1. INTRODUCTION
In marine salvaged wood, such as in mudflat environment, the primary components like cellulose, hemicellulose, and lignin are decomposed while the voids are filled with moisture (NRIMCH, 2013). Therefore, to minimize contraction and maintain the shape of the wood, the conservation treatment aims to substitute the moisture within the voids with conservation agents (Lee et al., 2011). However, in shipwrecks using iron nails, degradation products were observed during or after the conservation process, leading to subsequent research due to the recognition of their presence.
These degradation products are generated by factors introduced by burial environment and the humid conditions post-excavation. The burial environment for waterlogged archaeological wood typically fosters anaerobic conditions, allowing certain microbes to proliferate. Among these, sulfate-reducing bacteria (SRB) are prevalent in sediment, particularly in marine sediment (Hwang et al., 2008). SRB utilize sulfate (SO42–) as an electron acceptor, and saccharides, amino acids, and dissolved organic matter as electron donors for respiration (Menert et al., 2004). They produce hydrogen sulfide (H2S), which accumulates in wood (Fors et al., 2014; Monachon et al., 2020; Pecoraro et al., 2023). Additionally, if iron artifacts are present, soluble iron (III) interacts with hydrogen sulfide to form iron sulfide, which accumulates in the wood (Wetherall et al., 2008). While SRB are classified as anaerobic, they can maintain an active dormant state in aerobic environments and resume growth under anaerobic conditions, indicating they can also be aerotolerant (Le Gall and Xavier, 1996; Ramel et al., 2015).
Exposed iron in humid environment reacts with sulfur irons and hydrogen sulfide to form iron sulfide [FeS; Table 1, (1)], which, when oxidized in high-humidity conditions, forms ferrous sulfate (FeSO4) and sulfuric acid (H2SO4), contributing to degradation [Table 1, (2)–(4)]. In such humid environments, the combination of iron and sulfur leads to the formation of degradation products, resulting in degradation [Table 1, (1) Kim, 1987; (2) Thiel et al., 2019; (3) Santos et al., 2016; (4) Kılıç et al., 2023].
Fe2+ + S2– → FeS | (1) |
FeS + H2S → FeS2 + H2 | (2) |
FeS2 + 7/2O2 + H2O → Fe2+ + 2SO42– + 2H+ | (3) |
FeS2 + 7/2O2 + (n+1)H2O → FeSO4 · nH2O + H2SO4 | (4) |
Such degradation products, caused by iron compounds, leads to oxidization and acid hydrolysis of organic matter, as well as crystallization, resulting in damage to the wood cell structure (Pelé-Meziani et al., 2023; Wetherall et al., 2008). Iron ions, when crystallized through various chemical reactions, adversely affect the wood (Almkvist and Persson, 2006). Iron (II) ions induce cellulose decomposition and oxidation via the Fenton reaction, leading to cracking and fragility in the wood, while the oxidation of sulfides generates sulfuric acid, causing further damage (Almkvist and Persson, 2006; Fors and Sandström, 2006; Pelé-Meziani et al., 2023; Sandström et al., 2002). Iron sulfate compounds within the degradation products exhibit yellow, orange, or white colors and, in inconsistent humidity, can damage cell and wood surfaces through volumetric expansion (Pecoraro et al., 2023). Furthermore, they can induce the hydrolysis of polyethylene glycol (PEG), a reinforcement treatment agent for waterlogged archaeological wood, leading to the generation of low-molecular-weight acids like formic acid or oxalic acid (Almkvist and Persson, 2011).
Similar degradation phenomena have been observed not only in Korea but also in foreign shipwrecks such as the Vasa in Sweden, the Mary Rose in the UK, and the Batavia in Western Australia (NRIMCH, 2022).
Studies analyzing these shipwrecks reported that degradation products form in humid environments and corrode the wood by oxidizing cellulose, among other factors (Almkvist and Persson, 2006, 2008, 2011; Fors and Sandström, 2006; Fors et al., 2014; Kim, 1987). Various methods, such as chelating agents (Sandström et al., 2004), bacteria (Monachon et al., 2019), and nanoparticles (Taglieri et al., 2020), are being researched to mitigate this damage.
Iron sulfide can only remain stable in environments with relative humidity below 60% (with RH 50% or lower being more ideal) at around 25°C, making it difficult to stabilize without environmental control despite conservation treatments (Howie, 1978). Therefore, until effective removal methods for degradation products are developed, environmental control remains the primary solution to mitigate degradation diffusion (Kim, 1987).
As such, this degradation phenomenon caused by iron compounds in waterlogged wood has been introduced both domestically and internationally. In Korea, research on waterlogged wood includes chemical composition analysis (Kim and Kim, 2020; Kim et al., 1990; Seo et al., 2020), microbiology (Cha et al., 2022; Lim and Kim, 2022, 2023), species and dating estimation (Nam and Kim, 2021; Yoon et al., 2011), and conservation treatments (Oh et al., 2022; Park et al., 2021). However, cases of investigating degradation products inside and outside waterlogged wood from ancient shipwreck, except for the Shinan shipwreck, are rare in Korea. Therefore, this study analyzed the Shinan, Jindo, Yeongheungdo, Sibidongpado, and Wando shipwrecks salvaged by the national Research Institute of Maritime Heritage (Table 2). The Shinan and Jindo shipwrecks are Chinese vessels with iron nails, resulting in the presence of iron ions within the wood, and they have completed PEG reinforcement treatment. The Yeongheungdo shipwreck, dating to the Unified Silla period in Korea, has iron corrosion penetrating the wood due to loaded iron artifacts and is currently undergoing desalination treatment. The Sibidongpado and Wando shipwrecks are Korean vessels from Goryeo period, constructed using wooden nails without iron, thus no degradation products from iron ions were observed. They were used as comparative groups, and PEG reinforcement treatment has been completed. Samples were collected from these five ancient shipwrecks to compare and analyze the condition of both the interior and exterior wood, as well as the accumulated degradation products, aiming to create foundational data on degradation products in waterlogged wood.
