1. INTRODUCTION
Logistics plays a pivotal role in efficiently distributing vast quantities of goods worldwide in the current global trading era. Ensuring safe transportation of commodities from suppliers to manufacturers, warehouses, retailers, and consumers is crucial. Within logistics systems, pallets are commonly used to safely and efficiently distribute large quantities of goods through various modes of transportation (Bilbao et al., 2011; Handoko et al., 2021; Waseem et al., 2013). Wood pallets have traditionally been relied upon as a dependable means of facilitating this process. However, the use of solid wood for block pallet production raises concerns regarding illegal logging and its detrimental environmental impacts. Addressing this issue in the wood industry requires exploring alternative materials and adopting sustainable practices aligned with ecological responsibility and adherence to legality. On the other hand, plastic pallets have emerged as an alternative to wooden pallets. These plastic pallets can be reused multiple times, and possess strengths comparable to those of their wooden counterparts. However, their production process consumes more energy than standard wooden pallets, resulting in a greater impact on climate change (Deviatkin et al., 2019; Khan et al., 2021).
Teak wood (Tectona grandis sp.) from the family Verbenaceae is a high-quality wood with a beautiful texture. The original distribution area of this species includes India, Laos, Myanmar, Indonesia, and Thailand, with plantations estimated to cover 4.346 million hectares (Kollert and Cherubini, 2012). In Indonesia, particularly in Jepara and Central Java, teak wood has become a significant local commodity for the furniture industry, driving the wheels of local and national economies through furniture and handicraft exports to approximately 193 countries worldwide (Gatut Prakosa and Ramadhan, 2021). However, the wood production process also generates approximately 1.4 million cubic meters of wood waste annually, consisting of 22.32% wood slashes, 9.39% wood chips, and 8.77% sawdust (Obidzinski et al., 2014; Purwanto, 2009). Traditionally, this waste has been used as compost to enhance soil fertility. Nevertheless, the economic value derived from this utilization remains relatively low and needs to be increased by exploring its potential in composite product applications. In addition, pellet-based packaging and logistics are predominantly employed. The use of waste wood products to produce chip block pallets (CBP; pallet bearings) as functional composite products presents an opportunity for a sustainable pallet solution.
Composite products are generally developed using formaldehyde-based synthetic adhesives (Iswanto et al., 2020; Jamaludin et al., 2020; Rachmawati et al., 2018; Sumardi et al., 2022; Trisatya et al., 2023; Zhang et al., 2019). In the case of composite products, particleboards commonly utilize formaldehyde-based adhesives owing to their low cost and favorable mechanical properties. However, these adhesives emit the unhealthy and environmentally unfriendly formaldehyde (Beane Freeman et al., 2013; Dorieh et al., 2019; Salthammer et al., 2010). An alternative adhesive is polyurethane, a polymeric material containing a urethane group (-NH-CO-O-) formed by the reaction of polyols with isocyanates (Chattopadhyay and Webster, 2009; Triwulandari and Ghozali, 2013). Isocyanate-based adhesives offer several advantages, including preservation, improved physical and mechanical properties, and the absence of harmful chemical emissions. Moreover, the use of polyurethane adhesive compared to conventional urea-formaldehyde (UF) adhesive is more efficient, as it requires a smaller proportion (no more than 12%) and eliminates the need for heat treatment (Song and Kim, 2022). In addition, polyurethane adhesives have been developed for composite applications, demonstrating physical and mechanical properties that meet the JIS A 5908-2003 (Kusumah et al., 2010; Masturi et al., 2020; Zaia et al., 2015).
Therefore, this study aimed to investigate the production of CBP from teak wood biomass with varying polyurethane contents. In addition, this study analyzed the optimum particle-size composition was determined. Teakwood biomass was selected as the raw material for CBP production because of its renewable nature and the potential to utilize byproducts from the furniture industry. The quality of the CBP products was assessed by examining their physical and mechanical properties using a Universal Testing Machine (UTM) and comparing them to standards set by the National Wooden Pallet and Container Association (NWPCA). Additionally, the morphology of the CBP products was evaluated using field-emission scanning electron microscopy (FE-SEM) to analyze the distribution of the polyurethane adhesive content within the product composition. The main objective of this study was to produce sustainable CBP products from waste wood in an industry that meets the required standards for use in packaging and logistics systems.
