1. INTRODUCTION
Currently, the supply of wood from plantation forests in Indonesia is dominating nearly 90% of the wood processing industry (KLHK, 2019). Owing to the impact of the high rate of deforestation in recent decades and the increasing need for wood in society, wood originating from these plantation forests has been developed to be harvested in a relatively short rotation period (Badalamenti et al., 2020). However, limited technological advancements in the field of sylviculture have caused this fast-growing wood to have poor physical and mechanical properties. Furthermore, wood from these plantation forests is generally not resistant to biologically degrading organisms such as termites and fungi. Hence, wood processing technologies are required to improve the properties of fast-growing wood species.
Several studies have been conducted and applied on a laboratory scale, and some have even been realized industrially to improve the properties of wood, particularly those derived from fast-growing species. In addition to the conventional preservation methods that have been used for decades, wood modification methods using high-temperature heating techniques (wood thermal modification) and wood chemical modification techniques have been widely studied. Moreover, a few techniques have been applied industrially (Gérardin, 2016; Ghani and Lee, 2021; Jones and Sandberg, 2020; Laksono et al., 2023; Lee et al., 2021; Mantanis, 2017; Militz, 2015). Environmental issues have become one of the main reasons for the development of wood modification technology.
Impregnation of wood with styrene monomers is a chemical modification technique that has been extensively studied (Budiman et al., 2019; Hadi et al., 2015, 2021; Hadjib, 2005; Hartono et al., 2009; Syamani et al., 2012). Although this modification is a passive modification of wood, meaning that it does not have a major influence on the chemical components of the wood, it was developed to make wood have properties such as plasticity and better physical and mechanical properties. Chao and Lee (2003) reported that the density, water repellence, and hardness of southern pine increased after styrene impregnation. Ibach and Rowell (2013) also reported that polystyrene-impregnated wood has better hardness, compression and shear strengths, bending and cleavage strengths, and improved resistance to wear, especially abrasion resistance, than untreated wood. Budiman et al. (2019) studied five Indonesian wood species and reported that polystyrene-impregnated wood improved the moisture content, density, water absorption, shrinkage-swelling, modulus of rupture, modulus of elasticity, and hardness of untreated wood.
In addition to improving the physical and mechanical properties of wood, polystyrene-impregnated wood can increase its resistance to biologically degrading organisms such as fungi and termites. Hadi et al. (1998) investigated four polystyrene-impregnated woods from Poland with a weight-percent gain average of 113%. After the baiting process for one month to dry-wood termites in the laboratory and another durability test for one year to subterranean termites in the field, the results showed that the weight losses due to dry-wood and subterranean termites for untreated wood were 1.38% and 100%, respectively, whereas for polystyrene-impregnated wood were 0.1% and 19%, respectively. In other words, the polystyrene-impregnated wood exhibited better resistance than the untreated wood. For further verification, several years later, Hadi et al. (2016) also studied three polystyrene-impregnated wood species from Indonesia with a weight percent gain average of 14% and then exposed three months in the field against subterranean termites. The weight loss values of untreated and polystyrene-impregnated wood were 47% and 9%, respectively, indicating that polystyrene-impregnated wood has much more resistance than untreated wood. Similarly, Acosta et al. (2020) impregnated Brazilian fast-growing pinewood with polystyrene and then baited to Coptotermes curvignathus subterranean termite using a no-choice test, and the results showed that polystyrene-impregnated wood was better than untreated wood for mass loss and feeding test in the laboratory test.
With the rapidly increasing technology in the wood processing industry, fast-growing wood, which generally has a small diameter, can still be processed into engineered wood products, such as glulam. Glulam, formed by combining small-diameter wood with a gluing system, is widely used in the industries as a substitute for large-diameter wooden blocks/beams. However, as previously mentioned, owing to the basic nature of the fast-growing wood, which is generally not resistant to biological degrading organisms, especially termites (Arinana et al., 2012), one of the biggest threats in Indonesia as termite damage to wood is the most difficult to detect with naked eyes (Lee and Kim, 2022), impregnation of fast-growing wood using monostyrene is an alternative technique that can be applied for making glulam.