Shinan shipwreck | Yeongheungdo shipwreck | |
![]() |
![]() |
|
Jindo shipwreck | Sibidongpado shipwreck | Wando shipwreck |
![]() |
![]() |
![]() |
2. MATERIALS and METHODS
Samples were collected from the surfaces (exteriors) and interiors of the shipwrecks’ materials among the ancient shipwrecks salvaged by the National Research Institute of Maritime Heritage, including the Shinan, Jindo, Yeong-heungdo, Wando, and Sibidongpado shipwrecks: The Shinan and Jindo shipwrecks, which used iron nails and exhibited signs of degradation, the Yeongheungdo shipwreck, covered in iron corrosion due to the presence of iron artifacts, and the Wando and Sibidongpado shipwrecks, which did not use iron nails and instead utilized wooden nails. However, in the case of the Shinan shipwreck, samples were collected from areas where degradation was not observed on the surface (SAN) to compare interior degradation with areas where degradation was observed (SAD; Tables 3 and 4).
Shinan shipwreck | Yeongheungdo shipwreck | |
![]() |
![]() |
|
Jindo shipwreck | Sibidongpado shipwreck | Wando shipwreck |
![]() |
![]() |
![]() |
Samples from the Shinan, Wando, and Sibidongpado shipwrecks were cut from remaining pieces after conservation into 1 × 1 × 1 cm sizes for both surface and interior collection. Samples from the Yeongheungdo and Jindo shipwrecks were collected using the coring method, which combines a Haglof increment borer (Sweden) and a core bit, to obtain cores with a diameter of 6 mm and a length of up to 8 cm.
The samples collected for component analysis were ground to below 100 μm using an Agate Mortar, and analysis was conducted after categorizing each shipwreck into specific sections.
For the analysis of major components, Wavelength Dispersive X-ray Fluorescence Spectrometry (WD-XRF, XRF-1800, Shimadzu, Kyoto, Japan) was commissioned to the center for research facilities of Pukyong National University. The analysis was performed with an acceleration voltage of 40 kV and a current of 70 mA. Prior to analysis, the samples were glass-bead fused, and the results were expressed as semi-quantitative analysis.
Elemental content analysis was conducted using inductively coupled plasma atomic emission spectrometer (ICP-AES, Optima 8300, PerkinElmer, Waltham, MA, USA) at the Korea Basic Science Institute. Sample pre-treatment involved acid digestion, and silicon (Si) was not measurable due to the use of a small amount of hydrofluoric acid during pre-treatment. The analysis was conducted with an R.F. frequency of 40.68 MHZ and 27.12 MHZ, and R.F. power of 1,300 W.
Additionally, X-ray diffraction (XRD) was performed to analyze the compound state using an X-ray diffractometer (EMPYREAN, Malvern Panalytical, Almelo, The Netherlands). The analysis was conducted with a 2theta range of 3–90 degrees, a scan speed of 1 sec/step, and a step size of 0.03 degrees, with a voltage of 40 kV and a current of 30 mA. Copper was used as the target during analysis, and the peaks were identified using X’Pert HighScore program after measurement.
To observe the cellular tissue and distribution of inorganic elements within the cellular tissue, the following procedures were conducted.
Samples were prepared by mounting them in epoxy resin and sequentially grinding them from 220 mesh to 4,000 mesh. Subsequently, fine griding was carried out using 3 μm and 1 μm (DP-Spray, Struers, Ballerup, Denmark). Once the fine grinding was completed, the samples were observed using an optical microscope (Axioplan 2, Carl Zeiss, Oberkochen, Germany) to examine the cellular tissue.
For detailed observation of the cellular tissue, the analysis samples were coated with gold (Au) and observed using a scanning electron microscope (SEM, JSM-IT800, JEOL, Tokyo, Japan). The chemical composition of the cellular tissue was analyzed using energy dispersive spectrometer (EDS, ULTIM MAX 100, Oxford Instruments, Oxford, UK).
3. RESULTS and DISCUSSION
The samples from the Shinan, Yeongheungdo, Jindo, Sibidongpado, and Wando shipwrecks were subjected to XRF, ICP-AES, and XRD analyses to determine the composition and quantitative values of major components within the samples and to identify the forms of compounds present. For the composition ratios and quantitative values, three samples were analyzed, and the results are presented as averages. The findings are as follows.
The analysis of component composition ratios revealed that in Shinan, Yeongheungdo, and Jindo shipwrecks, where the influence of iron ions was observed, the iron and sulfur composition ratios accounted for over 80%. In contrast, in Sibidongpado and Wando shipwrecks, where no signs of degradation were observed, the iron and sulfur composition ratios ranged from approximately 35% to 61%. In addition to iron and sulfur, soil components such as calcium (Ca), silicon (Si), and aluminum (Al) were also detected (Table 5, Fig. 1).
