1. INTRODUCTION
Wood is an important construction material that has been used for hundreds of years. The material shows good properties under dry conditions and when kept from weathering and biological attack. Moreover, wood is a relatively durable construction material, and domestic wood construction contains many wood construction buildings ranging from ancient traditional architecture to modern construction.
During its lifetime, timber is susceptible to biological, chemical, mechanical, and physical degradation related to the ambient environment (Kloiber et al., 2014). After several years of exposure to environmental conditions, wood is subject to degradation that can reduce the physical and mechanical properties of wood and affect its use. Although the effect of degradation on the mechanical properties of wood is a crucial issue in timber construction, it is not well understood. An in situ assessment of existing timber structures will assist in their maintenance (Yamaguchi, 2013). As a part of an assessment of existing timber structures, Hwang et al. (2020) evaluated the wood of historical architecture in Korea. They suggested an effective method to identify wood species using synchrotron X-ray microtomography-based three-dimensional microstructural imaging.
During the assessment of existing timber structures, a diagnostic examination of the structural members and connection is generally necessary (Gonzalez et al., 2015). Methods of in-situ evaluation can be classified as destructive, semi-destructive, and non-destructive (Kasal and Anthony, 2004). Some non-destructive testing methods (NDT) have been proposed for in situ assessments of wood and shown to be very efficient methods (Ross and Pellerin, 1994). Oh (2020) reported the possibility of using NDT for an in situ evaluation of spruce lumber. NDT is also useful for the rapid screening of potential problem areas and is generally based on correlations between non-destructive parameters (such as stress wave velocity) and destructive parameters (such as strength or modulus of elasticity) (Riggio et al., 2014). Nevertheless, NDT requires member extraction, and the destructive parameters are obtained using a destructive method. In the case of an existing construction, the extraction of samples is generally impossible because of the building character, particularly in historic buildings (Jasieńko et al., 2013).
Another method for an in situ assessment of timber is semi-destructive testing (SDT). SDT bridge the gap between indirect non-destructive and direct fully destructive methods of strength measurement. SDT is helpful for determining the extent of degradation through the resistance of the material using various methods, such as drilling, cores, screw withdrawal, needle penetration, and pin pushing. The probe test as SDT through the measurement of pullout force was carried out to determine the residual strength of Pinus sylvestris and showed a good relationship between pullout force and compressive strength (Gilfillan and Gilbert, 2001). Ahn et al. (2020) reported that the withdrawal resistances of axially loaded self-tapping screws on wood products made from Korean larch were predicted with the existing estimation equation and compared with experimental test data. Lee et al. (2021) evaluated the withdrawal resistance of the screw-type fasteners. They analyzed the effects of the lead-hole size, relative grain direction (tangential, radial, and cross-sections) of the wood member, screw diameter, screw type, and species.
Several semi-destructive techniques were also summarized to test the recommendations and understand the theoretical basis, typical equipment set up, and basic capabilities and limitations (Tannert et al., 2014). The screw withdrawal force is an SDT to estimate the wood density, material strength, and shear modulus. This method is based on the correlation between the screw withdrawal force and MOR, screw withdrawal force and density, and screw withdrawal force and shear modulus. A screw withdrawal test provides a local parameter. Therefore, tests are applied to multiple locations, and the average value should be used to estimate the properties (Tannert et al., 2014). A direct estimation of the residual strength of wood in existing timber construction is difficult because the extraction of test wood samples is very limited, especially in historic wood buildings. In this case, an SDT test method would be very useful for providing an on-site estimation of the timber structure for the residual strength of wood elements. Despite several studies aimed at estimating the wood strength related to traditional wood buildings, an in-situ estimation of the strength of wood is limited to domestic wood buildings. In particular, wood decay reduces the physical and mechanical properties of wood. Park et al. (2018) estimated the decay resistance of larch wood and observed up to 5% weight loss in untreated wood. Kim and Kim (2020) tested the physical and mechanical properties of wood after one year of exposure and observed a decrease in the ultimate load in bending, but they did not mention the effects of decay damage.
This paper proposes an evaluation method that predicts the physical and mechanical properties of decayed wood, such as the density and compressive strength, through an estimation of the screw withdrawal resistance.
2. MATERIALS and METHODS
The test samples used in this study came from radiata pine lumber, 50 × 40 × 1200 mm (W × T × L) in size, decayed by insect damage. The lumber was purchased from a local sawmill and used as a woodworking project for students. After the experiment, the remaining samples were exposed to the outdoors over four years, as shown in Fig. 1(a). The damage to the sample lumber was classified into insect damage by Cerambicidae and blue stain. Samples were cut from ten pieces of lumber and processed to a size of 50 × 40 × 50 mm for compression parallel to the grain test and 50 × 40 × 150 mm for the screw withdrawal test, as shown in Fig. 1(c). Forty-two samples were prepared for each test and conditioned indoors for one week at approximately 19℃ with a relative humidity of 55%. Before testing, the test samples were weighed, and their dimensions were measured to obtain the air-dried density. The screw used in this study was 4 mm in diameter with a thread length of 65 mm.
