1. INTRODUCTION
Meranti and keruing wood are two types of tropical hardwood that are widely used in construction, particularly as a raw material for the building and housing industries (Lee et al., 2024; Viholainen et al., 2021). Wood is a renewable resource, has great mechanical properties, is an excellent thermal insulator, and is easy to shape and join (Popovski et al., 2014).
Several pieces of wood can be nailed together to form a specific structure to achieve the assembly convenience of wood (Han et al., 2023). The advancement and enhancement of connectivity technologies utilizing nails will additionally expand the potential of construction methods involving wood (Pang et al., 2017). Local carpenters in East Kalimantan frequently use both ordinary and “ulin nail” or concrete nails to join wood. Concrete nails are classified as “square boat nails”. They have a square cross-section, as opposed to the round cross-section of ordinary nails. For the same length, ordinary nails have a marginally smaller cross section than concrete nails (Cohen et al., 2015). Galvanized concrete nails are more expensive than ordinary nails (Amayreh, 2022).
After the wood has been fastened, nails that are left in place for an extended period of time will begin to corrode. If left undetected, corrosion of the nails can cause the nail joints to come loose (Takanashi and Sawata, 2017), as a result of metal corrosion, there has been a decrease in weight (Suprapti et al., 2020). Knowing the rate at which nails corrode will help avoid the danger of disconnecting a joint where the nails have corroded.
Each species of wood is distinguished by its anatomical structure, physical properties, mechanical properties, and chemical properties (Berglund and Burgert, 2018) such as wood extractives. Some wood extractives, such as phenolic compounds (Yeon et al., 2019), inhibit corrosion (Aourabi et al., 2021), while others, such as organic acid compounds such as fatty acid and resin acid in heartwood (Arisandi et al., 2024), accelerate corrosion (Maraveas, 2020). Corrosion of metals embedded in wood is affected by three types of extractives: organic acids, tannins and phenols (Abo Elgat et al., 2021). Unfortunately, the amount and types of extractives in keruing and meranti wood sold in lumber stores and their effect on the corrosion rates of ordinary and concrete nails, have not been investigated. The purpose of this study was to determine the amount and types of extractives in keruing and meranti wood sold in lumber stores, and their effects on the corrosion rates of ordinary and concrete nails based on electrochemical test.
2. MATERIALS and METHODS
Meranti wood sample was purchased from Mustaqim Proprietorship lumber store, and keruing wood sample was purchased from Putera Harapan Ibu Proprietorship lumber store, in Samarinda City, East Kalimantan Province, Indonesia. The wood samples purchased from the lumber stores, each measuring 4 × 6 × 400 cm, consisted of two pieces per wood species. Sawdust was produced by cutting the wood at every 100 cm intervals using a circular saw.
The extractive content of the wood was evaluated according to TAPPI 204 cm-97 (Arango-Perez et al., 2023). The thimble containing 2 ± 0.1 grams of 40–60 mesh sawdust was placed in the Soxhlet extractor containing 300 mL of methanol. The duration of the extraction process was four to five hours. The extracted sawdust was dried in an oven at 103 ± 2°C to determine its extractive free weight. The extracted solution was filtered using Whatman microporous filter paper with a pore size of 0.45 μm and a diameter of 47 mm. The resulting filtrate was filtered and divided into two portions, 200 mL for electrochemical testing and 100 mL for gas chromatograph-mass spectrometer (GC-MS) testing.
The chemical compound content of the wood extractives was determined by GC-MS (Mangindaan et al., 2017; Yeon et al., 2019). A rotary evaporator was used to concentrate 100 mL of the Soxhlet extraction filtrate, yielding 8 g of concentrated extract, which was used for GC-MS analysis. A Shimadzu QP 2010 GC-MS was used for the measurement. The GC-MS was equipped with a Restek column, RTx-5MS capillary column (30 m × 0.25 mm I.D. and 0.25 μm). The RTx-5MS column has a working temperature range of 50°C–300°C, initial temperature 50°C (hold 5 min) to final temperature 300°C in increments of 5°C/min (hold 12 min); interface temperature 270°C; split ratio 1:0; and pressure 108.1 kPA; helium gas as carrier gas. GC-MS readings indicate retention time, chemical constituents, and concentrations (Guerrero-Chanivet et al., 2020). The National Institute of Standards and Technology (NIST) library database version 11 was used for component identification (Vieira et al., 2021). Chemical compound concentrations were determined from the peak area of the GC-MS graph (Baccolo et al., 2021).
Four-inch ordinary nails and concrete nails were purchased from a hardware store in Samarinda. The ends and heads of the nails were then cut to a length of four centimeters for use in the electrochemical test. The treatment for electrochemical testing is shown in Table 1. Each treatment was tested in three replicates.
