1. INTRODUCTION
The high demand for teak wood has been fulfilled with an effort to develop fast-growing teak (FGT). Biotrop teak is one of the products of FGT development in Indonesia. The increasing development of FGT in Indonesia will inevitably increase the amount of waste produced, especially from shoot waste. The abandoned shoot waste of teak is not considered as a valuable part of trees, not converted to industrial wood, and only categorized as waste and utilized as firewood (Nurullah, 2023). To solve this problem, reducing waste is an effective approach to advancing environmentally friendly wood production and technology (Agrawal and Chopra, 2020). This appropriate utilization of wood waste can affect the ecosystem (Owoyemi et al., 2016); for example, the production of charcoal from biomass has increased owing to the recent environmental problems of increased fuel use (Kwon et al., 2018). Pyrolysis is the current trend for optimizing products and achieving sustainable processes and products in the utilization of biomass waste (Januszewicz et al., 2020). An alternative effort to optimally utilize shoot waste of FGT is its conversion into activated carbon.
From the perspective of industrial development, activated carbon is among the most important adsorbents. Activated carbon is derived from many types of carbonaceous materials, such as coal, peat soil, peanut shells, coconut husks, wood, and solid waste (Kim et al., 2019). From its initial development, activated carbon garnered considerable attention owing to its high adsorptive, thermal, ecological, electrical, and mechanical properties (Dungani et al., 2022). The basic concept is that the original features of the surface area of charcoal is covered by a hydrocarbon residue that blocks its adsorption effectiveness. After thermal treatment, the surface area of activated carbon is relatively free of residue, its surface becomes wider, and the pores are open, thereby increasing its adsorption capacity (Lempang, 2014). This advantage of activated carbon, which has a large surface area, is the increase in its adsorption capability to reduce pollutants and contaminants (Aini et al., 2021). The activation process aids in the development of a greater surface area and improves the internal porosity to increase the adsorption function (Contescu et al., 2018). Owing to its specific characteristics of surface area and porosity, activated carbon can be applied to the purification of domestic and industrial wastewater, desalination, gas distillation, and separation, removal of odor and pollutants, as well as medical applications globally (Heidarinejad et al., 2020). Activated carbon in industrial applications is commonly used as an adsorbent of liquid pollutants, gas separator, and catalyst support material (Sadir et al., 2022).
Utilizing the shoot waste of FGT for the production of activated carbon is one alternative for waste utilization that is in line with FGT development. With appropriate strategies, converting the shoot waste of FGT to activated carbon will help maintain the equilibrium between the needs of economic growth and environmental protection. We investigated the conversion of shoot waste of FGT to activated carbon to determine the best conditions for the activation process; we implemented two treatment factors for the activation process, including thermal treatment (750°C, 850°C, and 950°C) and heating period (30, 60, and 90 min). Additionally, the appropriate utilization of the shoot waste of FGT to produce activated carbon can result in valuable byproducts, reduce the amount of waste, and contribute to sustainable development; therefore, this process holds great potential.
2. MATERIALS and METHODS
The objective of this study was to realize the appropriate utilization of shoot waste of FGT to produce activated carbon. We evaluated the effect of thermal treatment and heating period on the quality of produced activated carbon, especially the adsorption properties of activated carbon derived from the shoot waste of FGT.
The raw material used was shoot waste from 14-year-old FGT initiated from three trees, which was extracted from the community forest in Wonosari, Gunungkidul, Yogyakarta Special Region, Indonesia. Shoot waste of FGT was collected from the top of the tree after decreasing the diameters to less than 5 cm (Fig. 1). Shoot waste of FGT was then formed into disks with a thickness of 5 cm and cleaved into chips with a thickness and length of 0.5–0.9 and 8–10 cm, respectively. The chips were then air dried to achieve a water content of 10%–15%. The chemicals used showed pro-analysis purity levels, and included sodium thiosulfate (Na2S2O3), sodium hydrogen carbonate (NaHCO3), potassium iodide (KI), benzene (C6H6), distilled water, methylene blue, and starch indicator.
The research apparatus includes a furnace (FB 1410M-33, Thermo Fisher Scientific, Waltham, MA, USA) with a capacity of 2.1 L and an energy consumption of 1,520 W. It has a temperature range of 100°C to 1,100°C, a temperature of stability of ± 5.0 at 1,000°C, and requires an electrical input of 240 V at 50/60 Hz. Additionally, we used an oven (UN 55, Memmert, Eagle, WI, USA) that requires an electrical input of 230 V at 50/60 Hz and consumes 2,000 W of power. We used an PAJ 2003 scale (Ohaus, Parsippany, NJ, USA) and 188CT hot plate (Shindo, Fukui, Japan) consuming 200 W of power and requiring an electrical input of 240 V. The WP S800+ spectrophotometry machine was used, requiring an electrical input of 90–265 V at 50/60 Hz. The other equipment included a magnetic stirrer, pipette, filter paper, porcelain cup, mesh-screen, desiccator, beaker glass, and burette.
This study was conducted with a completely randomized design and implemented by an activation process with two treatment factors, including thermal treatment (750°C, 850°C, and 950°C) and heating period (30, 60, and 90 min) with five replications.