2. MATERIALS and METHODS
Teakwood (T. grandis sp.) biomass, such as chips, particles, and sawdust, obtained from the home furniture industry in Jepara (Central Java, Indonesia), was used as the raw material. These materials were sifted into two types of particles, namely coarse (4–14 mesh) and powder (> 60 mesh), then dried in the oven at 80°C until the moisture content (MC) reached 5%. Adhesive resins containing a Polyol Blend (ARK R01880/AD, Batch number: PB/59600921) and methylene diphenyl diisocyanate (MDI; ARK R01880/AD, Batch number: UTAA270632) were purchased from PT. Anugerah Raya Kencana, Tangerang, Banten, Indonesia. The polyurethane adhesives were prepared at a polyol:MDI molar ratio of 1:1 (w/w%). The polyurethane was mixed with methyl chloride at a ratio of 1:2 (w/w) for adhesive applications.
CBP production was divided into two stages (Table 1 and Fig. 1). The first stage evaluated the use of polyurethane adhesive content, whereas the second stage considered the effect of particle size composition. In the first stage, polyurethane adhesive content was varied in four variants (2.5%, 3%, 3.5%, 4.5%), with 50:50 particle size composition between coarse and powdered (4–14 mesh and > 60 mesh; Sijabat et al., 2017). The particles were mixed and sprayed with adhesive resins using a spray gun (Fig. 1) and then formed in a 9 × 9 × 9 cm3 molding box. The particles’ weight is adjusted to attain a density target of 0.6 g/cm3 and pressed using the cold press method for 4 hours at a specific pressure of 9.8 MPa. Subsequently, CBP’s physical and mechanical properties of CBP were evaluated to determine the optimal adhesive content for the second manufacturing stage. In the second stage, the compositions of coarse (4–14 mesh) and powder (> 60 mesh) particles were varied in five ways, as shown in Table 1. Before evaluation, every CBP product is conditioned for 7 days at room temperature (25 ± 2°C).
NWPCA standards were utilized to evaluate the CBP products’ density, MC, dimensional stability (DS), water absorption (WA), compressive strength (CS), and screw-holding strength. The samples were prepared with dimensions of 5 × 5 × 5 cm3 (Fig. 2). To check density and MC, the test samples were weighed initially and then dried for 24 hours at 105 ± 2°C until constant weight was achieved. The DS and WA were measured by immersing the CBP sample for 24 h. After immersion, the sample was dried and weighed, and its width, length, and height were measured. The screw holding strength (SHS) and CS were evaluated using a UTM 50 kN load (AG-IS, Shimadzu, Kyoto, Japan). Each sample was evaluated in triplicate.
The CBP morphology was observed using a FE-SEM to observe the distribution of particles and their interactions with the polyurethane adhesive resins. This characterization was performed using FE-SEM (Quattro S, Thermo Fisher Scientific, Waltham, MA, USA) with 100 × and 500 × magnification (Fig. 3).
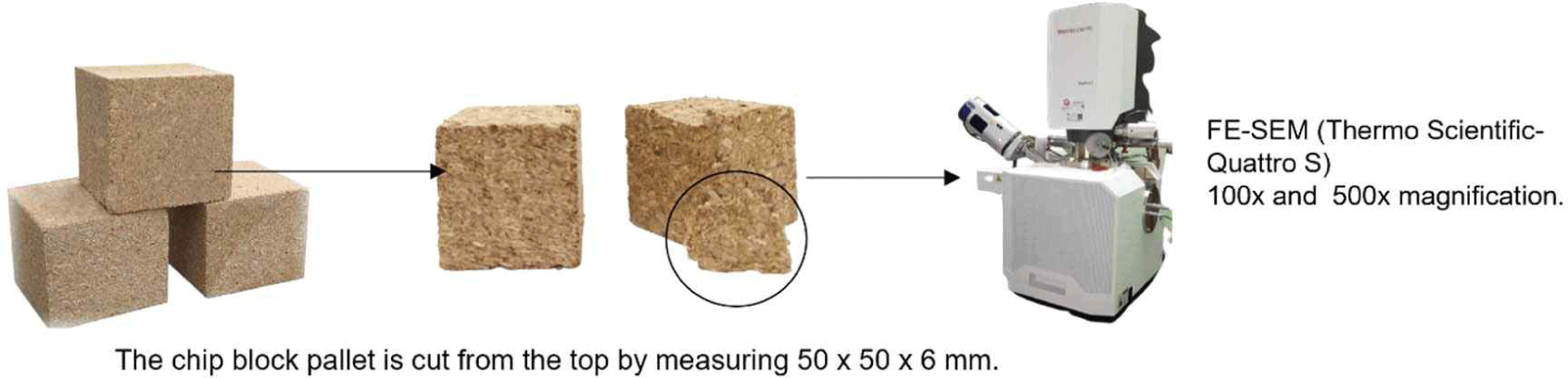
All data were evaluated using IBM Statistical Product and Service Solution ver. 23 with the analysis of variance (ANOVA) method to assess the importance of the variation between variables and levels. The Duncan multi-range test was used to compare the means and determine whether groups had substantially different means at a 95% confidence level.
