1. INTRODUCTION
Lignin and crystalline structure of cellulose are known as major inhibitor in conversion of biomass into sugar and bioethanol production. Lignin acts as the glue that binds cellulose and hemicellulose via covalent and noncovalent bond, giving rigidity and resistance to lignocellulose (Wang et al., 2020). As a second major component of lignocellulose, lignin tends to have a significant negative influence on enzymatic hydrolysis resulting in low sugar yield. The high lignin content adversely affects the interaction between cellulase and cellulose. Besides that, the presence of lignin can also affect the path for cellulase action thus the hydrolysis becomes slow down (Qing et al., 2010). It was commonly found that lignin appeared to adsorb enzyme more easily than cellulose, leading to the reduce the effectiveness of enzyme activity (Rahikainen et al., 2013). To provide cellulose more accessible by cellulase and increase the possibility of interaction between cellulase and cellulose, the lignin in biomass should be removed by pretreatment. In the acid hydrolysis, lignin was reported inhibit cellulose and hemicellulose hydrolysis into glucose and xylose. Also, the hydrolysis of xylan to xylose and dehydration xylose to furfural from corncob was also interfered by the presence of lignin (Daorattanachai et al., 2013). Lower sugar yield was reported because of the presence of lignin that imply to require high enzyme dosage (Berlin et al., 2006).
In the field, Sugarcane thrash (ST) consists of leaves and tops of sugarcane plant usually left in the field as waste of sugarcane harvesting (Hermiati et al., 2020). ST is also just burnt at harvest (Hermiati et al., 2020) that can generate environmental problem in sugarcane plantation. It counts approximately 15-30% of the total above ground biomass at harvest. In some reports, ST leaves contain 35% cellulose, 23-30.8% hemicellulose, and 10-22.9% lignin (Moodley and Kana 2015, Jutakanoke et al., 2017, Gomez et al., 2014, Arnieyanto, 2018). Similar with ST, sweet sorghum bagasse (SSB) presents as by product of juice extraction of sweet sorghum stalk. This biomass is abundant but the further utilization into valuable materials is still limited (Fatriasari et al., 2015). Regarding its abundance and its chemical component (43.66% cellulose, 35.63% hemicellulose, 24.59% acid insoluble lignin and 0.66% acid soluble lignin) (Solihat et al., 2017), SSB has been utilized as pulp and paper (Fatriasari et al., 2015; Patil et al., 2011; Kumar and Marimuthu, 2012), particle board (Iswanto et al., 2017), sugar and bioethanol (Solihat et al., 2017; Choudhary et al., 2012; Goshadrou et al., 2011; Wang et al., 2012). Together with Malasyia, Indonesia includes in two biggest palm oil producers in the world (Wright and Wiyono, 2013). Oil palm empty fruit bunch (OPEFB) produces as by product of processing oil palm about 5.29 million tons of dry OPEFB in 2016 (Anita et al., 2020). It has 43.0% α.-cellulose, 12.7% hemicellulose, 17.8% AIL, and 1.30% acid soluble lignin (Fatriasari et al., 2018).
Pre-treatment uses various techniques, including chemical treatment with acid solution (Hendriks et al., 2009; Singh et al., 2013; Fatriasari et al., 2017), alkaline solution (Xu et al., 2010; Sills and Gossett 2011, Fajriutami et al., 2016), ionic liquid (Elgharbawy et al., 2016; Han et al., 2020), are aimed to alter the structure of cellulosic biomass to make cellulose more accessible. Acid pre-treatment removes hemicellulose and facilitates the cellulase to break down cellulose into sugar monomer. Compared to inorganic acid such as sulfuric acid, utilization of organic acid such as maleic acid and oxalic acid can minimize generation of secondary degradation products including sugar degradation products and also phenolic compound which are toxic to microorganisms (Oktaviani et al., 2019; Anita et al., 2020). It presents a higher catalytic efficiency for hydrolysis under the same severity conditions (Anita et al., 2020). Meanwhile, alkali pretreatment aims to remove lignin from biomass matrix thus the cellulose is more easily to be accessed. Kraft pulping includes in alkali pre-treatment that reported could effectively degrade lignin in biomass with minimum carbohydrate degradation (Fatriasari et al., 2015). Its ability to handle various raw materials and its high chemical recovery efficiency (~97%) cause this process is favored over other pulping methods (Fortunati et al., 2016).