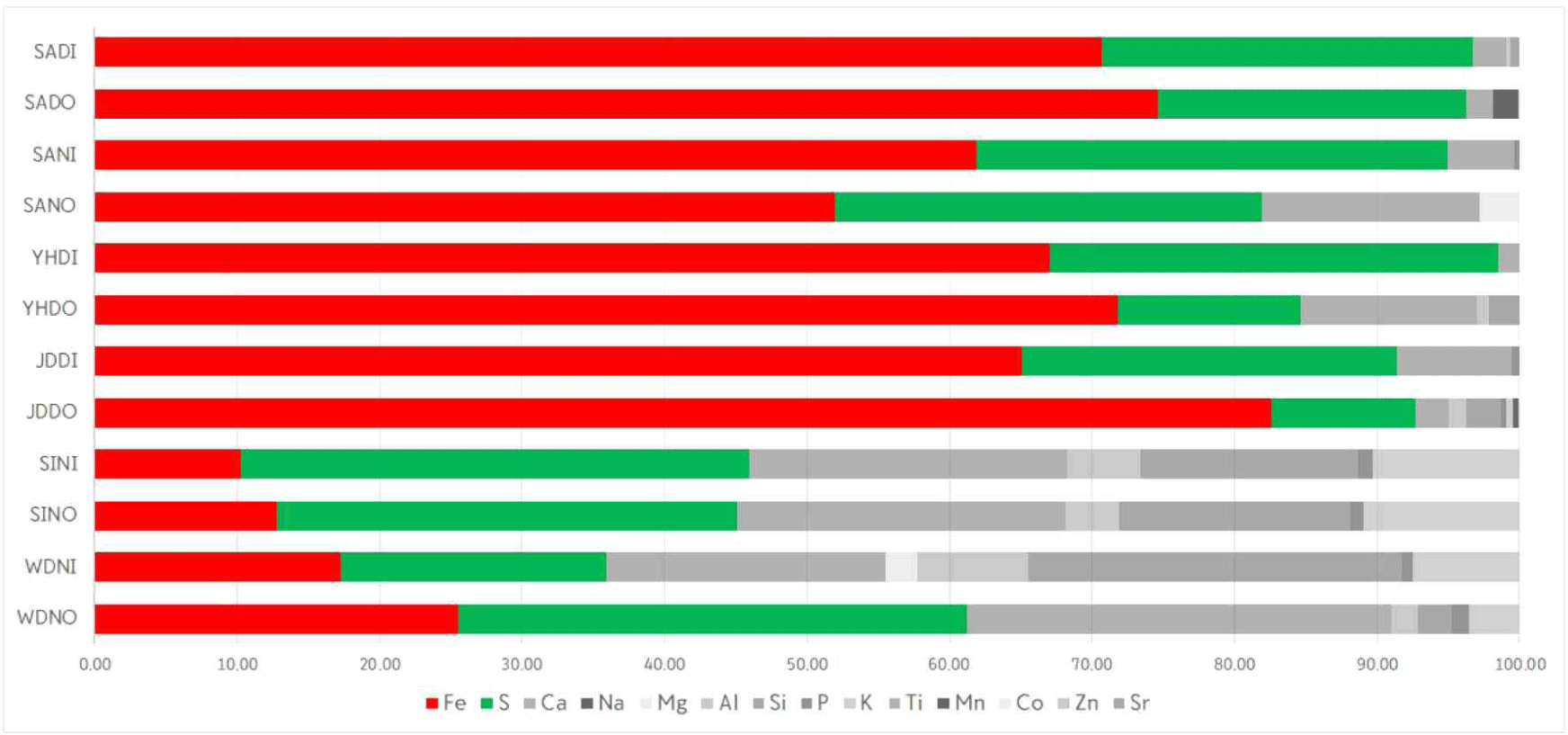
In most of the shipwrecks where degradation was observed, the ratio of iron was higher externally than internally, while sulfur showed higher ratios internally than externally. Particularly in the case of the Jindo shipwreck, the iron composition ratio externally was more than 17% higher compared to internally. Additionally, in the Shinan shipwreck, there was not a significant difference in the internal and external composition ratios in both degradation areas (SAD) and lightly degradation areas (SAN).
Even in shipwrecks such as Sibidongpado and Wando where no degradation was observed, the presence of sulfur suggests its accumulation in the burial environment due to hydrogen sulfide generated by the metabolic action of sulfate-reducing bacteria, as previously identified in research (Fors et al., 2014; Monachon et al., 2020; Pecoraro et al., 2023).
The elementary analysis results showed that iron and sulfur were primarily detected in all samples, with calcium, aluminum, magnesium, manganese, sodium, and other soil components also identified (Table 6, Fig. 2). The elements other than iron and sulfur were categorized as “Others” in both the tables and the figures.