3. RESULT and DISCUSSION
The CBPs exhibited a density ranging from 0.57 to 0.61 g/cm3, meeting the intended target and close to those of commercial products (Table 2). The NWPCA set no specific standards for density and MC. Nevertheless, the results indicate that CBPs produced using polyurethane resin can be used as pallet bearings in logistics. Furthermore, the study revealed a gradual increase in the density with increasing polyurethane adhesive content (Raharjo, 2020). ANOVA results demonstrated that density did not significantly affect CBP (Table 3). MC plays a crucial role in determining the quality of the composites produced (Suroto, 2010). The MC of CBPs exhibited values of 6.84%. Drying the particles before the treatment significantly lowered the MC. Moreover, statistical tests conducted on the MC of CBPs indicated that variations in adhesive content substantially affected the quality of CBPs.
DS refers to the ability of a material to resist changes in dimensions caused by fluctuations in MC, which can be influenced by weather and humidity conditions. The DS values of the CBPs are listed in Table 2. The DS tended to decrease as the adhesive content increased, which is consistent with previous findings (Syahfitri et al., 2022). This phenomenon can be attributed to the significant influence of the high-DS on the WA characteristics of CBPs. However, DS and WA are closely related parameters. The use of varying adhesive content resulted in a notable reduction in the DS of the CBPs. The lengths of the CBPs satisfied the required standards, with the lowest value recorded at 1.41% after immersion, surpassing the minimum requirement of 2%.
After immersion, it was observed that the height and width of CBPs did not meet the prescribed standards of 2% and 6%, respectively (Table 2). The WA values ranged from 80.80% to 84.91%. These values failed to meet the criteria outlined by the NWPCA standards, which stipulate a maximum allowable WA value of 25%. Statistical tests conducted using the WA did not show any significant effects. This can be attributed to the particle-to-matrix ratio of the raw materials. When the polyurethane resin content is low, the binding between the matrix and particles become less effective, forming voids (Desiasni et al., 2021). These voids can affect the ability of the material to resist WA because they can trap and retain water between particles (Desiasni et al., 2021; Syahfitri et al., 2022). These voids result in higher WA values (Jawaid et al., 2011; Prakoso and Pratiwi, 2018).
The mechanical properties of the CBPs were assessed to determine their CS and SHS. This evaluation aimed to demonstrate the successful mixing of adhesives and particles in the CBPs (Widyorini et al., 2019). The CS of the CBPs ranged from 11.18 MPa (Table 2). This value satisfies the standards set by the NWPCA, which require a minimum chip block strength value of 9.65 MPa. The statistical analysis results indicated that the variation in adhesive content significantly influenced the CS and SHS of the CBPs, as shown in Table 2.
The results indicated that an increased polyurethane adhesive content corresponds to a higher CS value, where the matrix effectively interacts with all particles (Zaia et al., 2015). During pressing, the polyurethane polymer primarily interacts with the particles. The addition of polyurethane adhesive enhanced the CS of the composite. It should be noted that when a polymer is added to the filler, there may be poor adhesion between the surface forces owing to the hydrophobic nature of the polyurethane matrix and the hydrophilic nature of teak wood (Jaya et al., 2016). The bonded matrix transmitted the load in all directions, making the composite more robust and capable of withstanding maximum loads (Masturi et al., 2011b). The increase in CS can also be attributed to reduced voids within the composite and increased adhesive content (Mirindi et al., 2021). The matrix and powder particles fill the voids, and the voids that remain unfilled during the formation process lead to a decrease in the CS of the CBPs. The matrix acted as a reinforcer and continuously received stress.
