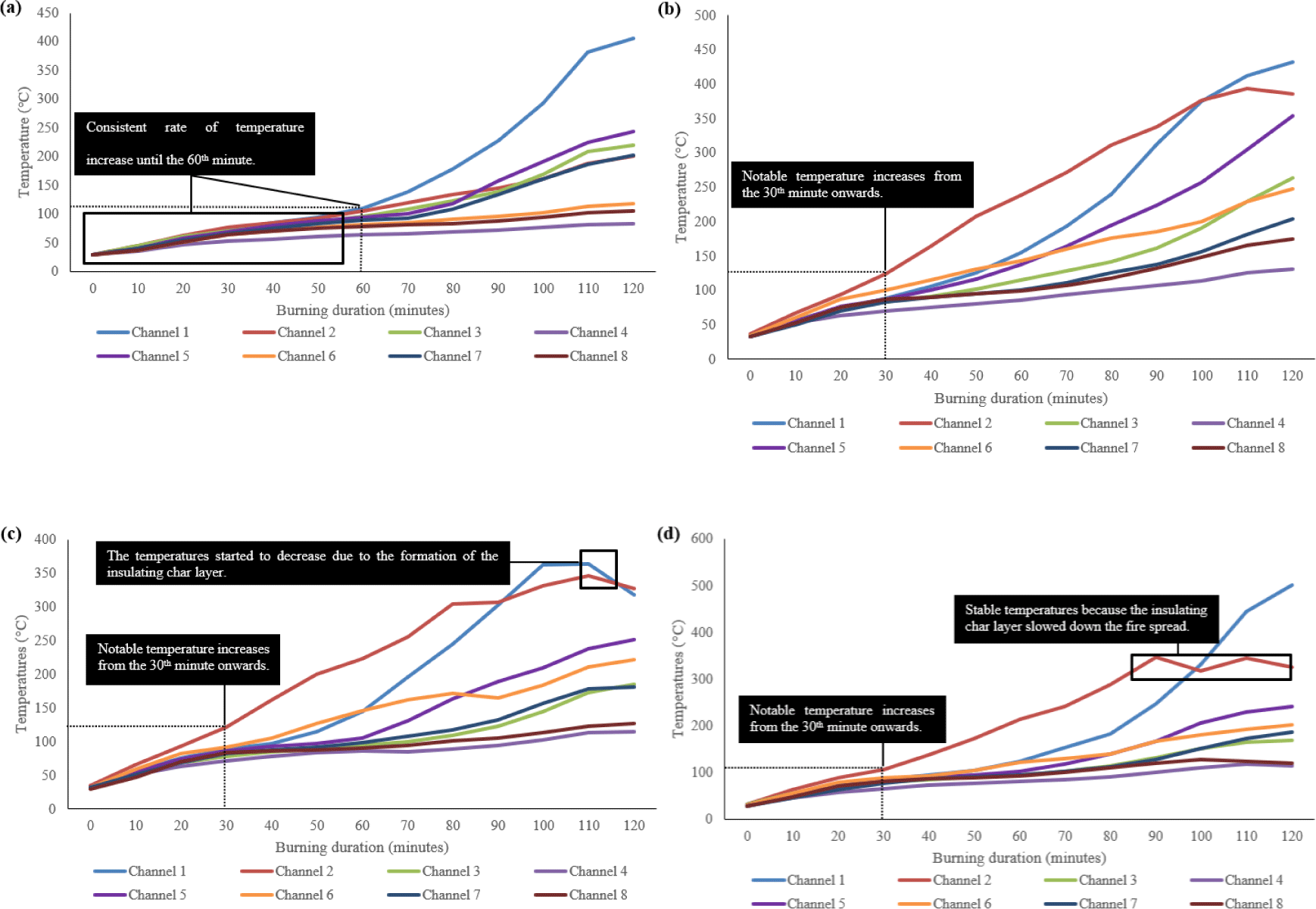
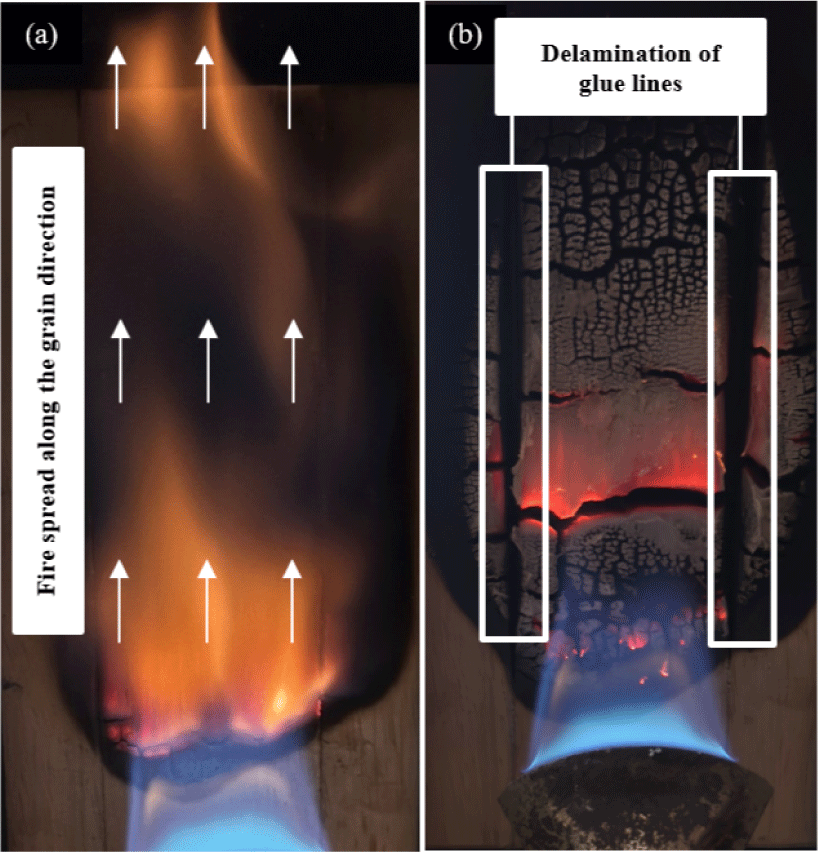
Within 120 minutes, the control achieved a maximum temperature peak of 405°C, with values as follows: 40CR (431.94°C), 50CR (364.19°C), and 60CR (502.25°C). The densified parameters were associated with notable temperature increases from the 30th minute (Fig. 9) due to delamination of the glue lines on the CLTs (Fig. 10). The delamination occurred because the polyvinyl acetate started to decompose rapidly as it reached its boiling point (> 75°C), causing the fire to propagate to the middle layer, reaching the thermocouple tip (Conner and Bhuiyan, 2017; Holland and Hay, 2002). A previous study found that higher compression ratios are associated with higher delamination rates due to inefficient adhesive penetration into the laminas (Albert and Liew, 2020). This phenomenon occurred because increasing the compression ratio resulted in compacted lumens inside the laminas after the densification process (Albert and Liew, 2020).
The control, however, exhibited a steady rate of temperature increases until the 60th minute because non-densified laminas have excellent wettability, which enabled efficient adhesive penetration. The rapid decomposition of polyvinyl acetate and the resulting delamination emphasize the importance of the adhesive properties in maintaining the structural integrity of CLT during fire scenarios (Conner and Bhuiyan, 2017). Higher compression ratios increase the physicomechanical properties of the timber but result in poor wettability. Therefore, the discovery of a balanced approach in the densification process is needed, where the densified laminas should have the desirable physicomechanical