Recently, our group implemented the impregnation of monostyrene into wood to manufacture glulam. The developed polystyrene-impregnated glulam provided better physical and mechanical properties than solid wood in terms of high density, low moisture content, high hardness, and equal shear strength (Hadi et al., 2021). A laboratory test against subterranean termite attacks revealed that these polystyrene-impregnated glulams had higher termite resistance than solid wood and control glulams (Hadi et al., 2022a). Because these glulams have not been exposed to subterranean termites in the field, the main objective of this study was to test the durability of polystyrene-impregnated glulams made of tropical fast-growing wood species, namely, mangium wood, manii wood, and rubberwood, against subterranean termites in the field (grave-yard test).
2. MATERIALS and METHODS
Three tropical woods, mangium (Acacia mangium), manii (Maesopsis eminii), and rubberwood (Hevea brasiliensis), were collected from less than ten years of forest stands in Bogor, West Java, Indonesia. The logs diameter ranged from 15 cm to 20 cm, and then flat sawn to be laminae with size of 1.7 cm (thickness) × 5.5 cm (width) × 50.0 cm (length). All laminae were kiln-dried to reduce moisture content to approximately 12%. and then the faces of the boards were planned, and afterwards, the laminae were cut to be two pieces along their length. The laminae were prepared for manufacturing the glued laminated lumber (glulam). The main modifying agents (styrene monomers) and potassium peroxydisulfate (catalyst) were purchased from TokoFRP Jakarta and PT. Merck Indonesia Tbk, Jakarta, Indonesia, respectively. The adhesive (water-based polymer isocyanate) was in the form of resin (PI-127T), a milky white viscous liquid (5,000–15,000 cP at 23°C) with a 40%–44% solid content and pH 6.5–8.5. While for the curing agent, we used hardener (H–3M), dark brown liquid (viscosity 150–250 cP at 23°C) with a 98% solid content. Both the compounds were supplied by PT Polychemical Asia Pacific Permai (Jakarta, Indonesia).
The laminae of each wood species were placed in a tank and then vacuumed at 600 mmHg for 30 min, submerged in a styrene monomer containing a catalyst (potassium peroxydisulfate; 100/1 w/w), followed by a pressure of 10 kg/cm2 for 30 min (Hadi et al., 2021, 2022b). The impregnated samples were then removed from the vacuum pressure apparatus. They were then placed on a rack to remove the excess impregnating solution. The impregnated wood was wrapped with aluminum foil, followed with a polymerization process at 80°C for 24 h. The weight percentage gain (WPG) of each wood sample was measured as described previously (Hadi et al., 2022a). To ensure that polystyrene entered the voids of the wood, a scanning electron microscope (SEM; Zeiss-Evo 50) was used to identify the presence of polystyrene in the wood in our previous study (Hadi et al., 2021). The images were captured using a SEM set to 10 kV.
At this stage, there were two types of laminae: polystyrene-impregnated and untreated laminae. Both laminae types were prepared to manufacture a three-ply glulam by composing three laminae parallel along the longitudinal grain direction. The polyurethane glue spreading rate used was 280 g/m2 for each glue line. The use of the isocyanate-based polyurethane adhesive was based on its better bonding performance than that of the non-isocyanate-based polyurethane adhesive (Song and Kim, 2022; Sumardi et al., 2022). The laminae were then cold-pressed at 10 kg/cm2 for three hours. All glulams of each species (manii, mangium, and rubberwood), and each product (control glulam, and polystyrene-impregnated glulam) were conditioned in a room (temperature 25°C–30°C, and RH 80%–90%) at least two weeks. For comparison purposes, each solid wood sample was also prepared with the same dimensions as the glulam. The appearance of the tested samples before the field tests is shown in Fig. 1. Before the wood products were tested in the field, their density and moisture content were measured, as described by Hadi et al. (2022a).
