1. INTRODUCTION
Dendrochronology is the study of dating annual rings and using various environmental and growth-related information stored in the annual rings. There are various study areas in Dendrochronology involves several areas, such as dendroclimatology, dendroecology, dendrogeomorphology, and dendroarchaeology (Bigler and Bugmann, 2003; Choi et al., 2020a, 2020b; Ju et al., 2023; Lee et al., 2022; Park et al., 2023; Rybníček et al., 2020; Struble et al., 2020). Among these, dendrogeomorphology involves the investigation of the occurrence years of landslides, soil erosion, debris flow, and floods or the topographical changes brought about by these natural events (Alestalo, 1971; Bovi et al., 2022; Shroder, 1980). According to Shroder’s diagram (Shroder, 1978), landslides cause wounds in the xylem, expose or damage the roots, and bury or tilt the stems. Moreover, the stress generated by landslides causes traumatic resin canal or callus in the tree rings, or compression or tension wood (Šilhán, 2021). Based on these anatomical characteristics, dendrogeomorphological studies are done mostly in Switzerland and Czech Republic (Šilhán, 2020; Stoffel et al., 2013), but not in Korea yet.
A landslide occurs when soil mass loses its balance and collapses due to gravity. The landslide is one of the major natural events that causes damage to the natural resources (Ma and Jeong, 2007; Yun et al., 2021). Most landslides in Korea are caused by heavy rain in summer and the number of landslides is increasing more and more due to abrupt changes in climate, such as local heavy rain (KFS, 2022). Since large or small landslides occur frequently in the National Park areas, studies of landslides are still continuing after it started in 2007 (Jeong et al., 2016; KNPRI, 2022; Ma and Jeong, 2007). According to a study, the damaged area in the Jirisan National Park was 143,524 m2, which is the 2nd largest damaged area after Seoraksan in Korea (KNPRI, 2022). By monitoring a wide variety of topographical parameters, namely slope gradient, aspect, vertical slope, cross slope, altitude, forest type, parent rock, soil depth, and landslide position, width, length, and area, it was verified that most landslides in the Jirisan National Park occurred at relatively lower altitudes and in conifer forests compared with the other national parks (KNPRI, 2020).
The area of the Jirisan National Park is approximately 483 km2, the largest one in Korea (KNPRI, 2019). In particular, the subalpine conifer area is approximately 46 km2, accounting for 9.4% of the park area, which is the second largest one in the national parks (KNPRI, 2016). Aerial images have revealed that the area of conifer species decreased from 16.1% to 13.8% over 20 years (Kim et al., 2019), and the population of dead Abies koreana increased 2–5 times (Park et al., 2020). In the mountain areas of southeastern Alaska, USA, it was reported that landslides increased 3.8 times due to decline in growth of yellow cedar (Chamaecyparis nootkatensis) in the area. Decay of tree roots, which renders the soil unstable, is considered the principal reason for landslides (Johnson and Wilcock, 2002; Preti, 2013). Based on such study, the enhanced tree mortality due to climate change was suggested as a parameter that induces landslides.
In the present study, we present the relationship between the timing of landslides and the death year of conifers in the landslide areas in the Jirisan National Park. Moreover, the growth vitality of the dead trees, when they were alive, was also investigated to improve our understanding of the mortality reason.
2. MATERIALS and METHODS
Among thirteen landslide areas in the Jirisan National Park, two locations were selected for the study where the conifer species were dominantly distributed. The two locations are located at Chibatmok (site ID: LSJR01-01-01) and Jangteomok (site ID: LSJR02-04-02; KNPRI, 2017) where the landslide occurred in 2011 (Fig. 1). The distribution of tree species was verified using a vegetation map (KNPRI, 2016) and artificial intelligence to monitor the dead trees there (KNPRI, 2021). The topographical characteristics of the study sites are metamorphic rock in the parent rock, mountain collapse type in the landslides, and debris flow in external appearance (Table 1). The slope gradients of Chibatmok and Jangteomok were 33.1 and 32.9 degrees, respectively. The Chibatmok is the second largest landslide site of area 20,128 m2 and the Jangteomok is the sixth of area 5,011 m2 among the 54 landslide sites distributed in thirteen areas.