The carbonization process of shoot waste of FGT was conducted with an electric retort at a heating temperature of 500°C for 4 h. The resulting charcoal was fractured into charcoal powder and filtered through a 10-mesh filter and stuck on a 20-mesh filter to ensure a uniform dimension size of charcoal, which was ready for the activation process (Fig. 2).
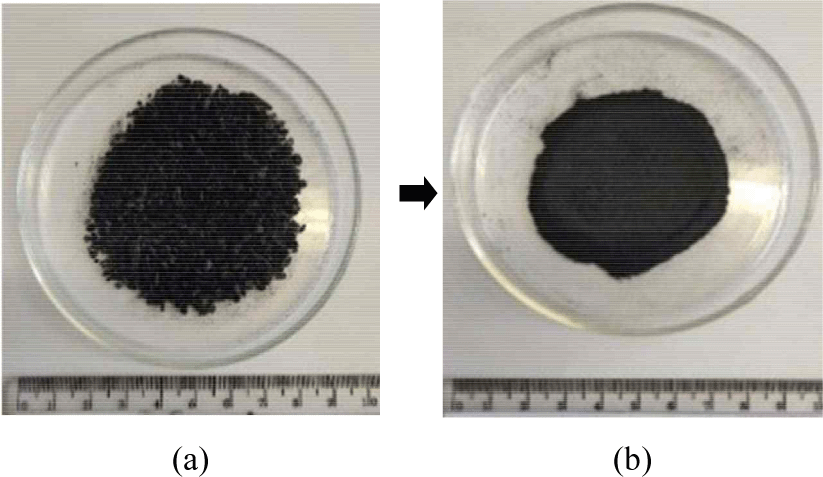
As much as 23 g of FGT charcoal powder derived from shoot waste was collected in each treatment and placed in a porcelain cup before placing it in the furnace for thermal activation at a heating rate of 25°C/min. The activation treatment was conducted with five replications.
The quality parameters of activated carbon derived from the shoot waste of FGT include: yield, moisture content (MC; ASTM D 1762-84; ASTM, 2007), volatile matter content (VMC; ASTM D 1762-84), ash content (AC; ASTM D 1762-84), fixed carbon content (FCC; ASTM D 3172-89; ASTM, 2002), and adsorption capacities of benzene (ACB; SNI 06-3730-1995; Badan Standardisasi Nasional, 1995), methylene blue (ACM; SNI 06-3730-1995), and iodine (ACI; SNI 06-3730-1995).
Yield was calculated based on the weight ratio of produced activated carbon to required charcoal, based on the following Equation (1):
W1: weight of charcoal (g), W2: weight of activated carbon (g).
The procedure for calculating MC is in accordance with the American Society for Testing and Materials (ASTM) D-1762-84 standard. MC was calculated using the following Equation (2):
W1: weight of the initial sample (g), W2: weight of the sample after heating (g).
The calculation of VMC was in accordance with the ASTM D-1762-84 standard. VMC was calculated using the following Equation (4):
WL: weight loss, W1: weight of the sample (g), W2: weight of the sample after heating (g).
The calculation of AC was in accordance with the ASTM D-1762-84 standard. AC was determined using the following Equation (5):
W1: weight of the sample (g), W2: weight of the sample after heating (g).
The measurement of FCC was in accordance with the ASTM D-3172-89 standard; FCC was calculated using the following Equation (6):
The measurement of ACB was in accordance with the Indonesian National Standard (SNI) 06-3730-1995. The procedure was conducted by inserting 1 g of activated charcoal into a desiccator that was saturated with benzene vapor for 24 h; subsequently, the final weight of activated charcoal was determined.
ACB was calculated using the following Equation (7):
W1: weight of the initial sample (g), W2: weight of the sample after the adsorption of benzene (g).
The measurement of ACM was in accordance with SNI 06-3730-1995. A 0.2 g sample of activated carbon with a passing size of 325-mesh was mixed with 25 mL of methylene blue by shaking for 45 min; it was then filtered and left for 24 h. The methylene blue-filtered solution was then diluted by adding 200 mL of distilled water. The absorbance of the solution was then tested using a spectrometer at a wavelength of 660 nm.
ACM was determined using the following Equation (8):
C: methylene blue concentration, Fp: dilution factor, B: weight of the sample (g).
The calculation of ACI was in accordance with SNI 06-3730-1995.
A 0.5-g sample of activated carbon with a passing size of 325-mesh was dissolved into 50 mL of iodine solution by shaking for 30 min. Subsequently, the mixture was filtered and left for 24 h. Finally, three drops of starch solution was added to 10 mL of the solution, followed by titration with sodium thiosulfate until the appearance of a bright yellow color.
ACI was calculated using the following Equation (9):
V: required sodium thiosulfate solution, N: normality of sodium thiosulfate, W: weight of the sample, 12.69: amount of iodine in the 0.1-N sodium thiosulfate solution.