After conditioning process, six glulam as replication of each wood species and wood product were exposed to the termites in an arboretum (6°33’27.4”S 106°43’46.7”E) belonging to the Faculty of Forestry and Environment, IPB University, Bogor, Indonesia, for four months. The dimensions of the glulam samples used for the field tests were 5 cm (thickness) × 5 cm (width) × 25 cm (length). The glulam samples were randomly stacked 20 cm vertically along the longitudinal grain direction on the ground. After the field test, the weight loss and protection levels of each glulam sample were calculated according to Hadi et al. (2022a). The field conditions at the start and end of the test are shown in Fig. 2. The recorded climate from October 2021 to January 2022 of the test site in terms of relative humidity, solar radiation, and rainfall were 84.4 ± 4.9%, 4.8 ± 2.5 h/d, and 283 ± 205 mm/month, respectively (Bureau of Meteorology, 2022). Referring to the Schmidt–Ferguson classification, this location was classified as A rainfall type. Fig. 3 shows an ombrothermic diagram for the test period (Bureau of Meteorology, 2022). The conditions described in Fig. 3 are suitable for termite survival. In addition, the arboretum (test area) is covered by high tree canopies, limiting the intensity of sunlight reaching the ground (Arinana et al., 2020).
After four months of field-testing, the tested samples were removed from the site, cleaned, dried at 103°C, and weighed. The weight losses of the tested samples were determined using Equation (1) (Priadi et al., 2023). For protection levels, the method was based on our previous work (Hadi et al., 2016, 2022a) according to Table 1, which is analogous to ASTM D 1758-06 (ASTM, 2006).
where W1 and W2 are the dried weights of the samples before and after the field tests, respectively.
Two species of subterranean termites were found at the site, Macrotermes sp. and Schedorhinotermes sp., as shown in Fig. 4. Arinana et al. (2022) also found both types of termites at the same test site during a field study of various community wood species in Bogor, Indonesia. Both termite species can be clearly distinguished from soldier termites. In the soldier Macrotermes sp., the color of the soldier head is reddish brown with fontanel in the upper part, reduced marginal teeth, spread hair, and a pronotum shaped like a horse paddle (Santoso et al., 2016). The other termite, Schedorhinotermes sp., includes both major and minor soldier termites. The major soldier of Schedorhinotermes sp. is characterized by a labrum that extends to three quarters of the mandible. The second segment was shorter than the third segment, the middle part of the postmentum was narrower than the end part, the edge of the pronotum was relatively wide, and the head had a yellowish-red color (Kutana, 2021).
