1. INTRODUCTION
The main performance required for the beams in buildings constructed using the column-beam method is to withstand the expected vertical load during the period of use. In this case, structural-sized lumbers are evaluated by applying an external force within a short period of time in a static strength test. However, the deformation behavior of the flexural member during the application of a long-term external force is also very important in terms of the reliability of the building structure (Byeon et al., 2017). For hydrophilic timbers, the moisture absorption and desorption have a significant effect on strength property and dimensional deformation. Therefore, the creep phenomenon should be considered in structural design by using viscoelastic timbers that are not completely elastic (Epmeier et al., 2007). In South Korea, where the temperature and humidity variations are mostly due to the distinct seasonal changes, it is deemed necessary to conduct further research on mechano-sorptive behavior, which causes deflection of members by the interaction between stress and moisture content. Mechano-sorptive behavior has been the focus of many studies because it can increase deflection and result in early failure of timber beams. Many researchers have attempted to investigate the variables about mechano-sorption and the effects on creep behavior.
Hunt (1991) raised a question about the importance of relative humidity variation and suggested that the change rate of relative humidity could be important in mechano-sorptive creep. Bengtsson (2001) investigated the factors that influence mechano-sorptive creep, such as timber density, modulus of elasticity, fiber slope, and annual ring width, and found that the modulus of elasticity is the best parameter for predicting the relative creep. Bengtsson and Kliger (2003) conducted a creep test for Norway spruce according to the drying temperature. As a result, the timber dried at a high temperature (115 °C) and showed a 30% smaller creep deformation than the timber dried at a low temperature (70 °C). Furthermore, it was less affected by the moisture cycles. Aratake et al. (2011) conducted a bending creep test for the glulams manufactured differently depending on the composition of the Japanese Sugi and Douglas fir timbers, which have a very low modulus of elasticity. They reported that the bending creep showed opposite trends for the outer- layer timbers with large and small moduli of elasticity, regardless of the modulus of elasticity of the middle-layer timber. Ranta-Maunus and Kortesmaa (2000) researched on whether wood-based materials, such as glulams, could resist creep by surface coating. The creep tests were performed for seven to eight years in two environments: an environment where the temperature and humidity change under a low load, and a heated environment. As a result, the creep of wood-based materials progressed continuously and slowly, showing a high dependence on surface coating. The durations and moisture cycles of the creep test vary greatly according to the literature. O’Ceallaigh et al. (2014) reported that the wood specimens must be adjusted in accordance with the equilibrium moisture content in a new environmental condition during the creep test in order to produce the mechano-sorptive effect. For this reason, they suggested that the test must be conducted for a duration that allows sufficient observation of the moisture cycle because a short moisture cycle offers inaccurate result about the mechano- sorptive effect of timber.
Meanwhile, the increase of the beam span is inevitable with the increase in the number of large timber structures. The most basic solution for this is to extend the length of the members by applying connections between the beams. The most representative method of longitudinal joining of glulams is to put a metal plate, such as steel plate or gusset, on the outside of a member or insert it into a member and fix it with a metal fastener (Lee et al., 2017). It has been reported that the behavior of this connection or joint is affected by moisture and has a close relationship with the structural performance of timber buildings (Sjödin et al., 2004; Hong and Park, 2006). The effect of moisture cycling can be significant and should be taken into account in timber design when the timber structural members are exposed to cyclic moisture conditions (Svensson and Toratti, 2002; Chen et al., 2003; Awaludin et al., 2008; Guan and Rodd, 2001; Xu et al., 2009).
The objective of this study is to present research data about bending creep deflection according to the change of the relative humidity of glulam and bolted glulam under a load. In a room under the influence of an ambient relative humidity, glulams and bolted glulams were observed for approximately four years (33,000 h) under 20% and 30% stress levels, respectively.