3. RESULTS and DISCUSSION
The yield is defined as the ratio of weight of produced activated carbon to the original charcoal required (Maia et al., 2021). During production, high yield is preferred as it contributes to minimizing the production costs (Soleimani and Kaghazchi, 2007). The thermal treatment and heating period showed a significant influence on the yield of activated carbon of shoot waste of FGT (Table 1). Fig. 3 shows that the highest temperature during thermal treatment (950°C) resulted in the lowest yield of activated carbon (72.69%). The highest activated carbon yield of shoot waste of FGT (75.88%) was achieved by thermal treatment at 750°C. The yield obtained in this study result is similar with those of previous studies; namely, higher rates of thermal treatment and heating period will increase the surface area but reduce yield (Jun, 2010; Sulaiman et al., 2018). The yield obtained in this study was higher than those of activated carbon derived from teak wood waste (38.23%; Yusop et al., 2022) and paper mill sludge (49.2%; Begum et al., 2023). Another previous study found that the rate of thermal treatment has a significant impact on the yield (Zhao et al., 2017), and increasing the heating period during the activation process decreased the yield (Jun, 2010). This is because higher activation temperatures resulted in the further loss of carbon or decreased stability of the carbon structure, implying decreased yield (Brunner and Roberts, 1980).
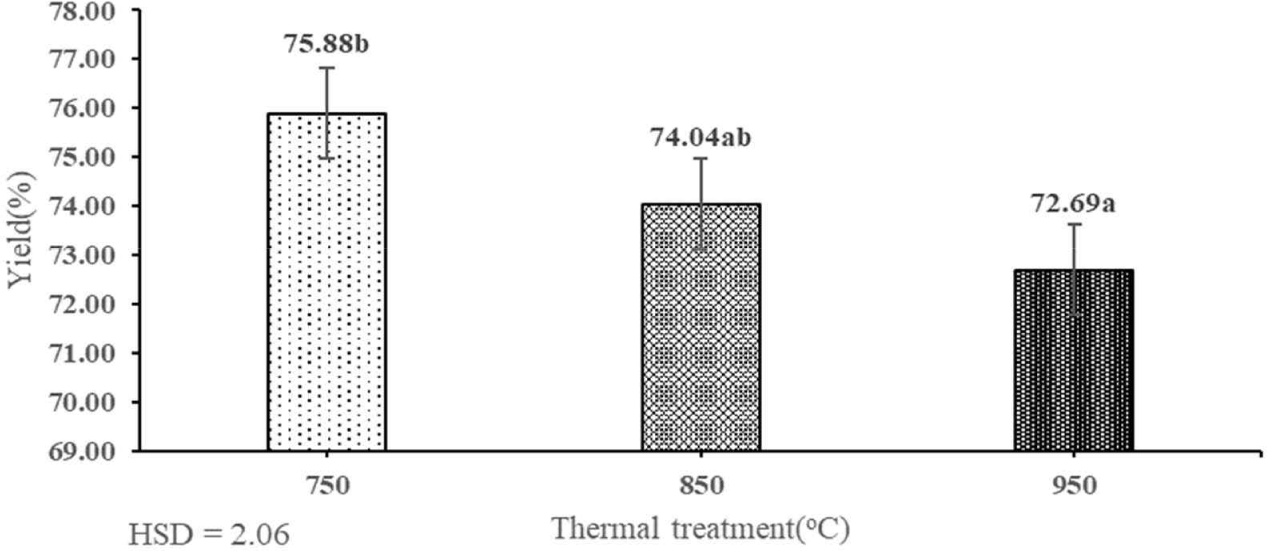
The MC of activated carbon is an important quality parameter as an increase in MC resulted in the decrease of its absorption capability (Abiko et al., 2010). The MC of activated carbon of shoot waste of FGT ranged from 0.84% to 6.13% (Table 2). This result indicated that the interaction between thermal treatment and heating period had a significant effect on the MC of activated carbon of shoot waste of FGT at the 1% precision level (Fig. 4). The best MC (0.84%) was obtained by thermal treatment at 950°C for a period of 90 min. The lowest MC of activated carbon (0.84%) was higher than that of activated carbon derived from mangrove charcoal (0.38%; Budianto et al., 2019). Conversely, the MC of activated carbon derived from the shoot waste of FGT was lower than those derived from orange peel (10%; Lam et al., 2017) and teak sawdust (5.02%; Ramirez et al., 2017). Pari (2000) found that a high level of MC is influenced by the hygroscopic characteristics of activated carbon. The MC depends on the temperature and heating period because by increasing the temperature, H2O begins to change into the gaseous phase; after reaching its boiling point (100°C), free water bound to carbon is released and the MC decreases. The MC of activated carbon derived from the shoot waste of FGT with thermal treatment at 750°C, 850°C, and 950°C and heating periods of 30, 60, and 90 min fulfilled the SNI quality standard (≤ 15%).