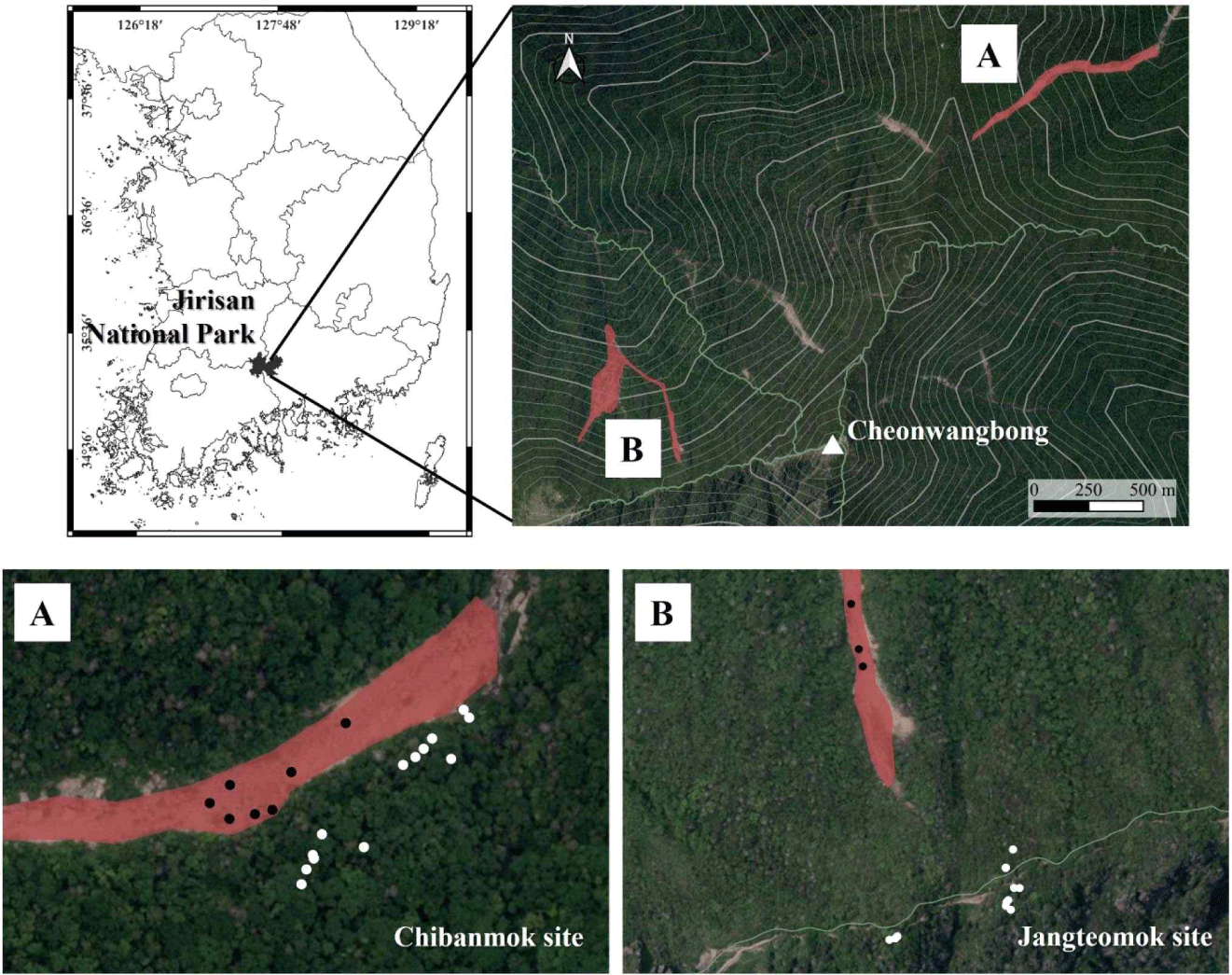
The vegetation of Chibatmok is dominated by A. koreana. Most of the dead trees in the site were identified as A. koreana based on the shape and bark. To verify the differences between the occurrences of landslide and the tree death, increment cores were acquired from seven dead A. koreana within the landslide site. Additionally, to establish the site chronology for dating the death years, increment cores were extracted from fourteen living trees close to the site (Table 2). On the other hand, the vegetation of Jangteomok is dominated by A. koreana and Picea jezoensis. Same as above, two increment cores were extracted from each dead A. koreana and P. jezoensis within the site, and eight and five cores from living A. koreana and P. jezoensis to establish the site chronologies, respectively. Among the dead trees, only limited numbers were selected due to dangerous site condition to reach the trees and no good bark condition was found.
The dead trees selected in the landslide sties were from the fallen ones as their roots were exposed, which is a typical damaged form by landslide (Šilhán, 2021). The tree-ring samples from the dead trees were collected as disks using a chainsaw at the height of 1 m from the boundary between the stem and root, whereas, the samples from living trees were collected using an increment borer (Ø5.12 mm) at breast height from the ground; 2 increment cores from each living tree. When the increment cores from the living trees were extracted, the direction was decided along the contour direction to avoid compression of wood due to slope (Schweingruber, 1988).
The increment cores were mounted on U-shaped bars so that the cells were vertically aligned, and the cross-sections were sanded using a belt sander. The entire cross-section of the disks collected from the dead trees was also sanded using a belt sander. After sanding all the samples, the annual ring boundaries were confirmed under a stereomicroscope (SMZ18, Nikon, Tokyo, Japan), while the ring widths were measured using a RINTAB (Rinntech, Heidelberg, Germany, measurement unit: 0.01 mm). To avoid errors due to measurement direction, the ring widths were measured very carefully by measuring the short distance between two adjacent ring boundaries.