2. MATERIALS and METHODS
The seven layers of glulam were manufactured by using domestic larch (Larix kaempferi Carr.) laminae, which were dried to the point of having 11% to 12% moisture content and were not end jointed. Phenol-resorcinol- formaldehyde (PRF) was used as an adhesive (Kangnam Chemical, Seoul, South Korea), and the glue spread was set at 300 g/m2 (single spread). The pressing pressure was set at 0.98 MPa. After being hardened for 24 hours, the specimens were cured at room temperature for one week. A total of 24 glulams were fabricated with a thickness of 175 mm, a width of 130 mm, and a length of 3500 mm.
There were 12 glulams manufactured as bolted glulams, and 12 glulams manufactured as non-bolted glulams. Bolted glulam is a specimen fabricated by interconnecting two beams using steel gussets and bolts to expand the beam span. The center of each of the glulams manufactured with seven layers was cut, and a steel-gusset plate was inserted in the center of the beam, as shown in Fig. 1. The connecter was a steel-gusset plate with a thickness of 8 mm, a width of 155 mm, and a length of 460 mm, and its bolts were 12 mm in diameter. At the center of each cut beam, the end distance of the bolt was 85 mm, the distance of the bolt diameter was 7d (d: bolt diameter), and the distance between the bolts was 95 mm. The bolts of the four pieces were used at each side of the cut beams, and a total of eight bolts were used for connecting.
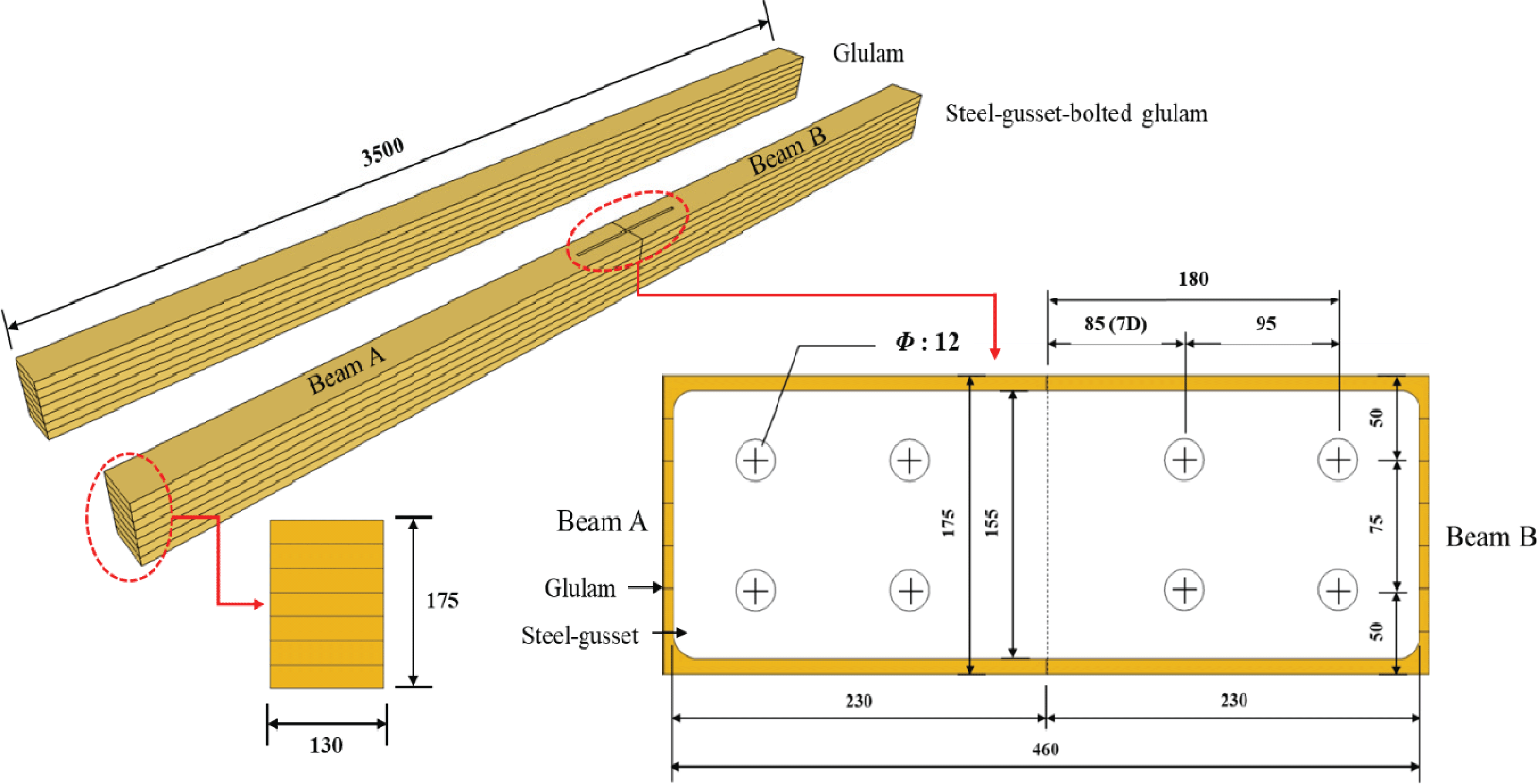
The glulams were subjected to a bending test by loading 4-point bending with a 2700-mm span and a 400-mm length between the load points. The bolted glulams were also subjected to a bending test after dividing the 2700-mm span into three parts, each with a 900-mm load length. The load and deflection were then measured by using a data logger (TDS-303). Fig. 2.