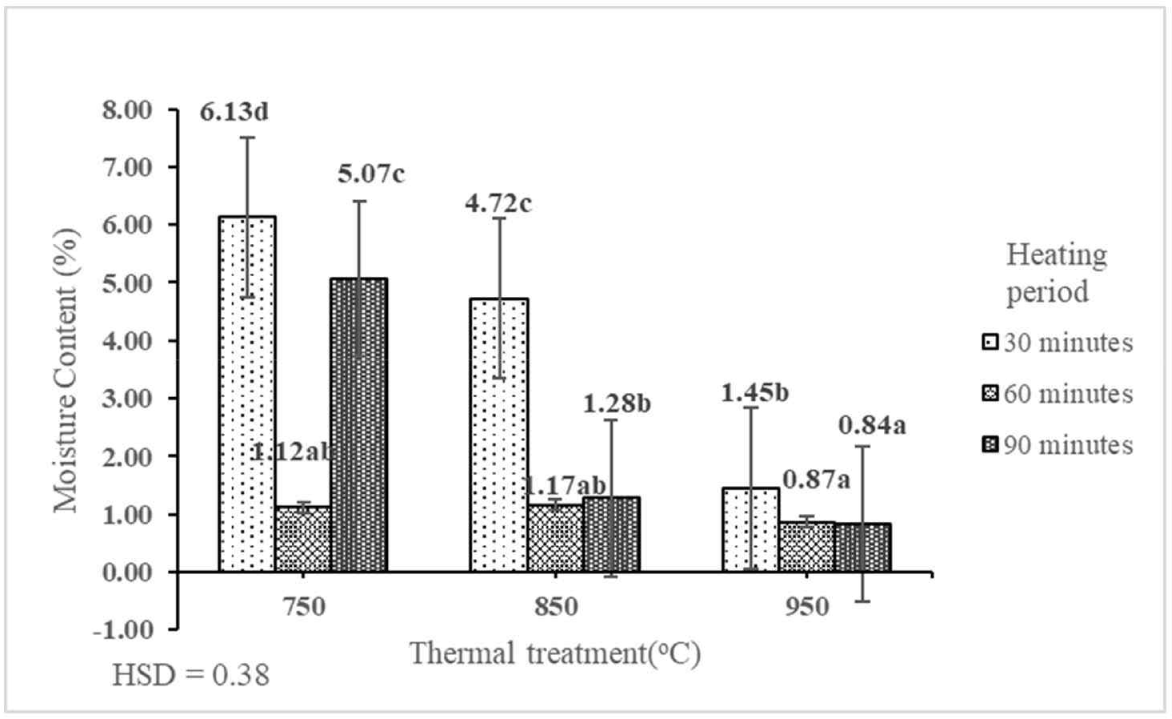
VMC indicated that the flying surface of activated carbon was still attached to the non-carbon compound fly and substances originating from the interaction of carbon with water vapor (Haji et al., 2013). VMC of activated carbon derived from the shoot waste of FGT ranged from 9.16% to 20.38% (Table 2). This result indicated that the interaction between thermal treatment and heating period had a significant effect on the value of VMC of activated carbon derived from the shoot waste of FGT at the 1% precision level (Table 1). The best VMC (9.16%) was obtained by thermal treatment at 950°C for 90 min. Furthermore, the VMC of activated carbon derived from the shoot waste of FGT decreased with the increase in the rate of thermal treatment and heating period (Fig. 5). This is because significantly lower VMC of the carbonized sample is caused by the removal of volatile compounds in the form of a gas during the high-rate thermal treatment (Ju et al., 2020). The best VMC (9.16%) in this study was lower than those of activated carbon derived from teak sawdust (83.92%; Ramirez et al., 2017) and raw rice husks (26.7%; Alvarez et al., 2014). The average VMC of activated carbon derived from the shoot waste of FGT (13.92%) was similar to that obtained by Haji et al. (2013), who produced activated carbon from urban organic waste (13.68%), which was produced with thermal treatment at 800°C and heating period of 60 min. The VMC of activated carbon derived from the shoot waste of FGT with thermal treatment at 750°C, 850°C, and 950°C and heating periods of 30, 60, and 90 min fulfilled the SNI quality standard (≤ 25%).
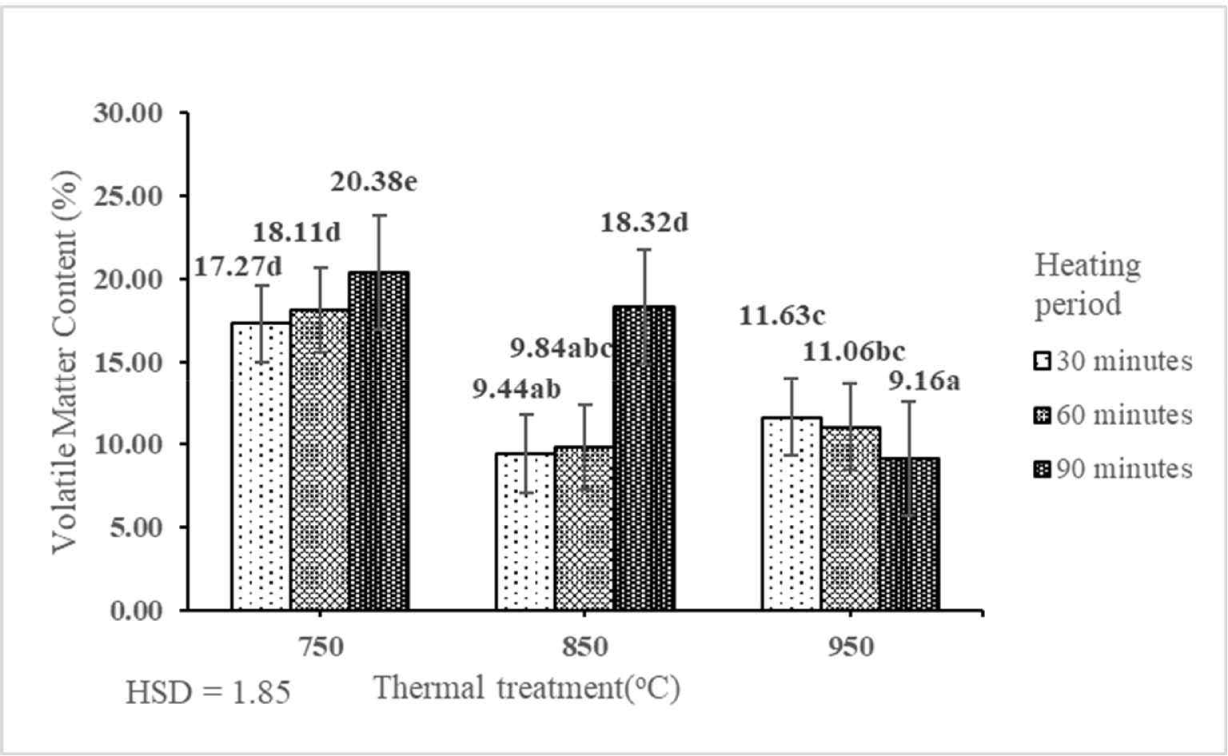
In this study, we showed that the AC of activated carbon derived from the shoot waste of FGT ranged from 4.03% to 5.24% (Table 2). This indicated that the interaction between the thermal treatment and heating period had a significant effect on the AC of activated carbon derived from the shoot waste of FGT at the 1% precision level (Table 1). The best/lowest AC (4.03%) was produced by thermal treatment at 950°C