Besides that, in the bioconversion of biomass, the hydrolytic performance of cellulase enzymes can be enhanced by adding promoters such as surfactant (Jonsson et al., 2013). By combining the treatment i.e. removal of the barrier factor for hydrolysis and addition of surfactant, the performance of hydrolysis will improve. Surfactants, amphiphilic compounds, can reduce surface and interfacial tensions by accumulating at the interface of immiscible fluids, increasing the solubility, motility, bioavailability and subsequent biodegradation of hydrophobic or insoluble organic compounds (Abdeli et al., 2019). It could change the nature of the substrate, e.g., by increasing the available cellulose surface or by removing inhibitory lignin (Helle et al., 1993). In many studies, addition of some commercial surfactant such as PEG 6000, Tween 80 and glyceryl alcohol has increased hydrolytic performance (Zong et al., 2015). The adsorbed surfactant to lignin surface could prevent non-specific binding between enzyme and lignin, leading to enzyme deactivation. As a result, the hydrolysis rate would be enhanced (Kim et al., 1982; Kaar and Holtzapple, 1998; Yoon and Robyt, 2005; Eriksson et al., 2002). In addition, surfactant causes alteration of substrate structure and it can increase the ability to be accessed by enzymes (Kaar and Holtzapple, 1998). Surfactant also causes enzyme stabilization and prevent enzyme denaturation during hydrolysis (Kaar and Holtzapple, 1998; Kim et al., 1982). Other studies reported positive interaction between substrate and enzymes (Eriksson et al., 2002; Kaar and Holtzapple 1998; Malmsten and Van Alstine, 1996) after a surfactant addition on hydrolysis process.
Lignin, a by-product of pre-treatment process, has great potential to be converted into value-added products that could significantly enhance the economics of various industrial applications. Some researchers reported the use of lignin as an additive in pellet (Min and Um, 2017), as activated carbon (Mussattoa et al., 2010), as adhesives (Alinejad et al., 2019), and as water- soluble biosurfactant i.e. Amphiphilic Lignin Derivatives (A-LD). A-LD prevents non-productive absorption of enzyme in hydrolysis process (Winarni et al., 2013; Winarni et al., 2014; Uraki et al., 2001). The addition of A-LD in enzymatic hydrolysis process improved the digestibility of biomass (Cheng et al., 2017; Fatriasari et al., 2018) and the enzyme can be reused (Winarni et al., 2013). A-LD increases the stability of the enzymes during hydrolysis therefore improves the bioethanol production (Cheng et al., 2017).
As reported before, lignin isolated from Acacia mangium black liquor has been converted into biosurfactant. In our previous study, we evaluate the effect of lignin isolation method in A-LD preparation on enzymatic hydrolysis performance of kraft pulp of sweet sorghum bagasse (SSB) (Fatriasari et al., 2018). Based on previous study, the addition 5% of A-LD on enzymatic hydrolysis resulted in higher reducing sugar yield. After that, optimization of the synthesis condition in A-LD reaction has been conducted (Fatriasari et al., 2020) of the synthesis condition in A-LD reaction has been conducted. The optimized A-LD was used in this study. The effect of A-LD derived from A mangium on enzymatic hydrolysis has not observed for other biomass with varies of lignin content. To ensure the improvement effect of A-LD addition, some pretreated biomass were used as substrate of the enzymatic hydrolysis.