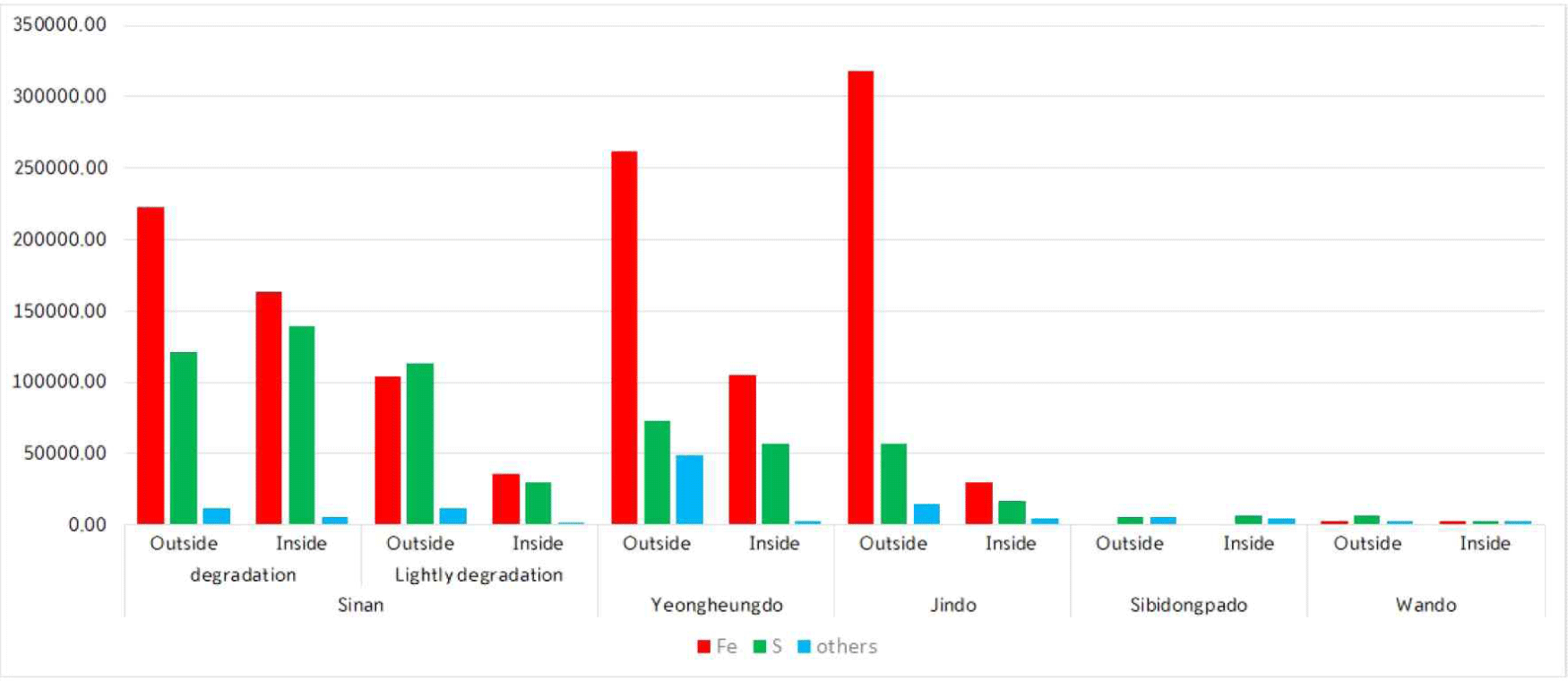
Iron and sulfur were labeled as “Fe + S” and were found to range from 30,820 to 529,744 ppm in shipwrecks where degradation products or degradation factors were present (Shinan, Yeongheungdo, Jindo), while in shipwrecks where no degradation was observed (Sibidongpado, Wando), they ranged from 4,014–14,657 ppm, indicating a significant difference depending on the presence of degradation factors (Fe, S) (Table 6). The Fe/S ratio compared the amounts of iron and sulfur, showing higher values when iron was predominant compared to sulfur. Particularly in shipwrecks influenced iron, such as Shinan, Yeongheungdo, and Jindo, the ratio fluctuated externally due to higher iron content but remained consistent internally, showing a ratio between 1 and 2. However, in shipwrecks unaffected by iron, such as Sibidongpado and Wando, most ratios were below 1 (Table 6, Fig. 2).
The mineral content in the Shinan shipwreck degraded areas showed approximately 1.2 times more material externally than internally, while in areas of slight degradation, the external material was about 3.4 times more abundant. Both external and internal areas in degraded regions showed substantial amounts of Fe and S. However, in the lightly degraded areas, Fe was detected approximately 2.9 times higher, and S was detected approximately 3.8 times higher in the external samples than the internal samples indicating observed differences between internal and external conditions due to the degradation (Table 7).
The Yeong-huengdo shipwreck had over twice as much external mineral content compared to internal content, with “Others” being approximately 22 times higher due to ongoing desalination treatment. The difference between internal and external sulfur content was relatively small, about 1.3 times. (a difference of 15,844 ppm; Table 7).
In Jindo shipwreck, external iron content was the highest among all samples at 317,637 ppm, approximately 10.6 times higher than internal content. The ratio of iron and sulfur was about 8 times higher than the interior, indicating a significant difference compared to Shinan and Yeongheungdo shipwrecks (Table 7).
For the shipwrecks made with wooden nails like Sibidongpado and Wando, sulfur content ranged from 2,241–6,665 ppm, lower than in shipwrecks where degradation products was observed. However, sulfur accumulation due to sulfur-reducing bacteria was observed in all hulls (Table 6, Fig. 2).
Shinan shipwreck exhibited the presence of various iron compounds such as iron sulfide, iron sulfate hydrate, iron carbonate, and iron oxide, with sulfur observed both internally and externally. Among them, calcium sulfate hydrate was observed in the external samples of the degraded area. Additionally, various inorganic compounds such as silicon oxide were observed both internally and externally (Table 8).
Yeongheungdo and Jindo shipwrecks showed the presence of iron oxide, iron oxide hydrate, and iron sulfide, with Jindo shipwreck exhibiting external iron compounds but only sulfur internally, suggesting limited penetration of iron compounds internally (Table 8).