for 90 min. The AC of activated carbon derived from the shoot waste of FGT decreased with the increase in the rates of thermal treatment and heating period (Fig. 6). The best AC (4.03%) in this study was lower than those of activated carbon derived from orange peel (10%; Lam et al., 2017) and raw rice husk (6.3%; Alvarez et al., 2014). The advantage of lower AC is that activated carbon is more effective as an adsorbent (Ekpete and Horsfall, 2011). This is owing to the lack of carbon elements in the ash and the predominance of several minerals, such as potassium, calcium, magnesium, and silica, which are unfavorable for adsorbents (Cahyani et al., 2023). Inorganic components of the raw material may contribute to the AC. The AC of activated carbon is the residue left behind after the combustion of the carbon portion of the material (Soleimani and Kaghazchi, 2007). The AC of activated carbon derived from the shoot waste of FGT with thermal treatment at 750°C, 850°C, and 950°C and heating periods of 30, 60, and 90 min fulfilled the SNI quality standard (≤ 10%).
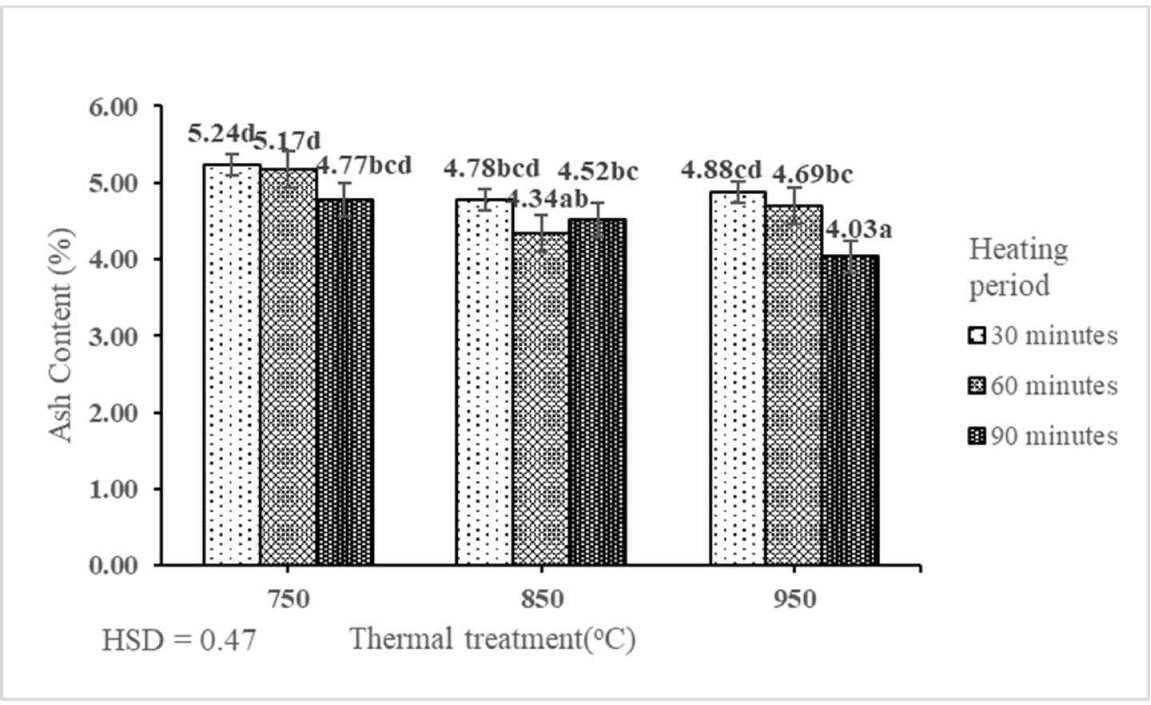
The FCC of activated carbon derived from the shoot waste of FGT ranged from 74.84% to 86.80% (Table 2). This result indicated that the interaction between thermal treatment and heating period had a significant effect on the FCC of activated carbon derived from the shoot waste of FGT at the 1% precision level (Table 1). The best FCC (86.80%) was obtained by thermal treatment at 950°C for 90 min. This indicated that the FCC of activated carbon derived from the shoot waste of FGT increased with increasing rates of thermal treatment and heating period (Fig. 7). The best FCC (86.80%) in this study was higher than those of activated carbon derived from teak sawdust (15.67%), which was calcined at 550°C and washed with 2 M HCl (Ramirez et al., 2017), and raw rice husk (67%; Alvarez et al., 2014). The FCC of activated carbon derived from the shoot waste of FGT with thermal treatment at 750°C, 850°C, and 950°C and heating periods of 30, 60, and 90 min fulfilled the SNI quality standard (≥ 65%).