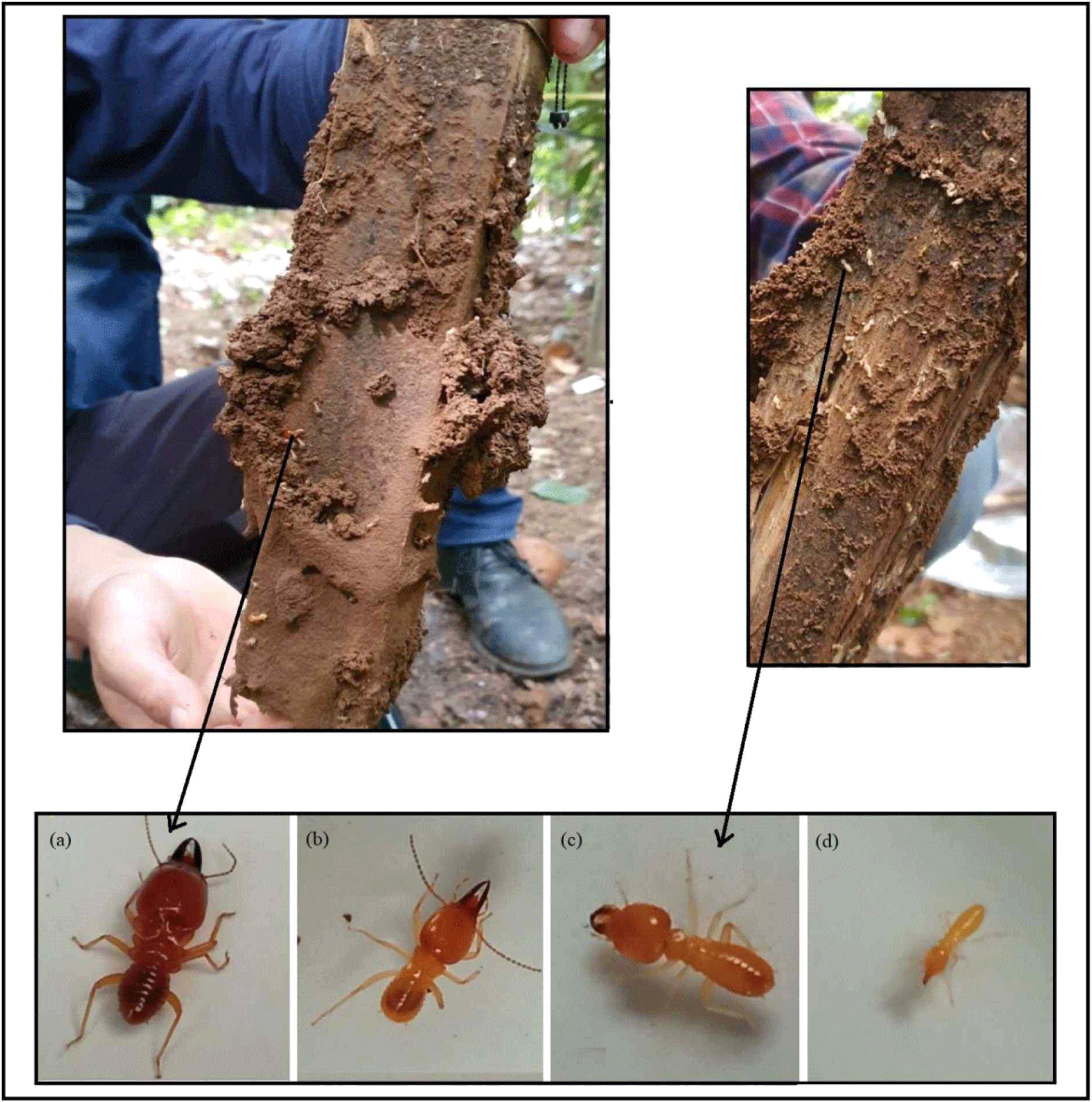
Wood species and wood products were evaluated, and numerical scores were assigned using a Likert scale (Likert, 1932), ranging from low to high, to quantify and categorize each parameter or response. A lower priority was indicated by a low score, and vice versa. Wood species are represented by letters a, b, and c, whereas wood products are represented by letters p, q, and r. These letters correspond to scores of one, two, and three, respectively. All letters were determined based on the results of Duncan’s multi-range test for each wood species and wood product. The total score was calculated by summing the scores of all parameters, including feeding rate, resistance class, termite mortality, protection level, and weight loss. A higher total score indicates a higher priority for both wood species and wood products.
A total of 54 test samples comprising glulam made from three different wood species, mangium, manii, and rubberwood, were used in this study. Each wood species was subjected to one of the three treatments: polystyrene-impregnated glulam, control glulam, or solid wood. The experiment followed a completely randomized 3 × 3 factorial design. The first factor investigated was wood species, whereas the second factor focused on wood products. Further analysis was conducted using Duncan’s multi-range test when significant differences were observed among the main factors at a significance level of p ≤ 0.05 (Hadi et al., 2021).
3. RESULTS and DISCUSSION
Before conducting the durability test in the field, the density and moisture content of each test sample were measured; the data are presented in Table 2. Based on the data, it could be stated that the density varied from 0.44 g/cm3 (solid manii) to 0.79 g/cm3 (rubberwood polystyrene-impregnated glulam), and the average density was 0.63 ± 0.12 g/cm3. According to Ashaari (2017), the solid wood of manii with a density of 0.48 g/cm3 was classified as low-density, while mangium (0.62 g/cm3) and rubberwood (0.70 g/cm3) were classified as medium-density woods.
As a result of the impregnation and polymerization of the styrene monomer in wood, all polystyrene-impregnated glulams had higher densities than solid wood and the control glulam. The polystyrene-impregnated material hardened and occupied wood voids. According to the SEM analysis in our previous study, which used the same tested samples (Hadi et al., 2021; Fig. 5), polystyrene-impregnated manii wood showed that some pores were filled with polystyrene (Fig. 5b), whereas pores in the untreated wood remained empty (Fig. 5a). This is in accordance with that observed by Stolf and Lahr (2004), who reported that styrene monomers penetrate and subsequently polymerize into polystyrene in the wood structure.