2. MATERIALS and METHODS
Sweet sorghum bagasse (SSB) of Numbu variety was collected from BIOTROP plantation (Bogor, West Java, Indonesia) as by product of sugar juice extraction process of sorghum straw. Oil palm empty fruit bunch (OPEFB) was obtained from oil palm plantation in Cikasungka, West Java, Indonesia and sugarcane trash (ST) was from PT PG Rajawali II, Subang, West Java, Indonesia. ST consisted of sugarcane leaves and upper part of sugarcane plant which were left on the field after harvesting. A mangium kraft black liquor with solid content of 70% from PT Tanjung Enim Lestari Pulp and Paper (TELPP), South Sumatera, Indonesia was used for A-LD preparation. Polyethylene glycol diglycidylethers (PEDGE) Mn 500 in analytical grade was used in synthesis of A-LD. The commercial cellulase enzyme (Sigma-Aldrich) from Trichoderma reesei ATCC 26921 containing 42.3 FPU/mL was used for hydrolysis process. The other reagents for sugar analysis were of analytical grade.
SSB chips (250 g Oven Dried Weight/ODW) was delignified by kraft pulping using rotary digester. The pre-treatment was conducted with 17% active alkali and 20% sulfidity, liquid to solid ratio of 10, temperature of 170 °C for 4 h following the Fatriasari et al. (2015). The moisture content, weight loss, and yield of pre-treated SSB were 79.15%, 43.39%, and 56.61%, respectively, while the reducing sugar yield without A-LD addition was 45.57% (Solihat et al., 2017).
OPEFB was pre-treated with oxalic acid at the optimum condition according to Anita et al. (2020). Three grams ODW of OPEFB (40-60 mesh) and 30 mL of oxalic acid solution (1.13% v/v) were inserted in a Teflon tube. Afterward, the sample was heated by microwave digester (START D Microwave Digestion System, Milestone, Italy) at 190 °C for 3.01 min, not including approximately 12 min pre-heating time to targeted temperature. After completed heating, the pre-treated samples were filtered to separate solid and liquid fraction. The moisture content, weight loss, and pulp recovery of OPEFB pre-treated by oxalic acid were 68.27%, 41.07% and 58.93%, respectively. The reducing s of OPEFB pre-treated oxalic acid without A-LD addition was 34.20% (Anita et al., 2020).
OPEFB was also pre-treated with microwave assisted MA using the optimum condition as reported in Fatriasari et al. (2016). As much as 3 g ODW OPEFB (40-60 mesh) was inserted into Teflon tube and then 30 mL of MA solution 1.2% (w/v) was added. The pre-treatment was conducted at 185 °C for 2.8 min. The liquid fraction was separated from solid fraction by filtration. The solid fraction was used in enzymatic hydrolysis with A-LD addition. The weight loss and pulp recovery of OPEFB after pre-treatment were 36.3% and 63.34%, respectively with 67.32% of moisture content. The reducing sugar yield of OPEFB pre-treated by MA in this optimum condition was 52.42% (Fatriasari et al., 2016)
The pre-treatment of ST was conducted by following the method of Arnieyanto (2018). Three grams ODW of sugarcane trash (ST) (40-60 mesh) was inserted into Teflon tube and then added with 30 mL of maleic acid solution (pH 2). The microwave pretreatment was conducted at 180 °C for 5 min, not including approximately 12 min pre-heating time to targeted temperature and 10 min cooling time. After irradiation the tubes were cooled in an ice bath for 30 min. The solid fraction was obtained by filtering the through filter paper by vacuum filtration. The solid fraction was to be subjected in enzymatic hydrolysis with A-LD addition.
Lignin isolation from A. mangium black liquor was conducted according to method of Fatriasari et al. (2018). There are two kinds of crude A-LD including A-LD L1S and A-LD L2S which were synthesized from kraft lignin 1 step (L1S) and 2 step (L2S) isolation method. The A-LD was prepared following the optimum condition of synthesis that reported by Fatriasari et al. (2020). One gram of L1S and 3 g of PEDGE were used for A-LD L1S synthesis, while the amount of L2S and PEDGE for A-LD L2S synthesis were 0.6 g and 2 g, respectively. Both variations were reacted at 60 °C for 1 h. A-LD LS (commercial lignin) was synthesized by reacting 0.6 g of LS and 3 g of PEDGE at 60 °C for 2 h. Three variations of A-LD were added in enzymatic hydrolysis of pretreated biomass.