characteristics and excellent wettability to produce CLTs with stable structural integrity upon exposure to fire. As shown in Fig. 9, the temperatures started to decrease or stabilize because the accumulated char layer acted as a heat insulator—rapid char layer formation when the pyrolysis rate of the laminas started to reach the temperature range between 280°C and 500°C (Albert and Liew, 2024).
4. CONCLUSIONS
In conclusion, this study provides insights into the fire performance of CLT manufactured from densified P. falcataria laminas. Higher compression ratios resulted in a protective char layer, substantially limiting the charring rate and boosting the fire resistance of the CLT. In particular, a 50% compression ratio delayed the charring rate compared to that of the control, indicating an optimal compression ratio for improving fire resistance. However, a 60% compression ratio did not result in further improvements. The study also revealed the effect of short-duration densification on the temperature profile during burning, where the densified parameters exhibited significant temperature spikes beginning at the 30th minute owing to glue line delamination. This delamination occurred due to the rapid decomposition of polyvinyl acetate, highlighting the importance of the adhesive characteristics in maintaining structural integrity during fires. Temperature changes and charring rates provide a more detailed understanding of how densified timber responds to open burning settings, whereby the findings highlight the importance of a balanced densification procedure, considering physicomechanical characteristics and wettability, to provide durable structural integrity of the CLT during fire situations.