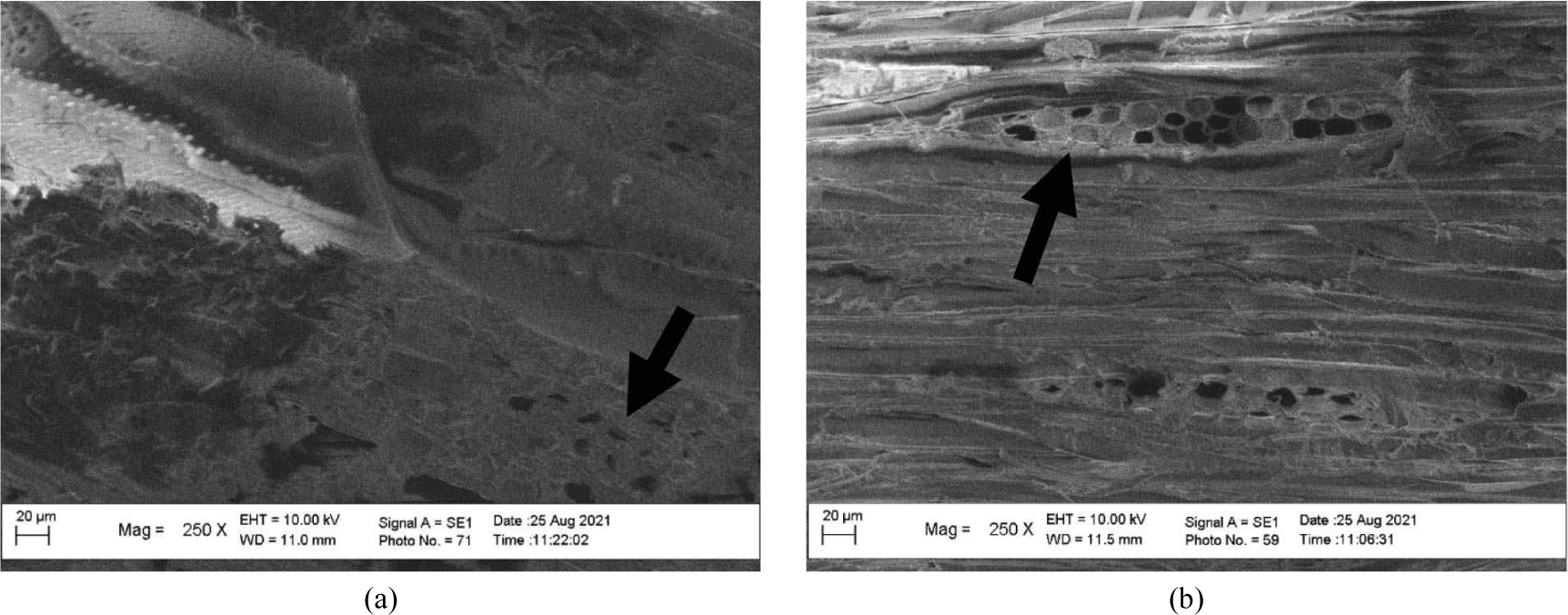
As the result of polystyrene deposit in wood, all impregnated polystyrene laminae automatically underwent weight increase; the WPG observed for manii was 18.22 ± 4.19%, mangium 22.30 ± 1.98%, and rubberwood 10.44 ± 2.09%. Owing to its high density among wood species, rubberwood has the lowest WPG value. On the other hand, manii, which has a lower density than mangium, had a lower WPG than mangium because manii has a fiber partition, interlocking fiber, aggregated radial cells, and rosin or tylosis in the void, which could be barriers for styrene monomers entering the void during the vacuum-pressure impregnation process (Muslich et al., 2013).
Referring to the variance analysis of the density in Table 3, all three factors of wood species, wood product, and interaction of both factors (wood species and wood product) highly significantly affected the density at p ≤ 0.05. The results of Duncan’s multi-range test of the interaction factor for density are presented in Table 4. The table shows that the highest density products were mangium polystyrene-impregnated glulam, followed by rubberwood polystyrene-impregnated glulam and rubberwood control glulam. In addition, it could be suggested that high-density wood has the advantage of being more resistant to subterranean termite attacks, as mentioned by Arango et al. (2006), and higher durability against subterranean termites is positively influenced by higher wood density. Moreover, polystyrene-impregnated wood has better physical-mechanical properties, as reported by Stolf and Lahr (2004), who reported that the physical-mechanical properties of Pinus caribaea impregnated with polystyrene were higher than those of untreated wood. Another study reported that aspen (Populus sp.) and fir (Abies sp.) wood impregnated with polystyrene provided a higher density and better MOE, MOR, and hardness properties than untreated wood (Hadjib, 2005). Finally, our previous study also corroborated previous research, proving that the better physical–mechanical properties of the product were positively influenced by its higher density (Hadi et al., 2021). As observed from Table 2, the lowest density products were manii control glulam, followed by manii solid wood and manii polystyrene-impregnated glulam, indicating that manii had low densities compared to the other two wood species.