In dendrochronology, cross-dating is based on the principle of limiting factors. The principle states that when the same species grow in the same environment, they share similar growth patterns due to the influence of the same limiting factors. Cross-dating refers to finding false and missing rings based on synchronization between time series graphs created with the annual ring width of each tree and assigning an accurate growth year to each annual ring. Additionally, assigning a year to a tree ring whose year of death or logging is not certain by checking the pattern of synchronization with the local master chronology for which the year is certain is also called cross-dating.
As a method to calculate pattern synchronization quantitatively, t-value [Equation (1)] and G value [Equation (2)] are used (Baillie and Pilcher, 1973; Eckstein and Bauch, 1969). In general, if the t-value and G value in dendrochronology are 3.5 and 65% or more, respectively based on a 100-year length, cross-dating done is considered statistically significant and successful; however, the final decision is made by checking synchronization with the naked eye (Kim, 2003).
Where r is the correlation coefficient between the individual ring-width time series and n is the number of overlapped years.
If (xi+1 – xi) > 0, Gix = +1/2, (yi+1 – yi) > 0, Giy = + 1/2, (xi+1 – xi) = 0, Gix = 0, (yi+1 – yi) = 0, Giy = 0, (xi+1 – xi) < 0, Gix = – 1/2, (yi+1 – yi) < 0, Giy = –1/2
Where G(x,y) is the G-value and xi and yi are the measurement ring-width values for the ith year.
The death year was confirmed through cross-dating between the master chronology and individual time series of dead conifers. After determining the death year, the season of death was also derived from the wood cells observed in the outermost tree ring. The season of death was determined as spring to summer if only earlywood cells were observed in the outermost annual ring, late summer to autumn if latewood cells were observed, whereas autumn of the current year to spring of the following year if completed latewood was observed. If incompletely developed latewood cells were observed, whereas autumn of the current year to spring of the following year if completed latewood was observed (Fig. 2). When latewood cells are observed, but the outermost cell wall is not fully thickened, it is part of latewood cells. When outermost latewood cells are fully thickened and have clear boundaries, the latewood is completed and that is called a tree ring (Seo et al., 2021).
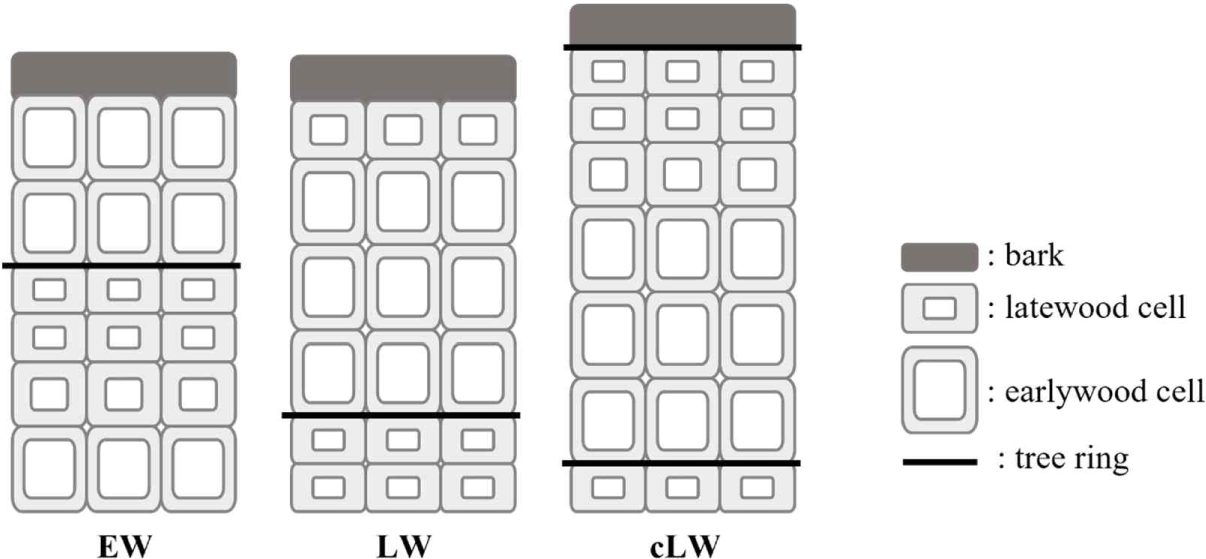
To investigate the growth vitality, the monitoring of abrupt growth reduction technique was applied to this study. The abrupt growth reduction was determined from the annual ring width. If the annual ring width decreases by more than 40% compared to the previous year and the decrease continues for more than 3 years, it is taken as a growth reduction (Schweingruber, 1988). To confirm the intensity of growth reduction, the growth reduction was classified into three levels based on the degree of decrease in annual ring width. A decrease in annual ring width compared to the previous year was considered ‘low’ if it is 40%–55%, ‘middle’ if it is 56%–70%, and ‘extreme (high)’ if it is more than 71% (Seo et al., 2019).