Sibidongpado and Wando shipwrecks showed the presence of PEG 4000, indicating the preservation treatment remained intact. Although sulfur was observed in Wando shipwreck, the minimal presence of iron prevented the observation of iron compounds (Table 8).
To examine the distribution patterns of iron (Fe) and sulfur (S) as degradation factors between the surface and the interior of the hulls, optical microscopy was employed, while the distribution of Fe and S within the cells were observed using scanning electron microscopy. Primarily, scanning electron microscopy observations and EDS analyses were conducted focusing on the parts observed with optical microscopy, and mapping was performed to confirm the distribution of inorganic substances (Tables 9 and 10). The colors of major elements are consistent with those listed in Table 11.
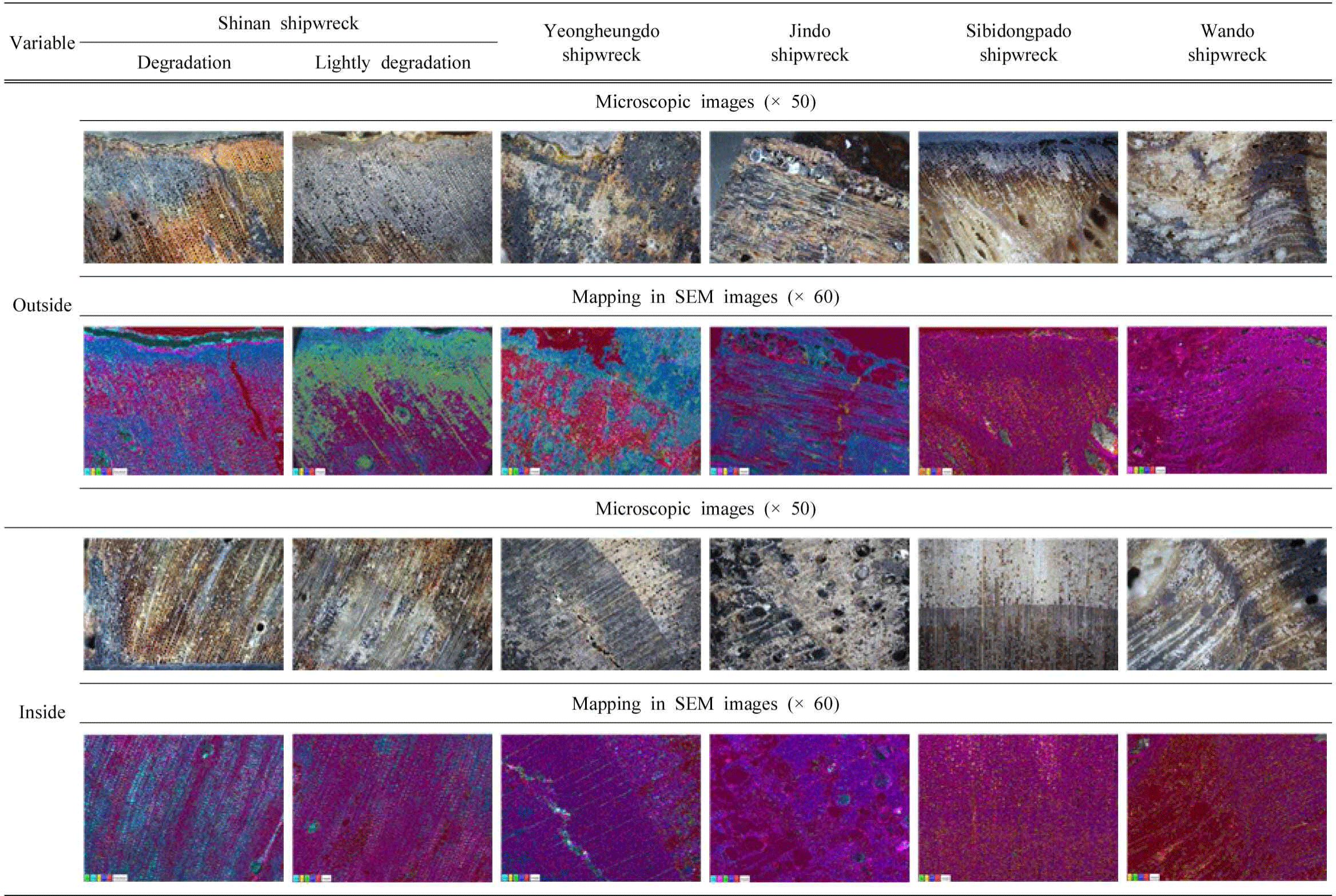
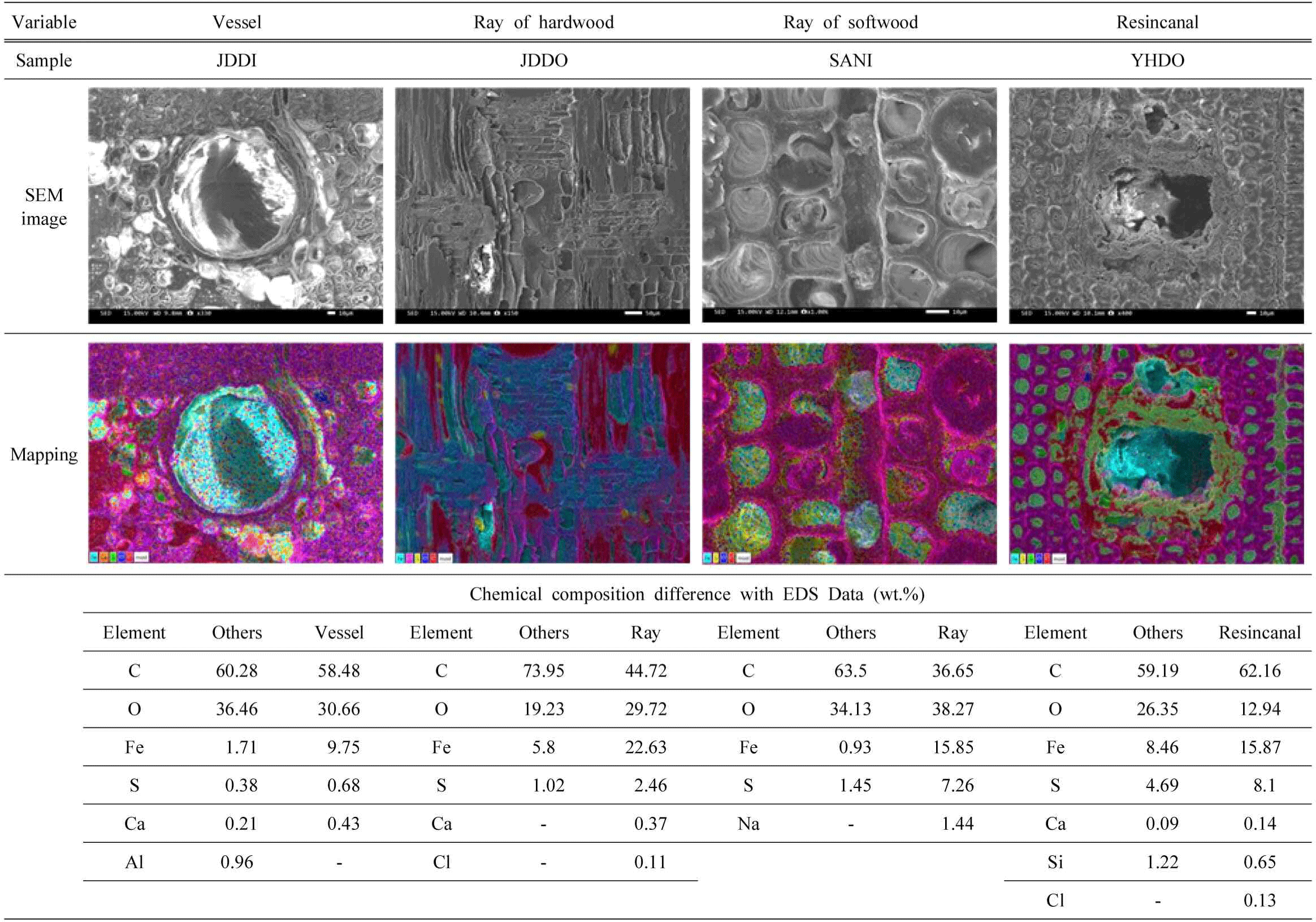
Element | C | O | Fe | S | Si | Ca |
---|---|---|---|---|---|---|
Color | Red | Blue | Sky blue | Yellow | Light green | Orange |
Optical microscopy revealed that the wood tissues of the shipwrecks Shinan, Yeongheungdo, and Jindo exhibited a reddish-brown color resembling the color of rusted iron, unlike the predominantly dark coloration due to PEG treatment in the woods of Sibidongpado and Wando shipwrecks. This coloration was consistent both externally and internally. Based on this, EDS analysis and mapping were conducted (Table 9). Dark and reddish-brown areas observed under optical microscopy were confirmed to contain abundant iron and sulfur, indicating color changes due to compounds of iron and sulfur. Regardless of the degree of degradation, Iron and sulfur were predominantly distributed near the surface, extending into the interior. However, internal sections of Shinan showed relatively fewer detections of iron, and almost no iron and sulfur were detected within the internal sections of Jindo shipwreck.