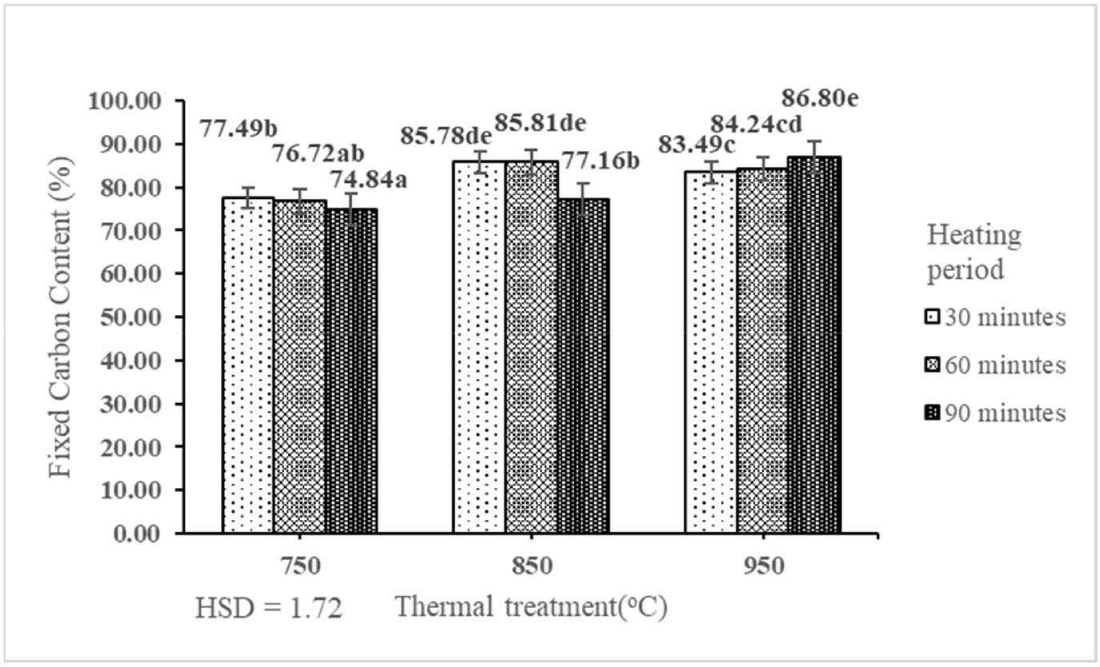
Micropores and the size of pores influence the adsorption capacity of gas molecules present in activated carbon (Danish and Ahmad, 2018). Benzene can be found in both primary and secondary pollutant forms (Isinkaralar, 2022). This indicated that the interaction between thermal treatment and heating period had a significant effect on the ACB value of activated carbon derived from the shoot waste of FGT at the 1% precision level (Table 1). The ACB of activated carbon derived from the shoot waste of FGT ranged from 7.53% to 10.29% (Table 3). The best ACB (10.29%) was produced by thermal treatment at 750°C for 60 min. The ACB of activated carbon derived from the shoot waste of FGT decreased with increasing rates of thermal treatment and heating period (Fig. 8). The best ACB (10.29%) of activated carbon derived from the shoot waste of FGT was lower than those of activated carbon derived from teak sawdust (38.85%; Erawati and Afifah, 2019) and banana peel (51%; Imelda et al., 2019). The low ACB indicates that the surface of activated carbon still contains non-carbon compounds, such as hydrogen and oxygen atoms, that make the surface more non-polar (Haji et al., 2013). The ACB of activated carbon of shoot waste of FGT with thermal treatment at 750°C, 850°C, and 950°C and heating periods of 30, 60, and 90 min did not fulfil the SNI quality standard (≥ 25%).