Code | Wood extractives | Nail |
---|---|---|
CK | Keruing | Ordinary nail |
CM | Meranti | Ordinary nail |
UK | Keruing | Concrete nail |
UM | Meranti | Concrete nail |
Electrochemical testing using a potentiostat/galvanostat to determine the corrosion rate was in accordance with ASTM 199-09 (Almeraya-Calderon et al., 2024). The electrolyte liquid was derived from meranti and keruing wood extracts produced by Soxhlet extraction. The electrochemical test results show the corrosion currents, potentials, and rates (Nyby et al., 2021). The electrochemical test results are reported as means with SDs.
3. RESULTS and DISCUSSION
Based on TAPPI 204 cm-97 test, the extractives content of keruing wood was 3.83% and meranti wood was 1.97%. Keruing wood contained 3.83% extractives and meranti wood contained 1.97% extractives. In comparison, the heartwood of the base of Shorea retusa had an extractive content of 4.69%–5.87%, Shorea macrophylla had an extractive content of 2.35%–4.27%, and Shorea macroptera had an extractive content of 10.62%–11.37% (Yunanta et al., 2014). Meanwhile, the extractive content of Dipterocarpus glabrigemmatus wood was 8.8%, Dipterocarpus stellatus was 6.8%, and Dipterocarpus pachyphyllus was 7.7% (Dewi and Supartini, 2017). The study results showed that the extractive content of the wood test samples had a lower value than the reference. The reference’s extractive content was measured from felled tree trunks, whereas the study’s extractive content was assessed from sawn wood sold in wood shops. Cutting wood from logs to lumber and the time the lumber is stored or stacked in the workshop may result in reduced wood extractives (Chen et al., 2020). The GC-MS test results of keruing wood extractives are shown in Table 2.
The keruing wood extractives contained a lot of oleoresin. The oleoresin in Dipterocarpus gracilis is dominated by caryophyllene and caryophyllene oxide (Fernandes and Maharani, 2019), in Dipterocarpus grandiflorus by β-bisabolene (Wahyudianto et al., 2020), and in Dipterocarpus verrucosus by caryophyllene and α-humulene (Fernandes and Maharani, 2022). Based on Table 2, keruing wood extractives contained coumaran (27.28%), sitostenone (17.26%), methyl p-coumarate (12.11%), antiarol (9.57%), and γ-sitosterol (9.86%). Coumaran compounds can be natural inhibitors to prevent corrosion in mild steel in 0.5 M HCl solution (Yaqoob et al., 2023). Methyl p-coumarate inhibits corrosion on metal surfaces by an anionic mechanism (Udabe et al., 2021). Whereas, antiarol compounds can inhibit corrosion by preventing excessive oxidation (Sprang et al., 2022). The γ-sitosterol compound can adhere parallel to the metal surface and is supported by the activity of heterooxygen atoms, aromatic carbon groups and the presence of double bonds, so it can effectively prevent corrosion reactions on metal surfaces (Rehioui et al., 2023). Meanwhile, sitostenone is a ketone derivative of sitosterol (Szakiel et al., 2022), so its corrosion inhibition mechanism is similar to that of sitosterol compounds. The results of GC-MS analysis of meranti wood extractives are shown in Table 3.
Shorea leprosula wood contains guaiacol, furfural, levoglucosan and 4-vinylguaiacol (Sulistyo et al., 2017). Based on Table 3, the investigated meranti wood was dominated by 1,8-nonadyine 76.15%. Compound 1,8-nonadiyne, can form a thin layer on metal surfaces to inhibit corrosion (Yoshinobu et al., 2018). Another extractive compound in meranti wood that acts as another inhibitor is 1-(4-Acetamidoanilino)-3,7-dimethylbenzo (4,5) imidazo (1,2-a) pyridine-4-carbonitrile. Derivatives of imidazo (1,2-a) pyridine effectively inhibit corrosion because metal surfaces readily absorb the proton transfer (Salim et al., 2019). The effect of wood extractives on the corrosion rate of ordinary nails and concrete nails is shown in the following Tafel plot in Fig. 1.