When CBPs are subjected to a load, the stress concentration moves to the void area, resulting in a reduction in the strength of the CBPs and the emergence of cracks, ultimately leading to composite failure (Hasan et al., 2020). The obtained CS results were consistent with the expected values for teak wood, including the CS, specific gravity, and density, as reported by Mirindi et al. (2021). The SHS value increased with the addition of polyurethane adhesive. The SHS of the CBPs ranges from 104.68 N to 336.56 N. The highest SHS value was achieved at an adhesive content of 4.5%. This study demonstrated that a higher concentration of adhesive leads to greater screw-retaining strength. This can be explained by the fact that a higher adhesive level increases the opportunity for the adhesive to enter the particle cavities, thereby strengthening the bonds between the particles (Aprillia et al., 2019; Iswanto et al., 2016; Jamaluddin et al., 2018).
Particle geometry, including the particle shape and size, is an important parameter that influences composites’ physical and mechanical properties (Eghtedarnejad et al., 2021; Mawardi et al., 2022). After determining that 4.5% polyurethane yielded the best results, the impact of particle size composition was evaluated. The CBPs exhibited a density range of 0.59–0.60 g/cm3 and a MC range of 5.07%–6.47% (Table 4). Several factors contribute to the difference between target and actual densities, including wasted particles, variations in manufacturing processes, sample measurements, and cutting methods (Mawardi et al., 2022). High-density CBPs contained more particles per unit volume than low-density CBPs. The incorporation of powder particles has been proven to establish strong binding between particles, reduce interparticle spacing, and prevent surface gaps in CBPs. The density of the produced CBP was comparable to that of commercial CBP products.
Furthermore, statistical tests indicated that the variance in particle size composition during CBP manufacturing did not significantly affect the density of the CBPs. In the preparation stages of composite production, the drying process is crucial for reducing high MC, optimizing energy usage, and achieving the desired quality (Dukarska et al., 2022). The MC of the CBPs, with a value below 12%, is comparable to that commercial CBP products. Statistical tests revealed that the variance in the particle size composition significantly affected the MC value of the CBPs.
WA and DS of the CBPs were assessed and compared with NWPCA standards. The WA of the CBPs ranged from 94.59, which was considerably high and did not comply with the specified standard of 25%. The selection of raw materials also influences WA, as the cellulose and hemicellulose components exhibit significant levels, leading to increased WA of sawdust particles owing to the presence of free hydroxyl groups that exhibit a high affinity for water (Hermawan et al., 2015). However, teak wood has a relatively high cellulose content, ranging from 45.7% to 29.7% for lignin (Lukmandaru, 2010). The ratio of the particle raw materials and the matrix used influences the DSA value of the CBP. The lower the polyurethane matrix used, the less effective the matrix is at binding particles, resulting in voids (Desiasni et al., 2021). Voids affect the material in terms of binding water. These voids can trap water between particles if the matrix does not cover the surface (Desiasni et al., 2021; Hasan et al., 2020). Water can fill wood lumens, is present in wood as free and bound water, and can chemically bond via hydrogen bonds. Similarly, isocyanate has a highly reactive group originating from the radical -N = C = O (Nuryawan et al., 2008).
DS reflects the performance of a material, as it can affect other attributes, such as mechanical performance, electrical conductivity, and physical performance (Taib and Julkapli, 2018). The DS values are presented in Table 4, and the statistical tests demonstrate that WA and DS are significantly affected by various particle size compositions (Table 5). Factors such as the density, fiber ratio, MC, WA, type of fiber or reinforcement, size, distribution, orientation, and porosity influence the material’s performance (Gao et al., 2016; Xu et al., 2012).
DS is strongly influenced by particle size. Consequently, component porosity can pose challenges when combining different materials, as these spaces can trap moisture and affect the DS. Voids can be reduced by uniformly spreading and mixing fillers of different particle sizes throughout the material. The size of the void pores can also vary, ranging from centimeters to micro-or nanoscale, depending on the homogeneity of the composite material. Reduced voids in the composite can enhance the DS by preventing water from filling the voids, leading to a lower WA. Zafar et al. (2016) reported a reduction in the number of voids when fillers were added to a composite matrix. However, the DS of CBPs with variations in particle size composition still does not meet the NWPCA standards.