Six glulams and six bolted glulams were used in the bending creep test. The geometrical moment of inertia of the glulam beams was 5.8×10-5 m4. The span and load length in the creep test were the same as those in the bending test. The creep test was performed with the TDS 303 data logger every hour after a displacement transducer was placed at the center of each specimen. The modulus of rupture (MOR), which is the basic stress level, was calculated by using the mean of the 12 manufactured glulams. The creep deflection according to the changes in the temperature and relative humidity were measured after loading 20% and 30% of the load from the result of the bending strength (Table 1). The creep test was performed under the same conditions as when the 12 specimens were placed in a workshop whose humidity was significantly changed by opening and shutting the window. The relative humidity was measured every hour. The mean of the relative humidity was the mean of the values obtained from after the measurement to before the next measurement. Fig. 3 shows the method that was used for the creep testing under changing humidity. The creep test was performed for approximately 33,000 hours.
3. RESULTS and DISCUSSION
As a result of the flexural strength tests for six glulams, the average MOR was measured at 50.0 MPa. Consequently, a stress of 10.0 MPa (stress level of 20%) was applied to the CON20 specimens and a stress of 15.0 MPa (stress level of 30%) was applied to the CON30 specimens.
The creep deflection of the glulams up to 33,000 hours is shown in Fig. 3. The creep deflection of the glulams up to 5,000 hours was deformed more by the load than by the temperature and relative humidity. After 5,000 hours, the creep deflection of the glulam was stabilized by loading, but the deflection progressed and was recovered by temperature and relative humidity. Fig. 4 shows a comparison of the relative humidity and relative creep of glulam CON20-2. The relative creep was affected by the load from the first spring to summer, and then stabilized and progressed slowly. The relative creep increased during the second autumn and winter, and recovered during spring and summer. The relative creep also increased during the third autumn and winter, and recovered during spring and summer. Deflection and recovery were repeated periodically as the temperature and humidity changed, and creep deflection progressed slowly.