EDS analysis of the cell structures of Sibidongpado and Wando shipwrecks revealed sulfur within the cell lumina, while the outermost black coloration observed under optical microscopy was confirmed to be a carbonized surface, with no detection of other inorganic components such as iron or sulfur.
Using scanning electron microscopy, detailed observations of the distribution of iron and sulfur within the cell structures of the wood were conducted (Table 10). In coniferous woods, iron compounds were detected along the tracheid walls, along with minor detections of sulfur and calcium. Abundant iron and sulfur, along with trace amounts of calcium, were observed in the surrounding wood fibers and the lumina of ray tissues through mapping. Within the rays, iron was observed within the ray parenchyma and other cell lumina, while sulfur was primarily found in crystalline forms along the cell walls. Based on the analysis of ray tissues, it is presumed that iron and sulfur are primarily diffused through ray tissues and wood fibers. Coniferous woods exhibited similarly high ratios of iron and sulfur within the ray tissues to broadleaf woods, with the presence of crystalline structures. Furthermore, within the resin ducts and epithelial cells, iron and sulfur were predominantly detected, and they were also observed within the surrounding vessels. In samples containing degradation products, higher concentration of iron and sulfur were observed in the vessels, resincanals, and ray tissues compared to the surrounding tissues, indicating their role as major transport pathways.