Parameter | Wood species | Wood product | Interaction |
---|---|---|---|
Density | ** | ** | ** |
Moisture content | ns | ns | ns |
Weight loss | ** | ** | ns |
Protection level | ** | ** | ns |
After conditioning at room temperature for at least two weeks and referring to the data of each tested sample, it could be expressed that the moisture content ranged from 12.57% (manii polystyrene-impregnated glulam) to 15.93% (rubberwood control glulam), while the obtained moisture average was 14.07 ± 0.83%. The moisture content of all the samples was still in the range of ambient moisture content in Bogor, ranging from 10% to 18% (Kadir, 1973).
The moisture contents of all tested samples are listed in Table 2. The lowest moisture content of manii could be related to its low density, indicating that it had the lowest cell wall weight. Consequently, the bonding water content was minimal. In contrast, rubberwood has the highest moisture content because it has the highest density. However, according to the results of the variance analysis in Table 3, the wood species and wood products did not affect the moisture content of the tested samples. In other words, all tested samples reached equilibrium moisture content. More specifically, both types of glulam met the Japanese standard JAS 234-2003 (maximum 15%) for their moisture content (JAS, 2023).
After four months exposure in the tropical field, all test samples were measured for their weight loss due to degradation below the ground level, the results of which are shown in Table 5, and the conditions of each tested sample are shown in Fig. 6. Based on the data, the lowest weight loss value was 0.21% belonging to mangium polystyrene-impregnated glulam, while the highest weight loss value was 86.75% belonging to manii solid wood, and the average of all tested samples was 27.20 ± 21.85%.
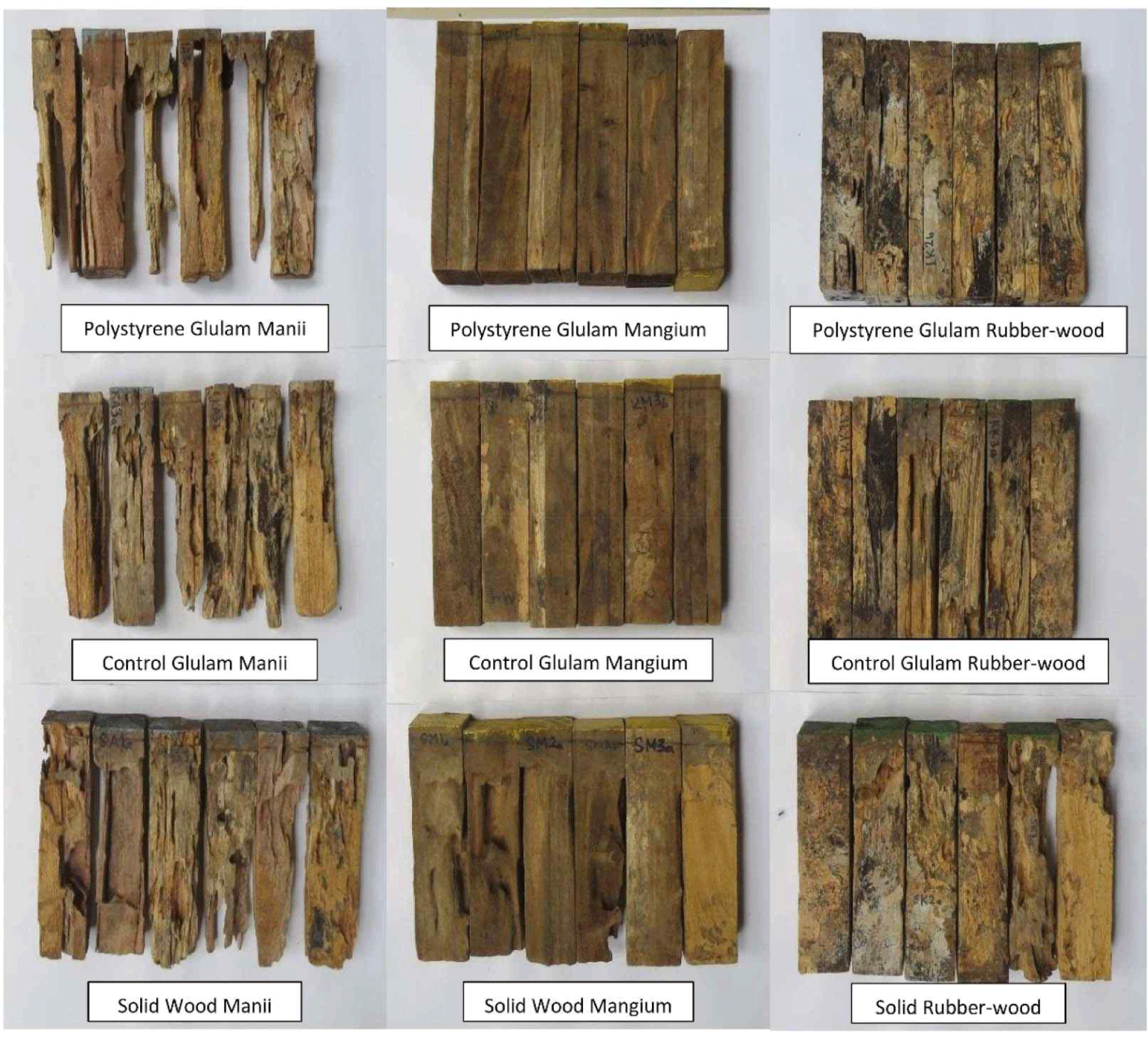
Based on the summary of the variance analysis presented in Table 3, only the wood species showed a significant difference in the weight loss of the tested samples. In contrast, wood products and interaction factors did not differ significantly. Further examination using Duncan’s multi-range test, as shown in Table 6, showed that mangium exhibited the most durable wood-to-subterranean termite attacks, followed by manii and rubberwood. The superior resistance of mangium can be attributed to its original classification as resistance class III (Oey, 1990), whereas rubberwood and manii are classified as class V (Arinana, 2012; Jasni, 2016). According to the Indonesian National Standard (2014), Class V