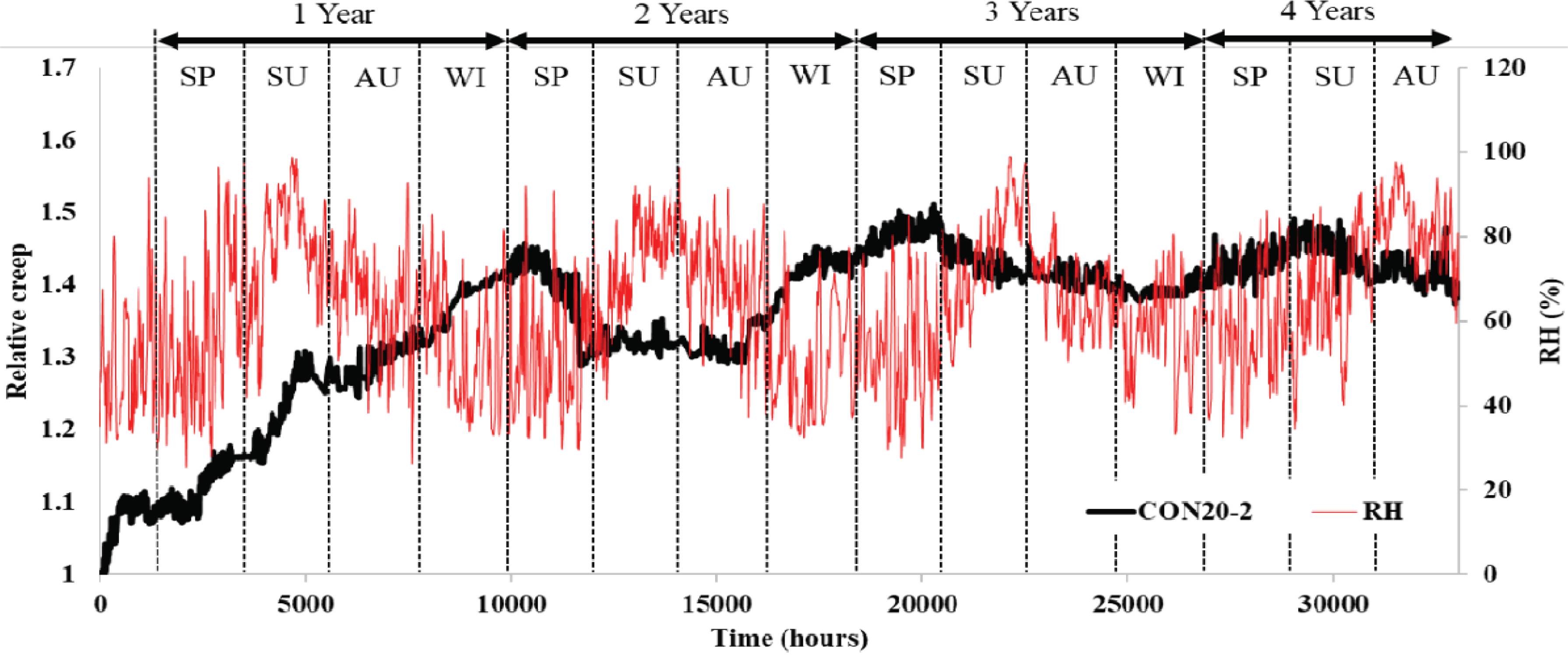
Table 2 shows the relative creep that occurred every spring (1st, April) and autumn (1st, October). The relative creep increased to 1.17, 1.34, and 1.41 as the time increased to 11,000 hours during the second spring, but recovered to 1.37 during autumn of the same year. The relative creep increased to 1.43 during the third spring and recovered to 1.41 during autumn. In the creep deflections of the glulams, there was repeated deflection and recovery. After 33,000 hours, the relative creep of CON20-1, CON20-2, and CON20-3 were 1.59, 1.39, and 1.99, respectively, and the relative creep of CON30-1, CON30-2, and CON30-3 were 1.67, 1.41, and 1.27, respectively. These results were similar to the relative creep (1.6) of the glulam made from spruce as obtained in the preceding study (Ranta-Maunus and Kortesmaa, 2000).
As a result of the flexural strength tests for six bolted glulams, the average MOR was measured at 13.3 MPa. According to this result, a stress of 2.7 MPa (stress level of 20%) was applied to the Bolt20 specimens and a stress of 4.0 MPa (stress level of 30%) was applied to the CON30 specimens.