Moreover, during the observation of wood tissues, crystalline compounds embedded within cell lumina were observed (Table 12). Compounds such as iron oxide (Fe2O3), iron sulfide (FeS), and iron sulfate (FeSO4) crystals were identified, particularly single-component sulfur crystals were noted. Crystalline forms of iron compounds were observed in Shinan, Jindo, and Yeong-heungdo shipwrecks, while in Sibidongpado and Wando shipwrecks, due to the lower iron content compared to the other vessels, occasional crystals of calcium sulfide (CaS) and calcium sulfate (CaSO4) were presumed in the cell walls rather than iron-sulfur compounds.
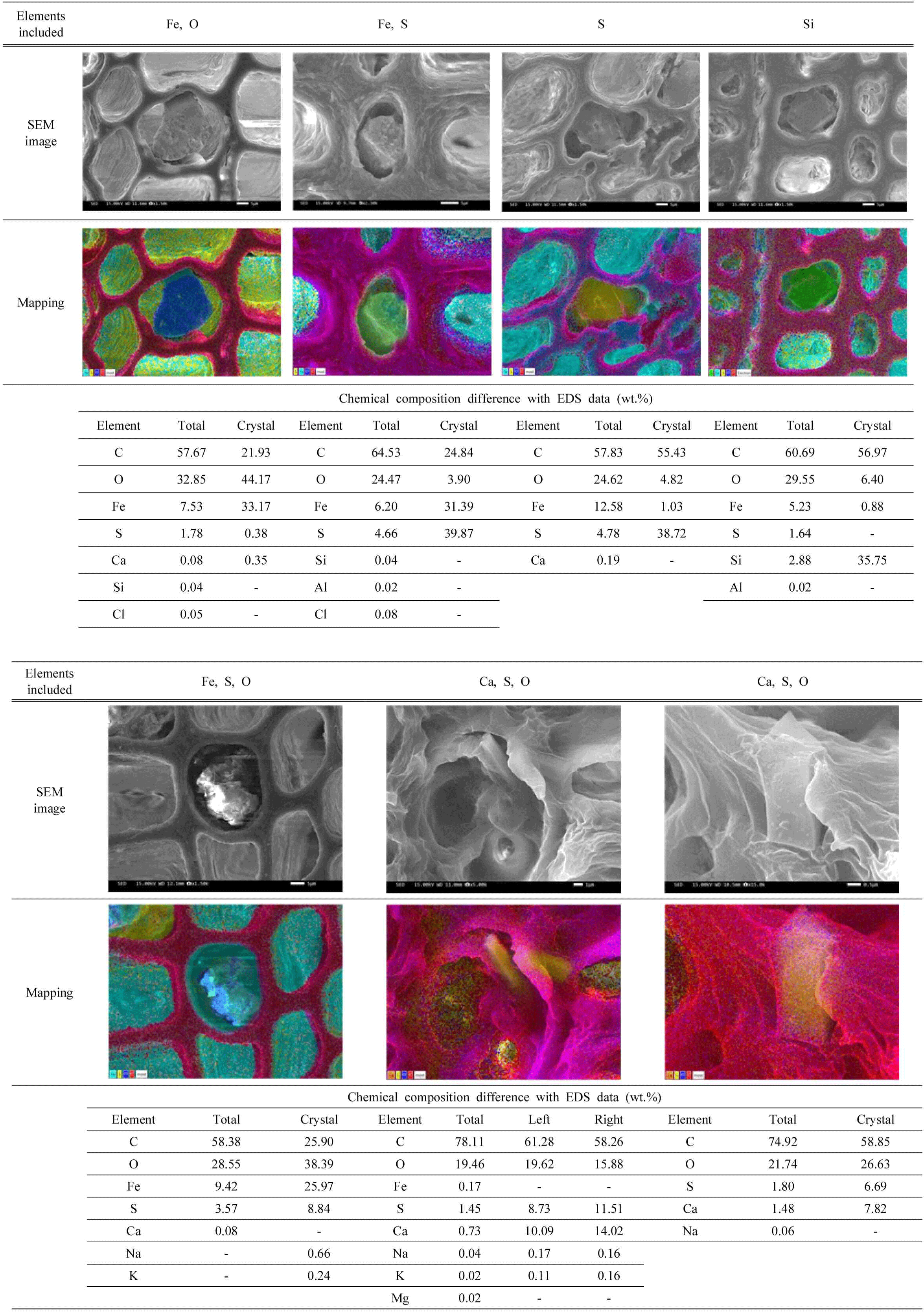
This study investigated the condition of both the interior and exterior of wooden structures and observed degradation products in five salvaged ancient shipwrecks: Shinan, Yeongheungdo, Jindo, Sibidongpado, and Wando. Despite variations in composition, sulfur and iron were present in all samples. Particularly high concentration of sulfur and iron was observed in Shinan and Jindo shipwrecks, where degradation products were present. Indeed, the presence and accumulation of sulfur can be closely related to the burial environment. In the case of ancient shipwrecks that are buried and salvaged in anaerobic environments, the activity of predominant sulfur-reducing bacteria in such anaerobic conditions enables the accumulation of sulfur inside the wood during the burial. This accumulation, especially in Shinan, Jindo, and Yeongheungdo shipwrecks, may be attributed to the influence of iron-oxidizing bacteria (IOB) and sulfur-reducing bacteria, which may synergize to facilitate sulfur accumulation.