A 200 mg ODW of pretreated biomass was inserted into 100 mL of conical flask and then added with 200 μL 2% w/v sodium azide, 10 mL sodium citrate buffer (0.05 M, pH 4.8), 30 FPU/g substrate of cellulase enzyme, and 5% A-LD/ODW substrate with total volume of 20 g obtained. Subsequently, the mixed samples were incubated using incubator shaker (WiseCube WIS-30R) at a rate of 150 rpm, and 50 °C for 72 h. The control of substrate, enzyme, and buffer was also prepared besides the samples. The replication of enzymatic hydrolysis was conducted in triplicate. After process completed, the mixtures were centrifuged at 10.000 rpm for 5 min to separate the supernatant from the residue, which was specifically placed in a freezer at temperatures of 5-10 °C. The supernatant was determined for its reducing sugar concentration using DNS method Miller (1959) by UV-Vis Hitachi U-2001 at wavenumber of 540 nm. By considering the weight loss during pre-treatment and reducing sugar concentration of the hydrolysate, the reducing sugar yield per biomass (g/100 g) were calculated based on the equation of Anita et al. (2020) with adjustment in total weight of enzymatic hydrolysis solution is 20 g that equal to 20 mL.
The chemical content of pre-treated biomass (ST and OPEFB) was analysed, including EtOH-benzene content (TAPPI T204 cm-97 1997), ash content (TAPPI T211 om-02, 2002), acid soluble lignin and Acid insoluble lignin (AIL) (Sluiter et al., 2012), holocellulose content (Wise et al., 1946), α.-cellulose content (Rowell et al., 2005). The hemicellulose content was obtained by subtracting the holocellulose content with α.-cellulose content (Punyamurthy et al., 2013).
To estimate organic acid pre-treatment severity and comparing with pre-treatment method, the severity factor (SF) and combined severity factor (CSF) that estimate the effect of an acidic catalyst (Jang et al., 2016) were determined using Eq. 1 and 2, respectively (Goh et al., 2012, Marklein et al., 2016). The SF does not take into account any acids used as a catalyst in the process (Marklein et al., 2016).
where t is the irradiation time (min), and T is the reaction temperature (°C).
The CSF (Eq. 2) was determined based on the equation of Lee et al. (2010) and Loow et al. (2016) with considering the pH of the solution
Crystallinity index of OPEFB pre-treated maleic acid and oxalic acid, ST pre-treated maleic acid was determined using Shimadzu XRD-700 MaximaX while SSB kraft pulp and was analysed using Rigaku SmartLab. The operation condition was 2θof 10-40° with Cu-Kα.radiation operated at 30 mA and 40 kV, and the crystallinity index (CI) was calculated based on Eq. 3 (Focher et al., 2001).
Fc and Fa denote the area of the crystalline region and non-crystalline region, respectively.