Fig. 5 shows the creep deflection of the bolted glulams up to 33,000 hours. For the creep deflection of the glulams, it changed abruptly after loading up to 5,000 hours, but stabilized after 5,000 hours, and then progressed until 33,000 hours. Fig. 6 shows a comparison of the relative humidity and the relative creep of bolted glulam Bolt30-2. For the creep deflection of the glulams, the relative creep progressed through loading during the first spring and summer, and then stabilized and increased during the second autumn and winter. In contrast to the creep deflection of the glulams, the relative creep did not recover during spring and summer, but instead stabilized and then increased again during the following autumn and winter.
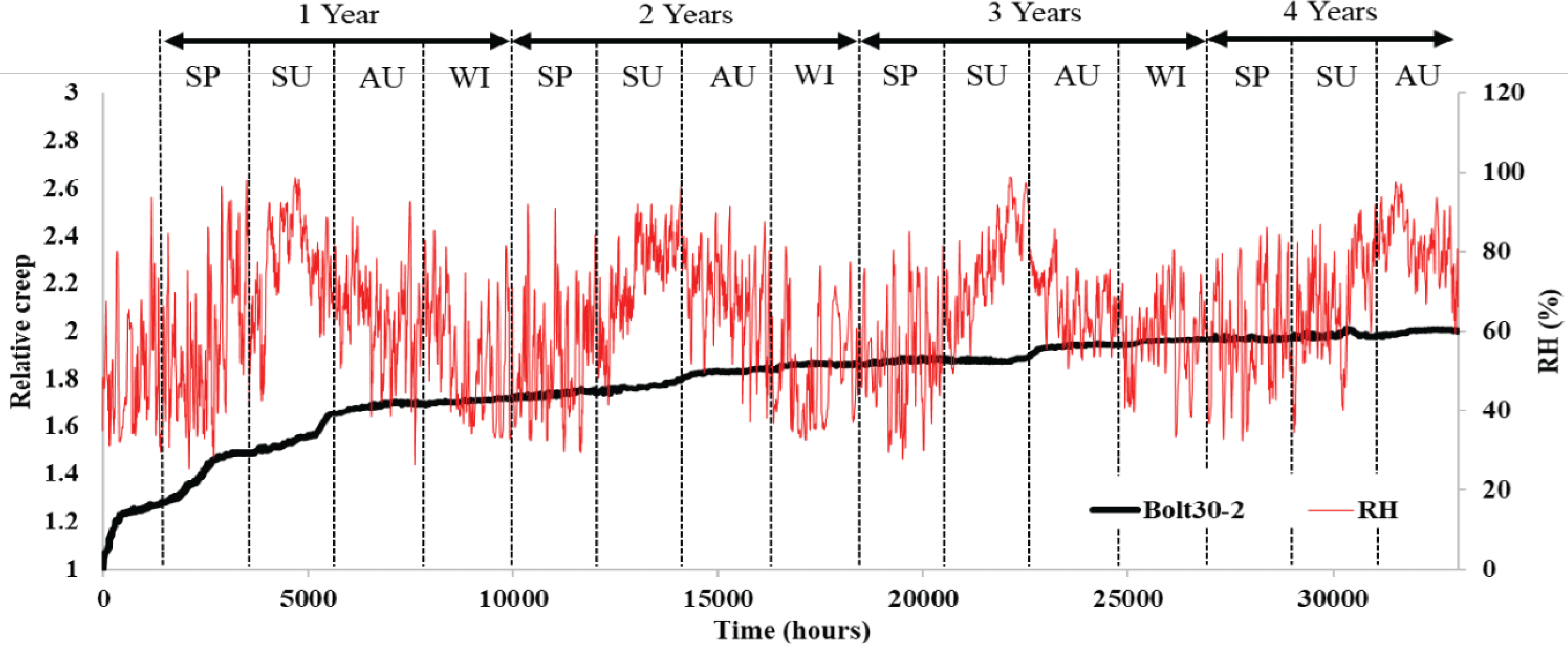
In the glulam connection, a high level of moisture movement occurs by the slot processing for steel plate insertion and the end grain of the members. It has also been reported that a significant change in the moisture content inside a member can result from direct exposure to outside air (Sjödin and Johansson, 2007). The existing research suggests that a change in relative humidity would alter the relative creep of the bolted glulam. However, the relative creep of the bolted glulams in this study showed a sharp increase in a short period of time as the relative humidity decreased between summer and autumn, and then stabilized until the next cycle. This trend reappeared every year. This is due to the fact that the steel plates and bolts in the connection area caused stress constraints by suppressing deformation induced by moisture in the wood, while the existence of the connection decreased the flexural stiffness of the glulam beam at the same time. In other words, the relative creep of the unconnected glulam in this study was more affected by a change of relative humidity than by a load, whereas the relative creep of the bolted glulam was more affected by a load than by relative humidity. The above two different causes that suppress the change in the relative creep of the bolted glulam or their combination are considered to cause a difference in the relative creep behavior between the glulam and the bolted glulam.