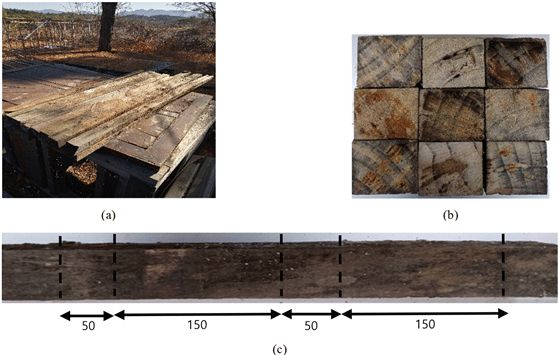
The compression test was carried out according to specified in KS F 2206 with the loading rate of 5 mm/min, and the maximum load was recorded using a universal testing machine. After testing, the moisture contents were measured using an electric resistance type moisture meter. The screw withdrawal test was conducted using a screw withdrawal resistance meter manufactured by Fakopp Enterprise, Hungry, as shown in Fig. 2(b). The sample size and position of the screws followed the standard KS F ISO 9087. The test process was as follows. First, the equipment was placed onto the screw. The screw head was caught, and the handle was turned slowly clockwise until the screw was removed according to the user’s guide. Before testing, lead holes were drilled 2 mm perpendicular to the wood surface, and screws were then screwed into the pre-drilled lead holes. The penetration depth was 20 mm. Two holes were drilled in one section, and the sample was then rotated 90°. Another two holes were drilled in the other section. The average of four test results per sample was recorded. The compressive strength parallel to the grain and screw withdrawal were calculated as follows:
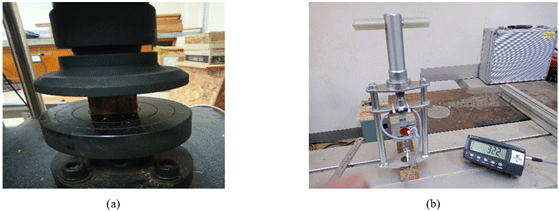
Where;
A = the cross section (mm2),
Pmax = the maximum compression load (N)
L = penetration depth (mm)
Smax = the maximum screw withdrawal load (N)
3. RESULTS and DISCUSSION
Table 1 lists the average moisture content and residual density. The original density of the same stock wood samples was 452 kg/m3. The average residual density of the tested samples was 350 kg/m3, and the ratio of the residual density to the original density was 0.77. Hence, there was approximately 23% density loss. The density reduction and decay were attributed to the weight loss caused by insect damage.
Moisture Content (%) | Residual density (kg/m3) | Original density | Ratio | |
---|---|---|---|---|
Minimum | 9.10 | 257 | ||
Maximum | 13.9 | 428 | ||
Average | 10.93 | 350 | 452 | 0.77 |
COV (%) | 10.06 | 11.58 |
The average compression strength parallel to the grain of the tested samples was 17.92 N/mm2 and ranged from 9.53 N/mm2 to 24.88 N/mm2, as shown in Table 2. The reference value of the same species in the wood handbook was 41.90 N/mm2, and decayed samples were lowered to 43% compared to the reference value. The lowered density due to decay was replaced with the strength reduction. Other studies reported that the residual strength of decayed wood in compression was 50% (Mizumoto, 1966) and 33.1% (Sousa et al., 2014) of the original strength. Stalnaker and Harris (1997) reported that the compressive strength was reduced markedly to 55% in the case of 5 to 10% weight loss. The weight loss of larch lumber was approximately 5% due to decay by termites (Park et al., 2018) and around 6.2 ‒ 10.6% for Parashorea spp. (Suprapti et al., 2020). The test result showed that a lower strength indicates a higher level of wood damage due to insects.
Samples | Compression parallel to the grain (N/mm2) | ||
---|---|---|---|
Min | Max | Mean | |
Decayed | 9.53 | 24.88 | 17.92 |
Reference value | 41.901) | ||
Ratio of decayed to the reference value | 43% |
Fig. 3 shows the correlation between the residual wood density and compression strength parallel to the grain of the tested samples. The coefficients of determination (R2) were 0.715, which provided a good correlation between the residual density and compression strength parallel to the grain in decayed wood samples. Cruz and Machado (2013) reported a 0.743 correlation between the density and compressive strength parallel to the grain of pinewood affected by beetle attack. Therefore, the density of decayed wood can be an indicator of the residual apparent compressive strength, which would be useful for an in-situ assessment of wood members in an old timber structure.
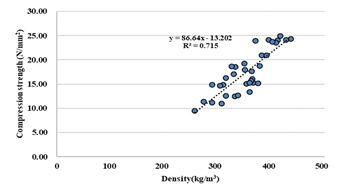
The failure mode in compression parallel to the grain is generally divided into six categories (Bodig and Jane, 1982). Fig. 4(a) shows the percentage of the failure types of the samples obtained from the compression test. Crushing was an effective form of failure and splitting, wedge splitting, brooming in order, and three main failure types of the samples, as shown in Fig. 4(b). In particular, brooming or end rolling was considered a special failure type in wood affected by decay. The end section of the samples was decayed and easily crushed under a load, as shown in Fig 1(b). Bodig and Jane (1982) also pointed out that such failure results from the peculiar end conditions, which were observed in the present case.