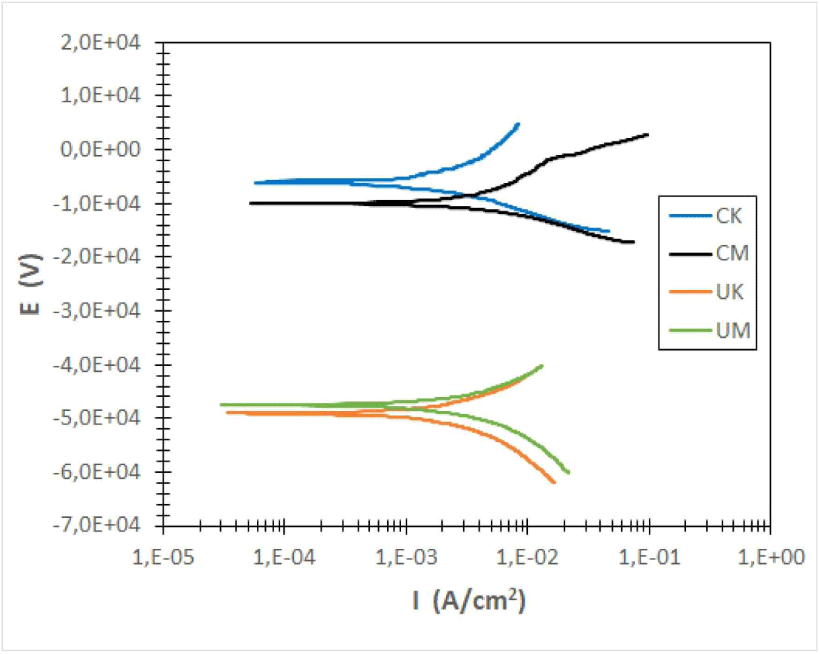
According to the Tafel plot, concrete nails corroded slower than ordinary nails. Ordinary nails are an industrial product with a low iron content and a high susceptibility to corrosion (Santoso et al., 2022). Concrete nails, on the other hand, are high-quality industrial nails manufactured from stainless steel with a surface coating (Du et al., 2015). The coating layer consists of corrosion-resistant substances, such as (Pb) lead, (Zn) zinc, (Cd) cadmium and (Sb) antimony (Bram et al., 2020). The corrosion rate of low-quality nails ranges from 0.12 to 0.06 mm/year (Li et al., 2011). The corrosion rate of steel nails is 90 μm/year and that of aluminum is 60 μm/year (Zelinka and Rammer, 2009).
Based on Table 2, chemical compounds with cyclic carboxylic groups, i.e., coumaran, antiarol, methyl p-coumarate, 2-cyclohexen-1-one, 4-hydroxy-3,5,6-trimethyl-4-(3-oxo-1-butenyl), γ-sitosterol, sitostenone, and only one compound with an aliphatic or straight-chain carbon structure, namely hexadecanal (2.30%), dominated the extractives of keruing wood. Whereas, based on Table 3, the meranti wood extractives were dominated by compounds with aliphatic or straight-chain carbon groups, i.e., 1,8-nonadiyne with 76.15% and methyl hexacosanoate with 0.74%. As corrosion inhibitors, compounds with a cyclic carbon structure have tremendous potential (Verma and Quraishi, 2021) as they inhibit corrosion more effectively than aliphatic or straight-chain carbon structures (Alamiery et al., 2021).
According to Table 2, the keruing wood extractives contained 5.87% elaol and 2.40% 3-(2,5-dimethoxyphenyl) propionic acid. Elaol is slightly soluble in water and is weakly acidic when dissolved in water. On the other hand, 3-(2,5-dimethoxyphenyl) propionic acid is a weak acid that dissolves in water based on an equilibrium reaction. From Table 3, it can be seen that meranti wood extractives contained 5.66% elaol and 0.73% sulfurous acid, cyclohexylmethyl pentadecyl ester, which is acidic and accelerates the corrosion rate. Therefore, the fastest corrosion rate occurred in ordinary nails on meranti wood extractive media than keruing wood as shown in Fig. 1.
According to Table 4, corrosion potential is the potential at which the anodic and cathodic reaction rates are equal, meaning that the measured current changes sign at this potential when the potential is scanned as in potentiodynamic polarisation. Current density is the current density on the metal surface describing the general corrosion rate of metals (Nyby et al., 2021). Lower corrosion current density shows that the metal corrodes faster (Sohail et al., 2020).
4. CONCLUSIONS
Keruing wood contained 3.83% extractives, dominated by cyclic carbon structure, such as coumaran. Meranti wood contained 1.97% extractives, dominated by aliphatic carbon structure such as 1,8-nonadiyne. The presence of organic acids, sulfurous acid, cyclohexylmethyl pentadecyl ester, in meranti wood extractives accelerates the corrosion rate faster than keruing wood extractives. The lowest corrosion rate based on electrochemical tests was on concrete nail in keruing wood extractive media, which was 0.65 μm/year, and the highest corrosion rate was on ordinary nail in meranti wood extractive media, 3,74 μm/year. The nail corrosion rate in keruing wood extractive media is lower than that in meranti wood extractive media.
In general, wood containing acidic extractives causes faster nail corrosion rates. Therefore, it is recommended to use steel nails with an anti-rust coating. Future studies should include testing wood nailing in outside circumstances.