Table 3 shows the relative creep of the bolted glulams. The relative creep of Bolt20-2 from 6,500 hours (autumn) to 11,000 hours (spring) increased from 2.02 to 2.06, but increased abruptly to 2.19 at 15,000 hours (autumn). The relative creep did not increase much (only to 2.21) during the following spring, but increased abruptly to 2.30 during autumn. The deflection of the bolted glulams increased slowly from autumn to spring, but changed significantly after summer. The relative creep increased 2.11 times on average after 33,000 hours. The creep deflection of the bolted glulams did not recover differently from that of the glulams, and the deflection progressed continually.
The variation in the creep deflection of glulam with relative humidity was greater than that of the bolted glulam. Between the relative humidity and the creep deflection of glulams, the tendency was close to the inverse behavior. In the case of the bolted glulam, an inverse behavior was observed with the relative humidity as creep deflection increased, but in contrast with the bolted glulam, the creep deflection did not recover, but instead remained stable and tended to increase again. It was confirmed that the larger the bending rigidity of the glulams, the more positive the effect it has on the relative creep and stabilization of creep. However, in the case of the bolted glulam, these tendencies were not significant.
The applied stress level affected the creep deflection of glulam. At 33,000 hours, the average relative creep values of glulam were 1.4 (stress level of 20%) and 1.45 (stress level of 30%). In particular, the average relative creep values of bolted glulam were 1.97 and 2.25, respectively, which exhibited more effect from the stress level than the glulam. Bolted glulam showed a repetitive increase and stability of the relative creep, unlike glulam, which had a repetitive increase and recovery of the relative creep. In addition, the bending rigidity of the bolted glulam did not significantly affect the resistance of creep deflection, which increased as the stress level increased. Eventually, after 33,000 hours, the relative creep of the bolted glulam was 49% higher than that of the glulam. These results indicate that the beam-to-beam connection of glulam is a factor in the deterioration of the bending creep performance and the need for further studies on the reinforcement of the connection.
4. CONCLUSION
In this study, the behavior of the creep deflection of glulam beams and steel-gusset-bolted glulam beams was examined under relative humidity variation.
The relative creep of the glulams was affected by loading until 5,000 hours. After 5,000 hours, their relative creep was stabilized by loading, but the relative creep progressed, and then recovered by the temperature and relative humidity.
After 33,000 hours, the relative creep of the CON20 series increased by 39% to 99%, and the relative creep of the CON30 series increased by 27% to 67%, as compared with instantaneous elastic deflection. The relative creep increased during autumn and winter, and the relative creep recovered during spring and summer.
The relative creep of the bolted glulams changed abruptly by loading up to 5,000 hours, but stabilized after 5,000 hours, and then gradually increased until 33,000 hours.
In the case of the bolted glulam, a rapid increase in relative creep occurred after summer, but the increase was moderate and steady. As a result, the relative creep of the bolted glulam increased 2.11 times after 33,000 hours, which was 49% higher than that of the glulam.