Additionally, coating applications using hydrophobic materials on CBPs are highly preferable for improving the DS and reducing WA (Nemli et al., 2007). If considered by calculating compression ratio, namely the comparison between the density of the board and the raw material, the CBP compression ratio value is 0.96, with the density of the teak raw material being 0.55 g/cm3 in line with research (Sulastiningsih et al., 2017) that to produce good contact between particles, a compression ratio of 1.3 is usually required. Maloney (1993) stated that the optimal compression ratio was 1.3. A high compression ratio will increase the mechanical properties of the board, but on the other hand, it will reduce DS (Suhasman et al., 2006).
The CS of the CBPs ranges from 3.80 MPa to 14.67 MPa (Table 4). This range meets the standards specified by the NWPCA, which require a minimum value of 9.65 MPa. CBPs composed of coarse particles exhibited the highest strength values. Statistical tests indicated that the CS value was significantly affected by the particle size. The strength of the CBPs is determined by the structure and properties of their components. The polymer matrix was bound to the particles and formed a composite. The matrix in the composite material plays a critical role in the reception and transmission of stress to the particle fibers. Therefore, the fiber component should possess a higher stress and elasticity modulus than the matrix. A chemical bond between the matrix and fiber is suspected to contribute to the ability of the material to withstand shear stress (Hamzeh et al., 2011; Velmurugan and Manikandan, 2005).
The presence of coarse particles in the composite can restrict chain flexibility, resulting in an enhanced CS (Masturi et al., 2011a). The SHS of the CBPs ranges from 209.43 N to 371.50 N (Table 4). CBPs composed of powder particles exhibited the lowest SHS values, whereas CBPs consisting of coarse particles demonstrated the highest SHS value of 371.50 N. The results of this study aligned with those of Rigg-Aguilar et al. (2019), who developed CBP using PVAc and UF adhesives. The statistical test results indicated that the variation in the particle size composition significantly affected the screw-holding strength (Table 5). The results showed that larger particles exhibited greater strength in holding screws than powder particles.
The CBPs were analyzed using FE-SEM to confirm optimal mixing of the adhesive and particles. Voids or pores often occur during the CBPs manufacturing process, which refers to gaps or imperfect arrangements of particles that can increase the panel weight owing to water entrapment in the voids (Prakoso and Pratiwi, 2018).