3. RESULTS AND DISCUSSION
In attempt to propose effective utilization as bioenergy of waste biomass i.e. ST, OPEFB and SSB, this study was conducted by evaluating the performance of pre-treated substrate for enzymatic hydrolysis with variation of their lignin content by addition of lignin based biosurfactant. Table 1 presents the comparison of chemical component of OPEFB, ST and SSB kraft pulp. Kraft pulping indicates as the most effective pre-treatment in removing lignin content in biomass. Kraft delignification facilitates in improving enzymatic hydrolysis performance (Monrroy et al., 2012). Solihat et al. (2017) reported that this pre-treatment has successfully removed 90% AIL and 50% hemicellulose, respectively. This effective delignification is affected by the chemical used in kraft delignification i.e. sodium hydroxide (NaOH) and sodium sulfide (Na2S). Alkali facilitates in removing lignin while hydrosulfide (HS-) and sulfide ions contained in Na2S can preserve the cellulose by stabilization at the end group of cellulose chain. The ions have capability in accelerating delignification process by attacking β aryl ether bonds in lignin polymer resulting high pulp yield (Fengel and Wegener, 1989; Sjostrom, 1981). In other hand, organic acids such as maleic acid and oxalic acid can be combined with microwave pre-treatment to optimize the pre-treatment process. They belong to dicarboxylic acid that can disrupt the lignocellulosic structure and hydrolyze hemicellulose into xylose selectively. Oxalic acid or Maleic acid is suitable for pre-treatment in releasing fermentable hemicellulose sugars without degradation product formation extensively. Both acids have greater effect in removing hemicellulose than that of lignin. Comparing with inorganic acid such as sulfuric acid (SA), the hydrolysis of β-1-4 glycosidic bonds by maleic acid is more selective (Fatriasari et al., 2018). Thus, it can be understood that the lignin content in biomass after kraft delignification was lower than those after the other pre-treatment methods. Comparing with mangkuang (Pandanus artocarpus Griff) that has been pretreated using biological-liquid hot water or liquid hot water-biological pre-treatment (Yanti et al., 2019), the kraft pulp of SSB and pulp of maleic acid/oxalic acid pre-treated OPEFB have higher cellulose and lower lignin content than does the mangkuang pulp. However, cellulose of kraft pulp of SSB is lower than that of soda pulp of jabon wood (79.85%) with AIL of 4.56%. Table 2 shows the SF of acid pre-treatment of biomass. The three acid pre-treatments tend to have similar SF and CSF with the highest SF and CSF are found in pre-treatment of OPEFB by maleic acid. Even though the CSF of oxalic acid pre-treatment OPEFB and maleic acid pre-treatment of ST are similar, the effect on chemical composition in the pre-treated maleic acid is different significantly, indicated by the much higher cellulose and lower hemicellulose content in the oxalic acid pre-treated OPEFB than those in the maleic acid pre-treated ST.
Pre-treatment method | Severity Factor (SF) | Combined Severity Factor (CSF) |
---|---|---|
OPEFB pre-treated maleic acid | 3.13 | 1.39 |
OPEFB pre-treated oxalic acid | 2.95 | 1.03 |
ST pre-treated maleic acid | 3.05 | 1.05 |
As known well that the presence lignin during hydrolysis can interfere the enzyme work to break down cellulose into its sugar monomer. Lignin was reported could absorp enzyme more easily than cellulose, thus, the enzyme cannot work effectively (Lee et al., 2010). During pre-treatment, a part of lignin can be solubilized. Besides that, lignin can undergo condensation and be deposited on the cellulose surface during pretreatment. It might block access enzyme into cellulose (Lai et al., 2014; Pareek et al., 2013). Lignin has significant negative effect on lowering sugar yield. Therefore, high enzyme dosage is required to achieve high sugar yield (Berlin et al., 2006). A surfactant addition in enzymatic hydrolysis is also the other method in improving high sugar achieved as reported in several previous studies after pre-treatment stage. Wang et al. (2020) reported alkali treated materials containing higher relative xylan contents with lower relative lignin contents has higher enzymatic efficiencies than acid treated materials. It means that xylan removal is less important than lignin removal. Based on CSF, the effectiveness of lignin removal by pre-treatment has different trend. Oxalic acid pre-treatment with the lowest CSF is more beneficial to OPEFB than does maleic acid pre-treatment in the removal of lignin and hemicellulose.