indicates very poor resistance to subterranean termite attacks, whereas Class I represents high resistance. In addition, Mihara et al. (2005) reported that mangium heartwood contains flavonoids, such as 3,40,7,8-tetrahydroxy-flavanone, teracacidin, 40,7,8-trihydroxyflavanon, and 2,3-trans-3,40,7,8-tetrahydroxyflavanone, which possess the ability to resist fungal attacks by Pogonomyrmex badius and Phellinus noxius. These flavonoids, which may also exist in our mangium wood, may also have acted as termiticides in this recent study.
Rubberwood has poor resistance or resistance class V to subterranean termite attacks. Consequently, this wood species is frequently used for termite attacks, and as stated by Arinana et al. (2012), Pinus merkusii, Paraserianthus falcataria, and Hevea brasiliensis can be used as reference controls in tests against subterranean termite attacks following the Indonesian standard SNI 7207-2014. Although rubberwood has a very poor resistance to biodeteriorating agents, it possesses sufficient physical and mechanical properties. Teoh et al. (2011) reported that under air-dry conditions, rubberwood has a density of 640 kg/m3, modulus of rupture of 66 N/mm2, modulus of elasticity of 9,240 N/mm2, and side hardness of 4,320 N. Moreover, wood has a cream color, attractive features, and good working properties, making it attractive to the furniture manufacturing sector. Consequently, wood should be preserved before processing the furniture components.
For wood products, the weight loss of the tested samples was significantly affected by the type of product. However, it was evident that the polystyrene-impregnated glulam exhibited the lowest weight loss (23.05%), whereas the other products showed higher, 27.55% for control glulam and 31.00% for solid wood. Furthermore, Table 6 shows that the polystyrene-impregnated glulam was significantly different (p < 0.05) from solid wood in terms of the percentage of weight loss. This result is consistent with that of Hadi et al. (2015), who found that wood impregnated with polystyrene exhibited higher durability against subterranean and dry wood termite attacks than solid wood. In this study, the weight loss value of polystyrene-impregnated glulam should be much lower to get significantly different (p ≤ 0.05) from the other products. In this case, the weight percent gain of lamina caused by polystyrene impregnation should be much higher, and as stated by Hadjib (2005), a higher WPG of the three Indonesian wood species impregnated with polystyrene resulted in higher physical and mechanical wood properties.