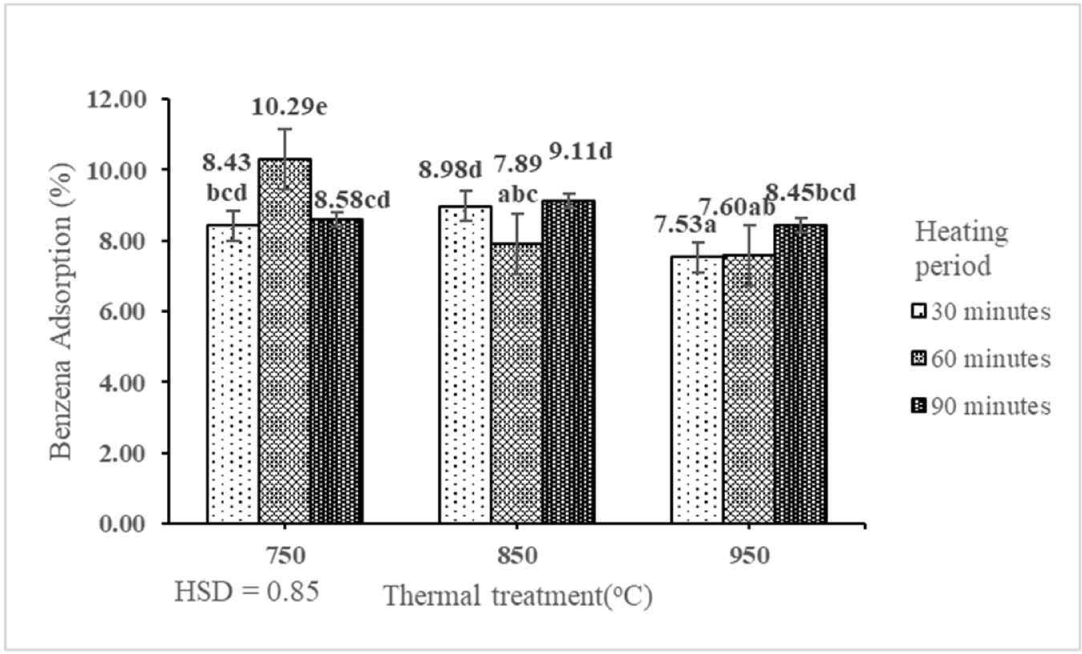
Methylene blue is used to determine the ability of activated carbon to adsorb liquids (Ahmad et al., 2007). Methylene blue dye with a molecular size of 19 nm is generally used as a dye in textile industries. Methylene blue is a model compound to test the adsorption of organic contaminants from a liquid solution (Banat et al., 2003). According to a review by Danish et al. (2018), activated carbon produced with a longer activation time (120 min) has a higher adsorption capacity compare with that produced with a shorter activation time (50 min); this difference depends on the pore size distribution of the produced activated carbon. The ACM of activated carbon derived from the shoot waste of FGT ranged from 69.66 to 111.77 mg/g (Table 3). The result indicated that the interaction between thermal treatment and heating period had a significant effect on the ACM of activated carbon derived from the shoot waste of FGT at the 1% precision level (Table 1). The best ACM (111.77 mg/g) was obtained by thermal treatment at 950°C for 90 min. The ACM of activated carbon derived from the shoot waste of FGT increased with increasing rates of thermal treatment and heating period (Fig. 9). The best ACM (111.77 mg/g) in this study was lower than those of activated carbon derived from Gumitir (199.93 mg/g; Sahara et al., 2017) and bamboo (183.3 mg/g; Liu et al., 2010). Conversely, the ACM in this study was higher than that of activated carbon derived from teak wood waste (66.69 mg/g; Yusop et al., 2022). Most of the methylene blue was already adsorbed within the first 20–30 min; subsequently, owing to the rapid increase in adsorption quantities, the increase in adsorption gradually slowed down with increasing contact period until pseudo-equilibrium was reached (Yang and Cannon, 2022). The methylene blue that could be adsorbed may decrease owing to its difficulty to access the pores inside the activated carbon samples as it is a high-molecular-weight dye (Duy Nguyen et al., 2019). The ACM of activated carbon derived from the shoot waste of FGT with thermal treatment at 750°C, 850°C, and 950°C and heating periods of 30, 60, and 90 min did not fulfil the SNI quality standard (≥ 120 mg/g).
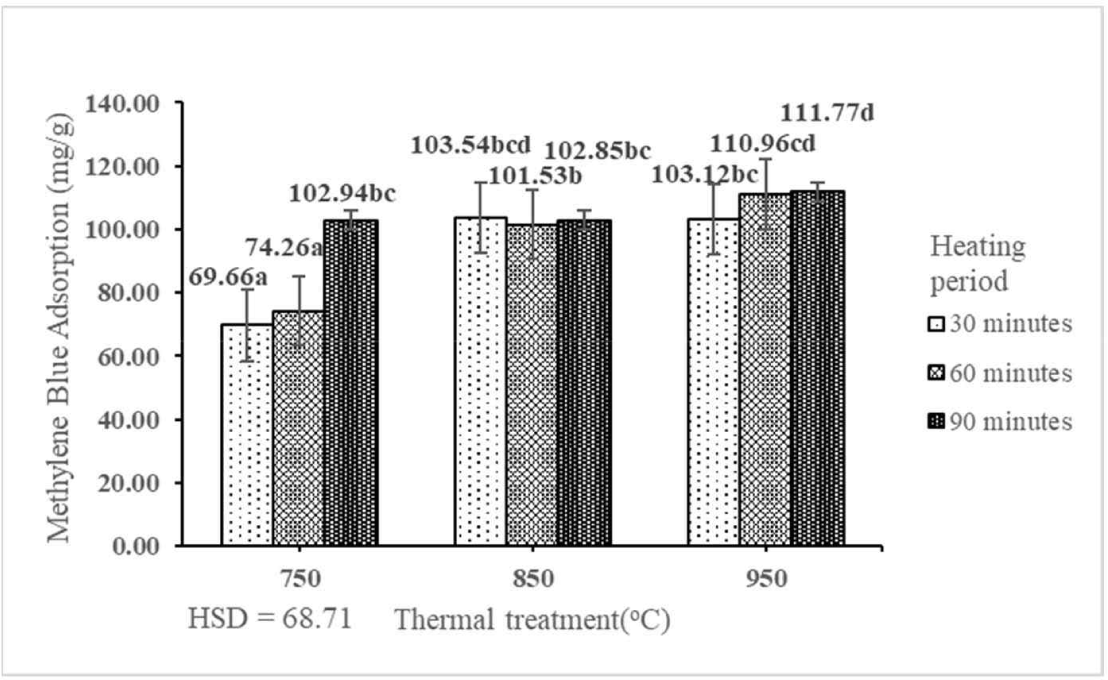