3. RESULTS and DISCUSSION
To date the death year of the dead trees, the site chronologies of Chibatmok and Jangteomok were built using individual ring-width time series which were cross-dated with each other. The t-value and G value between the individual ring-width time series and the chronology of A. kroeana at Chibatmok were 8.6 (2.0–14.8) and 75% (67–82), respectively. These values verified that the cross-dating was statistically successful (results not in shown). The mean t-values and G values of P. jezoensis and A. koreana at Jangteomok were 7.0 (0.1–12.9) and 4.0 (0.8–6.6), and 69% (60–74) and 71% (55–81), respectively. Therefore, the cross-dating at Jangteomok was also statistically successful. To visually check the success of cross-dating, the synchronization between individual tree-ring time series and the corresponding site chronologies were compared and the patterns were recognized as matching (Fig. 3). Using the cross-dated ring-width time series, 137-year (1885–2021) long chronology of A. koreana from Chibatmok [Fig. 3(a)], and 65-year (1957–2021) long and 364-year (1658–2021) long chronologies of A. koreana and P. jezoensis from Jangteomok, respectively, were built [Fig. 3(b) and (c)].
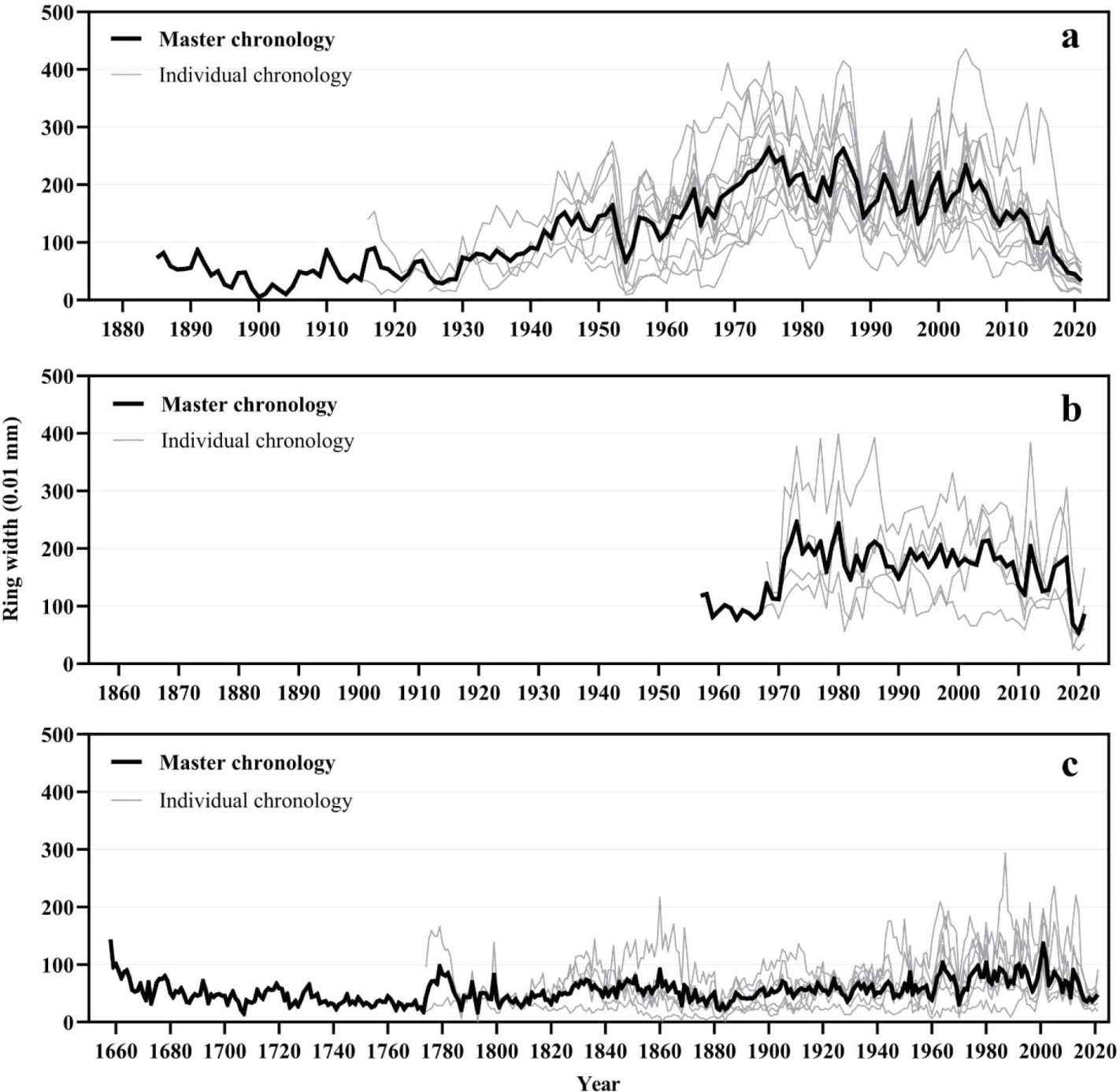
Among the 7 dead trees collected from Chibatmok, 5 were A. koreana and the other 2 were Taxus spp. and Fraxinus spp. Therefore, cross-dating to date the death year was conducted with only A. koreana which has the site chronology. By comparing the individual ring-width time series of dead trees with site chronology, the death years were successfully verified between 2012 and 2014 (Table 3). Although the t-values of 2 trees (JCBKD05 and 07) were low, their annual variation patterns were synchronized up to higher than 60%. Furthermore, presence of earlywood in the outermost tree ring of JCBKD05 and 07 and incomplete latewood in the outermost tree rings of JCBKD02 and 04 signified that the death seasons were between spring and early summer for the former and between late summer and autumn for the latter.