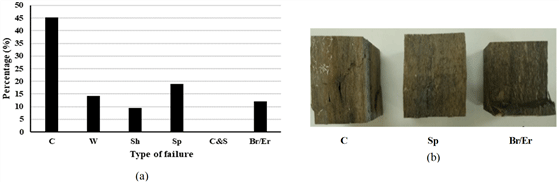
Table 3 lists the average screw withdrawal strength. The overall average of the screw withdrawal strength was 57.40 N/mm and showed a wide range of strength from 15.56 N/mm to 127.82 N/mm. This was attributed to density variations between the samples; a lower density leads to lower screw withdrawal strength. Íñiguez et al. (2010) reported screw withdrawal strengths of radiata pine of 2.19 kN and 110 N/mm with a penetration depth of 20 mm. They used the same equipment as this study with a 4 mm diameter and 70 mm long screw. The average screw withdrawal strength of this study was 57.5 N/mm and approximately 52% to the previous reference value. Therefore, insect-damaged wood was estimated to have a very low screw withdrawal strength.
Fig. 5 shows the relationship between the screw withdrawal strength and wood density. The coefficients of determination (R2) were 0.63, showing a positive correlation between the density and screw withdrawal strength in insect-damaged wood samples. Parracha et al. (2019) reported a strong correlation between the loss of density and the screw withdrawal strength of maritime pine lumber degraded by anobiids with a coefficient of 0.64. They also reported that the correlation between residual density and screw withdrawal strength was 0.84, but this value was obtained using a very limited number of samples. Nunes et al. (2019) obtained a correlation coefficient between the screw withdrawal strength and density of 0.56 for artificially degraded pine timbers. Therefore, the screw withdrawal strength can be a predictor of residual density. Hence, the density of decayed wood can be estimated from the screw withdrawal strength. This can be a useful tool for making in situ estimations of the residual density of decayed wood. Tannert et al. (2013) pointed out that screw withdrawal measurements provide local information on the measuring locations. Hence, the results from the test are valid for selecting the locations for the screw withdrawal measurements. Eight measurements per lumber sample were done by referring to their research.
Fig. 6 plots the relationship between the screw withdrawal strength and compression parallel to the grain using the mean values. The coefficients of determination (R2) were 0.645, and they showed a reasonable correlation, so two variables can be correlated. Table 4 lists the correlation between the three variables. The correlation between the density and compression strength parallel to the grain showed a closer relationship than the density and screw withdrawal strength, but the difference was very small. As the determined correlation coefficient showed a strong correlation between the three variables, these variables can be considered to be closely correlated. The in situ screw withdrawal strength was measured from the analysis, and the residual density and compressive strength to the grain were estimated. Therefore, the in situ screw withdrawal test method can be a useful tool as a semi-destructive test method to evaluate the residual density and compression strength of an existing timber construction.
Density | Compression Parallel to grain | Screw withdrawal | |
---|---|---|---|
Density | 1 | ||
Compression Parallel to grain | 0.843978 | 1 | |
Screw withdrawal | 0.801154 | 0.80291 | 1 |
4. CONCLUSION
Lumber exposed to outdoor conditions and decayed by insect damage was assessed by measuring the compressive strength parallel to the grain and performing the screw withdrawal strength test. The main conclusions of this study are as follows:
-
The average compression strength parallel to the grain of decayed samples was lowered to 43% of the reference value. The test result showed similar trends to previous research, which means that the lower strength indicates more significant damage to wood samples caused by insect damage. The correlation between the residual wood density and compression strength parallel to the grain of tested samples showed a good correlation between residual density and compression strength parallel to the grain. The residual apparent compressive strength was estimated from the density of the wood. In the failure type of compression, crushing was a major form of failure, and brooming or end rolling was considered a special failure type in wood affected by decay.
-
The average screw withdrawal strength was low relative to the reference value of undamaged lumber, and a wide range of strengths was observed. The lower density leads to lower screw withdrawal strengths. Hence, degraded wood was estimated to show much lower screw withdrawal strength. The relationship between the screw withdrawal strength and wood density provides a meaningful correlation between two variables. From the test results, the density of decayed wood can be estimated from the screw withdrawal strength, which can be a useful tool for estimating the residual density of decayed wood. This suggests that screw withdrawal strength can be a predictor of the residual density.
-
The relationship between the screw withdrawal strength and compression parallel to the grain showed a reasonable correlation. Hence, two variables can be correlated. Statistical analysis of the correlation between the three variables, the correlation between density and compression strength parallel to the grain showed the highest value. The correlation between the screw withdrawal strength and compression strength parallel to the grain was approximately the same as that between the density and the screw withdrawal strength.