Manii wood exhibited the highest weight loss (51.20%), followed by rubberwood with 26.09%, or about half that of manii wood, and mangium wood with 4.27%, or about 0.08 that of manii wood. The lowest density of manii compared to that of mangium and rubberwood could be the reason for its highest weight loss, corroborating the findings of Arango et al. (2006), who reported that wood with low density is more easily attacked by termites. Moreover, the samples tested in this study used young wood, which predictively had a low extractive content. Nevertheless, these three wood species are planted intensively in Indonesian plantation forests by the Indonesian government and private companies. This plantation program has been performed to fulfill logs supply, where in 2021, the production of logs in the country reached 64.42 million m3, of which 48.56% was from mangium, while the logs from the natural forest shared only in a small number (BPS-Statistics Indonesia, 2022).
Also after four months of exposure in the field, protection-level measurements of each tested sample were conducted. The protection levels of each tested sample are listed in Table 5. Regarding the analysis of variance (Table 3), the wood species and wood product factors significantly affected the protection level of the tested sample, but not the interaction of both factors.
In terms of wood species, analysis of data using Duncan’s multi-range test informed that mangium had the highest protection level with the rating score of 8.94 [following ASTM D 1758-06 (ASTM, 2006), describing that the rating score of 10 indicated no attack, whereas score 0 indicated failure], followed by rubberwood (6.72) and manii (4.94). Noted that the three wood species differed each other. Mangium had the highest protection level; in terms of resistance classification according to the Indonesian National Standard, wood is moderately resistant or belongs to class III (Oey, 1990), whereas the other two wood species are very poorly resistant or belong to class V (Arinana et al., 2012; Jasni, 2016). The higher termite resistance of mangium could be attributed to the higher phenolic compounds present in the wood (Hendrik et al., 2019).
The polystyrene-impregnated glulam provided the highest protection level with a rating score of 7.50, which was not different from that of the control glulam with a rating score of 7.00. These results indicate that the pretreatment of laminae with polystyrene impregnation did not effectively increase glulam resistance to subterranean attacks. Higher WPG of laminae impregnated with polystyrene should be achieved and improved. Furthermore, both types of glulam were different from solid wood, with a rating score of 6.11, showing that glulam manufacturing could increase product resistance to termite attacks.
As listed in Table 7, wood species and wood products have different priorities for further development. Based on the Likert scale, mangium presents the best response (the highest total score) among the wood species. However, according to the wood products, polystyrene-impregnated glulam exhibits the best response among the applied treatments. Impregnation of polystyrene into the wood has been proved to provide better durability properties to the wood products. Therefore, in terms of subterranean termite attack resistance investigated in this study, mangium can be selected as a wood species recommended for the future development of polystyrene-impregnated glulam.
From these observations, it can be concluded that mangium polystyrene-impregnated glulam was the wood product most resistant to subterranean termite attacks, followed by rubberwood polystyrene-impregnated glulam in the second place. However, according to our previous study (Hadi et al., 2021), medium-density wood is the most recommended wood for glulam manufacturing to meet the requirements of physical and mechanical properties. Based on these results, it is also recommended that the polystyrene-impregnated glulam of rubberwood and manii (both wood species belonging to medium-density woods) be processed for the further development of wood products that could provide satisfactory physical-mechanical properties and resistance to subterranean termite attacks.
4. CONCLUSIONS
The study results showed that the moisture content of all wood products was still within the range of the ambient moisture content in the Bogor area and met the standard. Manii wood, which is a low-density wood, had the lowest resistance to subterranean termite attacks compared to mangium and rubberwood, both of which are medium-density woods. Almost all glulamic wood products showed better durability against subterranean termites than solid wood products. However, there was no significant difference in termite resistance between the glulam control and polystyrene-impregnated glulam. To obtain excellent subterranean termite resistance in polystyrene-impregnated glulam, a higher WPG of polystyrene laminae through intensive polystyrene impregnation should be developed for future research. In this study, glulam from mangium and rubberwood was used to obtain maximum advantages not only in terms of resistance to subterranean termites but also in terms of physical-mechanical properties.