Among the 4 dead trees at Jangteomok, 2 were A. koreana and 2 were P. jezoensis. Comparing the individual ring-width time series of the dead trees with the corresponding site chronologies, it was verified that the death of A. koreana and P. jezoensis occurred during 2014–2021 (Table 4). According to t-value and G value, the synchronization patterns between the individual ring-width time series and the corresponding chronologies were higher than those at Chibatmok. Based on the development of wood cells in the outermost tree ring with bark, it was verified that P. jezoensis died between late autumn and the spring of the next year and A. koreana between spring and early summer.
The dead trees at Chibatmok and Jangteomok fell with their roots exposed within the landslide-affected area. Tree roots absorb moisture and inorganic nutrients from the soil. The roots particularly act as carbohydrate reservoirs from where the stored carbohydrates are utilized in various processes, such as vigorous cell division areas (buds, root meristems, cambium, fruits, etc.), energy supply for metabolic activities, and conversion into storage materials like starch (Pallardy, 2010). The present study found that death at the Chibatmok and Jangteomok sites occurred during 2012–2014 and 2014–2021, respectively. Therefore, the death would be the result of the root exposure by the landslide in 2012. According to a previous study, the death of trees at landslide sites occurred in the same year of the landslide or within maximum 3 years of the landslide (Šilhán, 2017). The results from Chabatmok were akin to the cases of the previous study. However, the death of the trees at Jangteomok begun 3 years later than the landslide and ceased after 10 years. A possible cause for the experimental trees at Jangteomok to be able to grow for the next 10 years even after root exposure might be the trees could use the nutrients stored in their roots or it depended on their vitality degree. What has to be considered further, the trees can fall due to an additional landslide after the landslide in 2011.
Out of the 14 living A. koreana in Chibatmok, all but two showed abrupt growth reduction at least once during their living (Fig. 4). Generally, 29% trees displayed growth reduction in 1950s and 71% in 2010s. Although most trees showed growth reduction in 2010s, the growth conditions for a couple of years before the landslide in 2011 were normal. The abrupt growth decline occurred mainly