One fundamental criterion for evaluating the effectiveness of activated carbon is the ACI (Ekpete and Horsfall, 2011). The ACI determines the ability of activated carbon to remove color from an aqueous solution (Bestani et al., 2008). The ACI of activated carbon is affected by the internal pore structure, surface characteristics, surface area, and presence of functional groups (Ismadji et al., 2005). The ACI of activated carbon derived from the shoot waste of FGT ranged from 862.68 to 1,102.57 mg/g (Table 3). The result indicated that the interaction between thermal treatment and heating period had a significant effect on the ACI of activated carbon derived from the shoot waste of FGT at the 1% precision level (Table 1). The best ACI (1,102.57 mg/g) was obtained by thermal treatment at 750°C for 30 min. The ACI of activated carbon derived from the shoot waste of FGT decreased with increasing rates of thermal treatment and heating period (Fig. 10). The best ACI (1,102.57 mg/g) was higher than those of activated carbon derived from paper mill sludge (761.4 mg/g; Begum et al., 2023), teak wood (314.15 mg/g; Ratnani et al., 2019), and palm oil fronds (888.55 mg/g; Maulina et al., 2020). Conversely, the ACI in this study was lower than that of activated carbon derived from banana peel (1,243.62 mg/g; Setiawan et al., 2023). The ACI of activated carbon derived from the shoot waste of FGT with thermal treatment at 750°C, 850°C, and 950°C and heating periods of 30, 60, and 90 min fulfilled the SNI quality standard (≥ 750 mg/g).
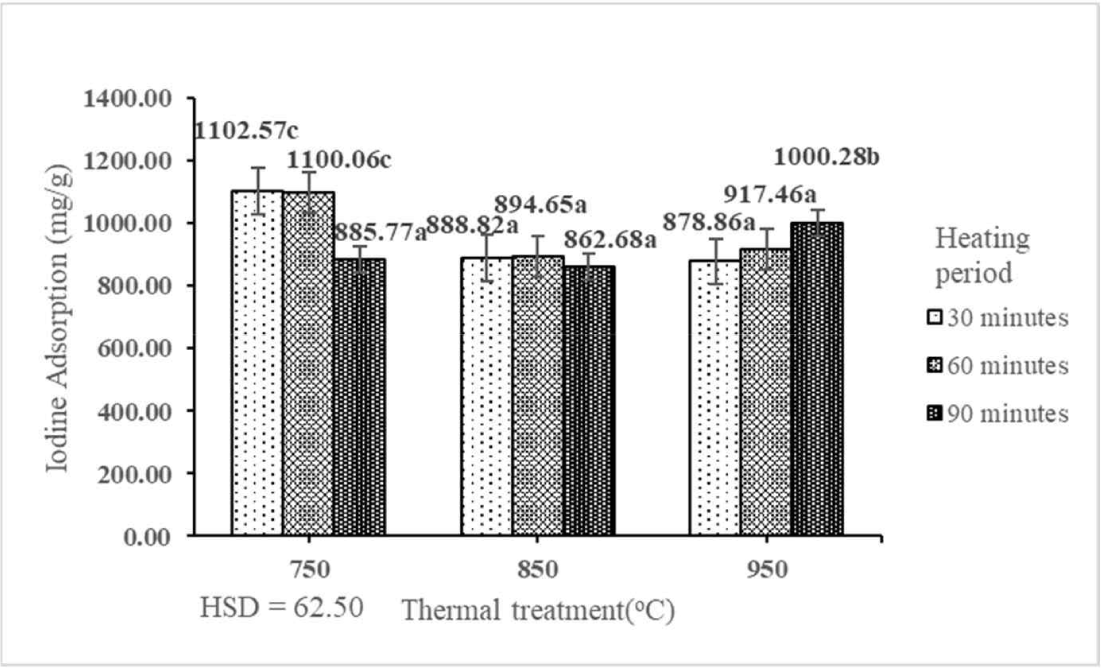
4. CONCLUSIONS
Based on our results, we concluded that the production of activated carbon from the shoot waste of FGT holds great potential, and the abandoned shoot waste of FGT could be classified as a prospective material for producing activated carbon for industrial application.
The highest ACI of activated carbon of shoot waste of FGT was 1,102.57 mg/g, which was obtained by thermal treatment at 750°C and heating period of 30 min; this value fulfilled the SNI 06-3730-1995 quality standards. Furthermore, the quality parameters of the produced activated carbon are as follows: MC of 6.13%; VMC of 17.27%; AC of 5.24%; FCC of 77.49%; benzene removal efficiency of 8.43%; and ACM of 69.66 mg/g.
Finally, the increase in thermal treatment and heating period decreased the yield of activated carbon derived from the shoot waste of FGT; thus, we recommend thermal treatment at 750°C and a heating period of 30 min to obtain a satisfactory yield of activated carbon of 75.88%.