The higher sulfur and iron content in these shipwrecks compared to Sibidongpado and Wando may be due to the synergistic effect of IOB and sulfur-reducing bacteria stimulated by iron release, resulting in decreased oxygen concentration and the creation of anaerobic conditions (Scotto et al., 1985). Furthermore, the combined culture of these bacteria leads to more vigorous activity (Sung et al., 2008) and increased corrosion production (Xu et al., 2007). This suggests that iron is a significant factor in sulfur and iron accumulation and a crucial factor in degradation. Additionally, Yeongheungdo, despite being salvaged from an aerobic environment, accumulated considerable sulfur due to the presence of abundant iron, suggesting a substantial influence of iron oxidizing bacteria. The significantly lower concentration of iron and sulfur in Sibidongpado and Wando shipwrecks compared to those with observed degradation products such as Shinan, Jindo, and Yeongheungdo can be attributed to the environment created by active sulfur-reducing bacteria.
The sulfur and iron ratios in the shipwrecks with observed degradation products showed similar proportions, with higher concentrations on the surface. Observation of cross-sections revealed blackening and reddish-brown discoloration, along with the presence of degradation-related crystals, except the internal samples of Jindo shipwreck. The outermost blackening observed in Sibidongpado and Wando was attributed to carbonization due to a lack of other inorganic components such as iron and sulfur. The carbonization is presumed to have aimed to adjust the curvature of wooden components (NRIMCH, 2010) or to carry out surface charring treatment for insect and corrosion prevention purposes (Cultural Properties Administration, 1985) during the shipbuilding process.
Shinan was divided into degraded and lightly degraded areas based on the presence of degradation products on the surface. Even in the lightly degraded areas without surface degradation, iron and sulfur concentrations were as high as the degraded areas, with a more significant presence externally than internally. Furthermore, the presence of degradation products such as iron sulfide and iron sulfate both internally and externally indicates that degradation was progressing, particularly within the interior of the wood. Yeongheungdo showed high external iron due to iron corrosion products from iron artifacts, suggesting significant iron penetration into the interior. Jindo shipwreck exhibited similar trends to Yeong-heungdo, with high external iron content and notable differences between internal and external concentrations. Being constructed from campo tree, Jindo shipwreck may not have allowed iron penetration into the exterior due to its unique wood structure (Lee, 1997). Sibidongpado and Wando, without observed degradation products, had lower iron and sulfur content. The wood’s original coloration remained unchanged on the cross-section, with minimal detection of iron, suggesting the absence of iron compounds. However, the presence of sulfur led to the observation of crystals combined with calcium.
Higher concentrations of iron and sulfur were observed in resin ducts, tracheids, and ray tissues, indicating the migration of degradation factors through warm water flow or PEG during desalination and reinforcement treatments. During the reinforcement treatment phase of preservation processes, especially in the case of ancient shipwrecks, the wood is often impregnated with PEG, facilitating the movement of iron and sulfur along with it. Therefore, it is essential to remove iron before proceeding with preservation treatments. Otherwise, the diffusion of iron, a degradation factor, during the reinforcement treatment may actually widen the scope of degradation.
Hydrogen sulfide (H2S) accumulated in the hull reacts with iron (Fe) to form iron sulfide (FeS), leading to the generation of iron sulfate (FeSO4) and sulfuric acid (H2SO4) in humid environments, inducing wood degradation. The presence of significant sulfur and iron indicates ongoing degradation. For instance, in the semi-degraded sections of Shinan, the internal concentration is approximately 60,000 ppm, and even in the interior of Jindo shipwreck, where penetration of degradation products is difficult due to the wood’s anatomical characteristics, it is approximately 40,000 ppm. This suggests that if the concentration exceeds 40,000 ppm, degradation is indeed occurring. However, as this is just the beginning of foundational research and data is still limited, continued and consistent research efforts will lead to the accumulation of results. Establishing thresholds for iron and sulfur degradation through this research would enable proactive preventive conservation treatments. Additionally, the lower iron content relative to sulfur in Sibidongpado and Wando suggests that removing iron until its concentration decreases relative to sulfur may be chemically stable during re-treatment.
4. CONCLUSIONS
This study investigated the internal condition of marine salvaged ancient wooden shipwrecks, focusing on five vessels: Shinan, Jindo, Yeongheungdo, Sibidongpado, and Wando. While Shinan and Jindo, where iron nails were used, showed signs of degradation products, Yeongheungdo exhibited penetration of iron corrosion into the wood due to the presence of iron artifacts. In contrast, Sibidongpado and Wando showed minimal evidence of iron compounds.
The presence of sulfur accumulation in waterlogged wood was confirmed, with vessels exhibiting degradation factors such as Shinan, Jindo, and Yeongheungdo showing higher mineral content compared to Sibidongpado and Wando, which showed minimal degradation products. This suggests that the presence of iron determines the formation of degradation products, indicating that iron is a key factor in wood degradation. Further research is needed to determine the combined effects of sulfur-reducing bacteria and iron-oxidizing bacteria on sulfur accumulation.
Additionally, higher concentrations of iron and sulfur were observed in resincanals, vessels, and ray tissues, and compounds in crystalline forms were identified on the cell walls, suggesting the migration of degradation factors along with warm water flow or PEG during desalination and consolidation treatments, necessitating iron removal before preservation.
While the phenomenon of degradation in waterlogged wood due to iron compounds has been documented, studies on the composition of degradation products in waterlogged wood from Korean ancient shipwrecks have been limited mainly to Shinan. Therefore, this study intends to utilize foundational data from various vessels to establish guidelines for degradation progression and propose reprocessing methods based on the analysis of degradation products in waterlogged wood.