after the landslide. The dead A. koreana within the landslide area showed stronger growth reduction than the living ones and 1 (JCBKD06) of 5 trees died before the landslide (Fig. 5). Except the dead tree, however, the trees did not exhibit any growth reduction in 40 years prior to the landslide.
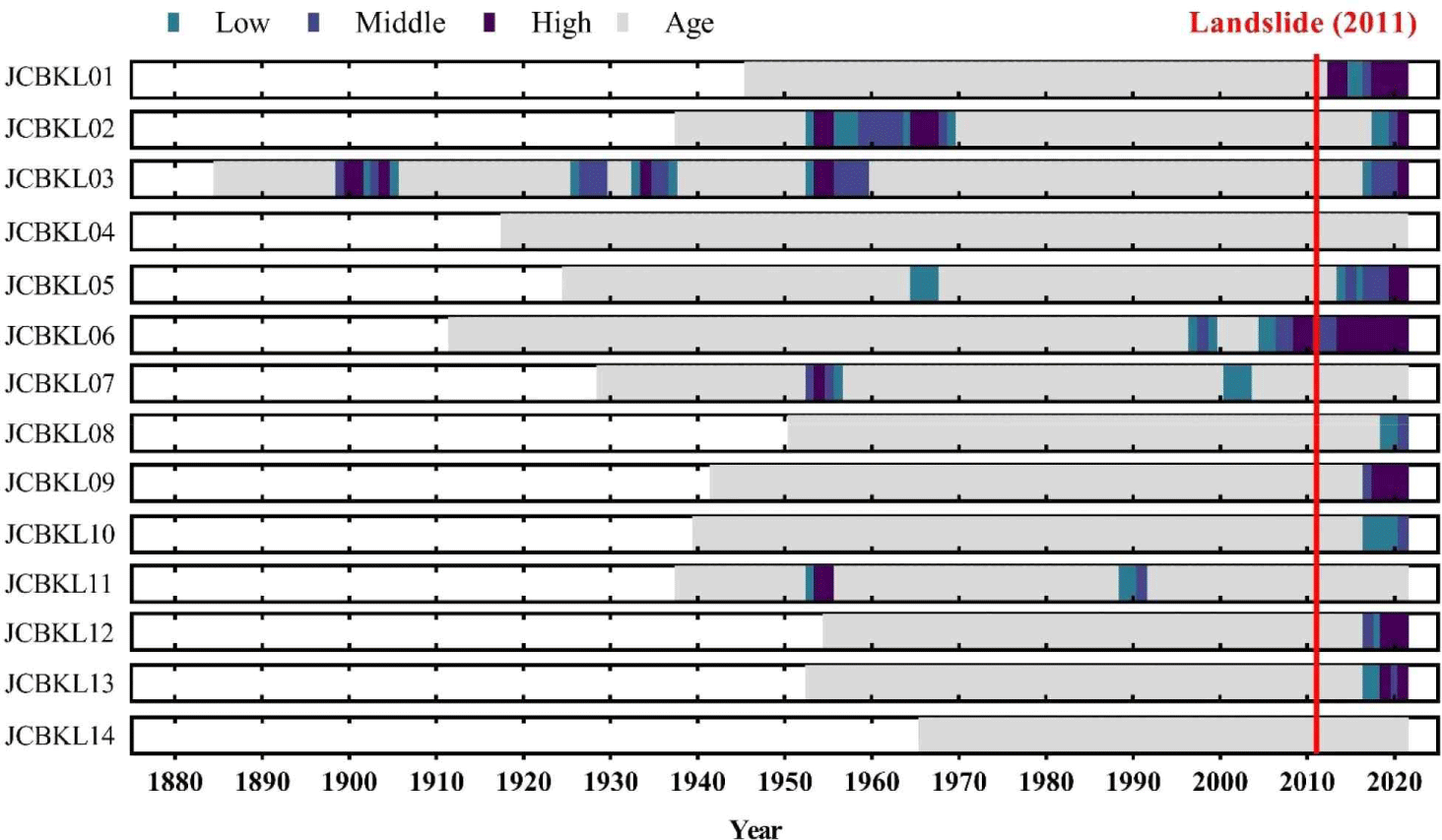
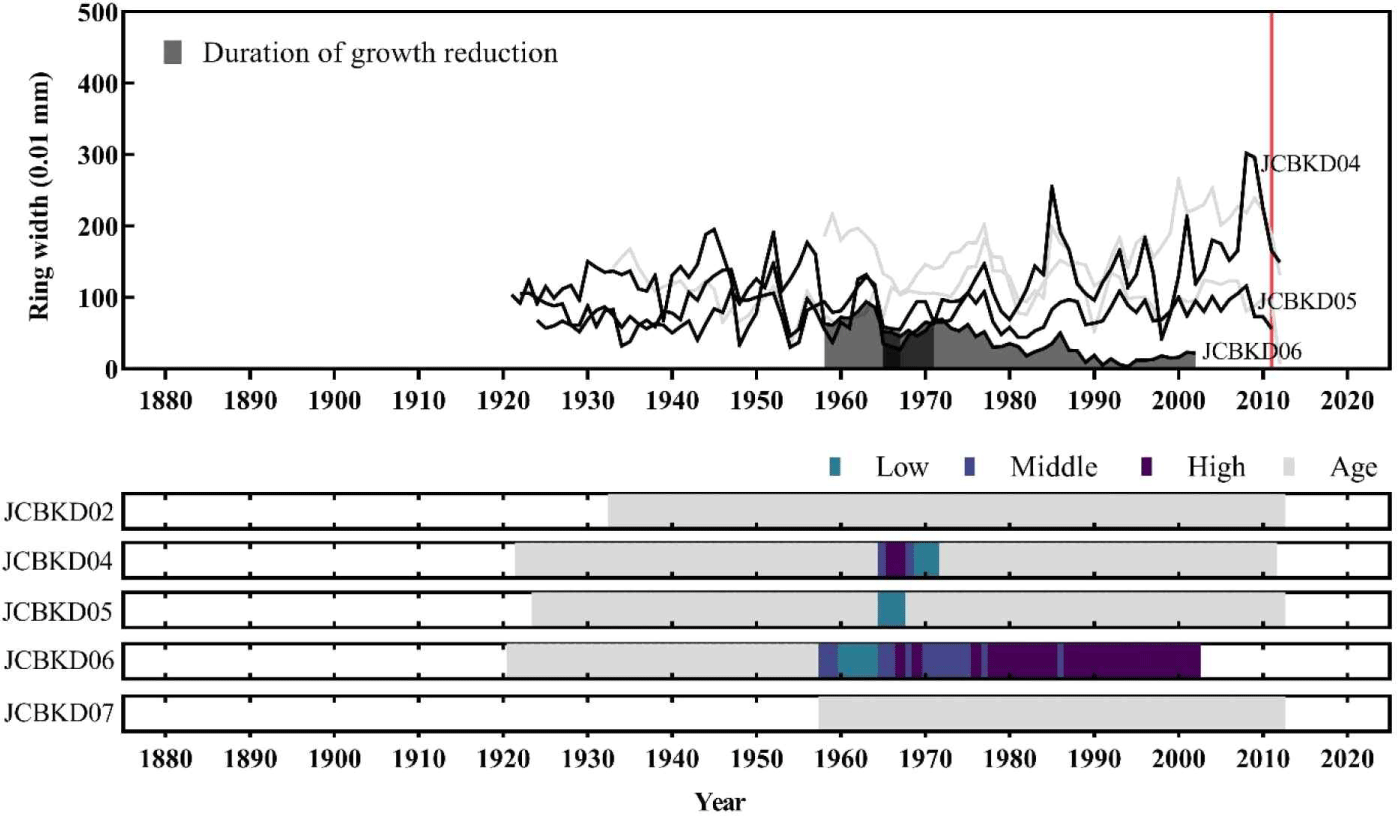
In Jangteomok, the living P. jezoensis showed more growth reduction than A. koreana before the landslide in 2011, although the growth reduction in recent years occurred more in A. koreana (Fig. 6). Moreover, except one A. koreana, the other living trees showed the growth reduction at least ones during their living. On the other hand, among the 4 dead trees, one from each species showed abrupt growth reduction while they were alive, whereas the other 2 showed none during their living (Fig. 7).
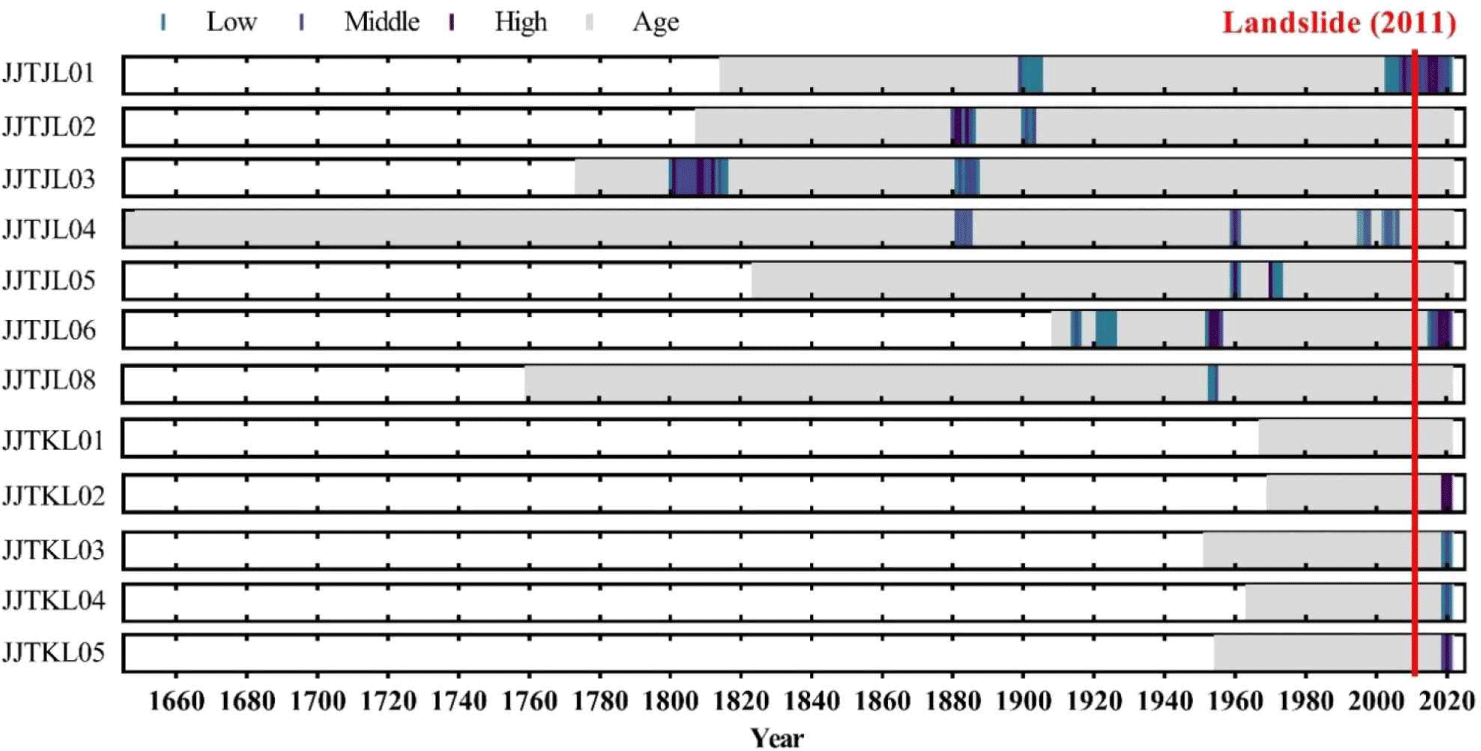
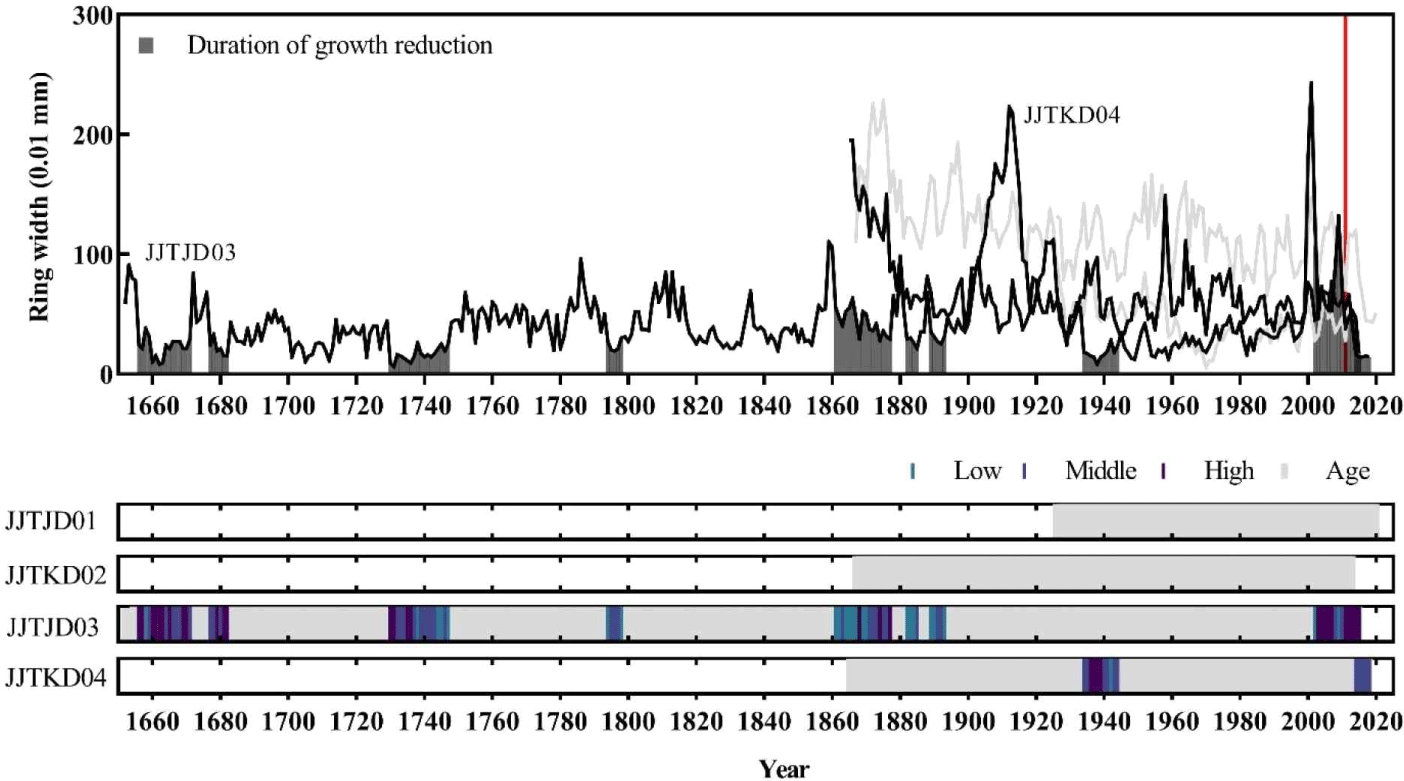
Overall, the living trees near the landslide sites at Chibatmok and Jangteomok showed lower abrupt growth reduction than the dead ones at the landslide locations. This can be justified that a landslide occurs where the growing condition is not good. Under such poor growing conditions, root development is not adequate to stabilize and hold the soil. It was also found in the present investigation that the growth of most living and dead trees was very sound before the landslide in 2011.
4. CONCLUSIONS
To conclude, the current study revealed how the occurrence of landslide can be verified using tree-ring analysis technique. The study found that although the growth vitality of the P. jezoensis and A. koreana near the landslide points at Chibatmok and Jangteomok were better than the vitality at the landslide sites, the growths of the living and dead trees were very favorable for more than a decade before the landslide. Based on the analysis results, it can be concluded that the death of the trees at the landslide sites happened due to the landslide. The finding that the death of the trees occurred 1 to 9 years after the landslide would play an important role in determining the year of any possible landslide event using the tree-ring analysis technique. The possibility that death could happen due to additional landslides should be considered to estimate the year of landslide happening. To develop further related research in Korea, more sufficient samples and/or various landslide areas need to be investigated.