Fig. 4(a) presents an FE-SEM image of mixed CBPs incorporating both coarse and powder particles. It is seen that the natural pores from the coarse particles are uniformly distributed through the sample. These voids can accelerate WA, leading to weak interfacial bonds and inefficient stress transfer (Obasi et al., 2014). CBPs quickly absorb water under extreme conditions, which affects their DS and WA properties. These findings align with those of previous studies, highlighting the use of powder particles to minimize pore formation within composites (Syahfitri et al., 2022; Zafar et al., 2016). Numerous voids within the composite indicate an unfavorable association with the physical properties (Odeyemi et al., 2020).
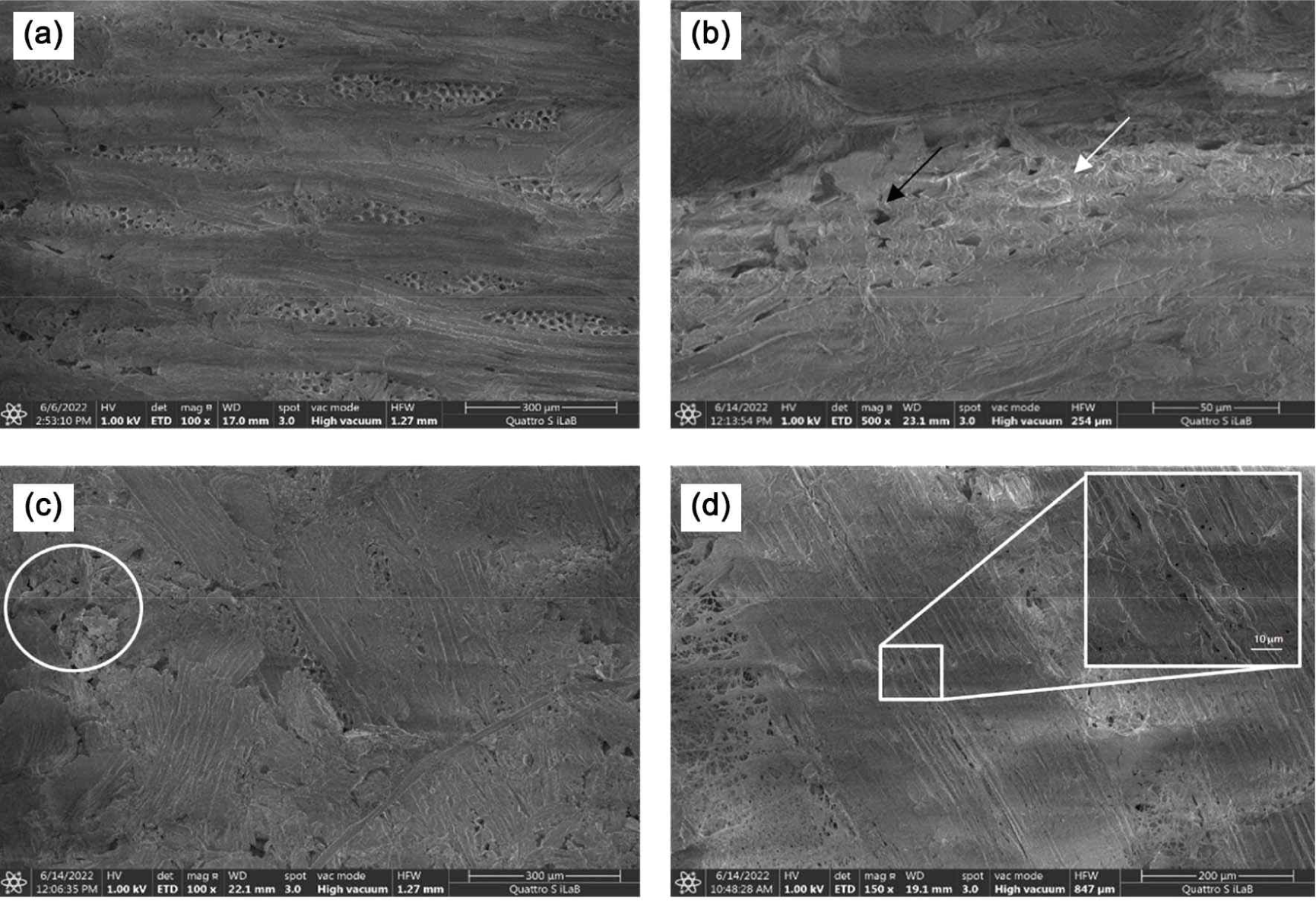
Another spot was captured at 500 × magnification [Fig. 4(b)]. The black arrows indicate empty void cavities that were not filled with adhesive. The white arrow denotes the adhesive infiltration into the voids, validating the successful mixing process and the subsequent decrease in voids within the CBPs. Moreover, a decrease in the number of voids filled with powder particles and polyurethane adhesive within the cavity regions was observed at the particle boundary area. This suggests the successful penetration of particles and adhesives into the voids, resulting in their size reduction Fig. 4(b), white arrow], strengthening the bond between the wood particles, and enhancing the physical and mechanical properties of the CBPs. Coarse particles exhibit better load-bearing capabilities than powdered particles (Kavitha et al., 2015).
CBPs made from coarse particles [Fig. 4(c)] contained voids of various sizes (white circles), contributing to the high WA value of the CBPs. The CBPs composed of coarse particles exhibited high CS values. This can be attributed to the ability of the fiber particles to effectively support the applied load stress, even in the presence of voids, indicating a direct interaction between the stress and the particles.
Fig. 4(d) shows a fine-particle FE-SEM image with a lower strength and a higher number of voids, resulting in rapid WA corresponding to the MC value. Many voids are assumed to originate from the PU adhesive, which produces a porous structure with insufficient particle reinforcement. The decrease in the CS value of the CBPs was explained by the applied load stress not being effectively restrained by the matrix and particle fibers. Consequently, stress is concentrated within the voids, leading to the quick cracking and crumbling of the chip blocks (Saidah et al., 2018).
4. CONCLUSIONS
CBP were successfully produced using a facile cold press method from teak wood (Tectona grandis) biomass. The CBP sample prepared using 4–14 mesh particle size and 4.5% adhesive content showed the optimal strength values, including a CS of 14.67 MPa and SHS of 371.50 N. The CBPs sample showed excellent physical and mechanical properties compared to other commercial CBP products and satisfied the NPWCA standards in terms of density, MC, CS, and screw-holding strength. However, the DS and WA of CBPs need to be improved by utilizing a hot press during their manufacturing.