The ethanol-benzene extractives content of oxalic acid and maleic acid OPEFB are higher than that of ST, while the ash content is vice versa. As non-structural component, extractives have also dissolved to facilitate enzyme accessibility into substrate (Fatriasari et al., 2018). Terpenoid compound, phenolic, waxes, fats, resins, oils, and tannins as well as certain components that are not soluble in ether can dissolve in alcohol- benzene solution (Lukmandaru, 2016; Sjostrom, 1998). Extractives of OPEFB that can dissolve in ethanol- benzene solution are greater than those of ST. This might be due to the oils and fats remained in the OPEFB after the palm oil was extracted from the fresh fruit bunch. A higher ash content can influence the enzymatic hydrolysis. The cations in ash contained in straw could migrate into the solution and affect the cellulase activities. In addition, the ash cation removal during pre-treatment can increase enzymatic hydrolysis efficiency especially at low concentration of cellulase (Bin et al., 2010). Therefore, high ash content in pre-treated ST might give more negative effect on enzymatic hydrolysis compared to pre-treated OPEFB.
Holocellulose is main component of biomass as source of sugar that consists of cellulose and hemicellulose. Maleic acid and oxalic acid assisted microwave pretreatment and kraft pulping are performed to facilitate enzyme to access carbohydrate more effectively. Based on Table 1, kraft pulping results in the highest α.-cellulose with comparable hemicellulose content with OPEFB pre-treated oxalic acid and maleic acid. It means that this pre-treatment facilitates delignification process and prevent extensive degradation of carbohydrate more effectively. Higher holocellulose content, means higher potency of sugar that can be converted into its sugar monomer. Even though the α. cellulose content of SSB kraft pulp is comparable with acid pre-treated OPEFB, the hemicellulose contained in the SSB kraft pulp is very high. The high hemicellulose percentage in the SSB kraft pulp was caused by the high loss of lignin during the pulping process. Compared to α.-cellulose that has high molecular weight with straight bond structure, hemicellulose has lower molecular weight with branch structure polymer. This structure make hemicellulose can be hydrolysed more easily.
The proposed reaction between kraft lignin and PEDGE to result A-LD is shown in Fig. 1. Fig. 2, 4 and 5 show the effect of biosurfactant addition on enzymatic hydrolysis of pre-treated biomass with variation of lignin content on sugar concentration, reducing sugar yield per pulp and reducing sugar yield per biomass, respectively. As can be seen in Fig. 2, A-LD (L1S, L2S and LS) did not always give positive effect on sugar concentration.
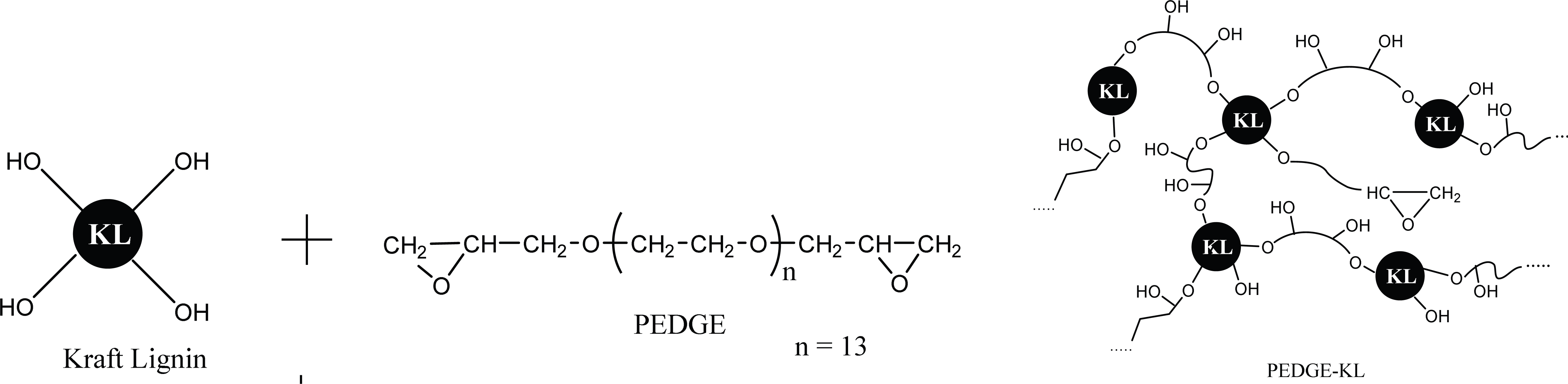
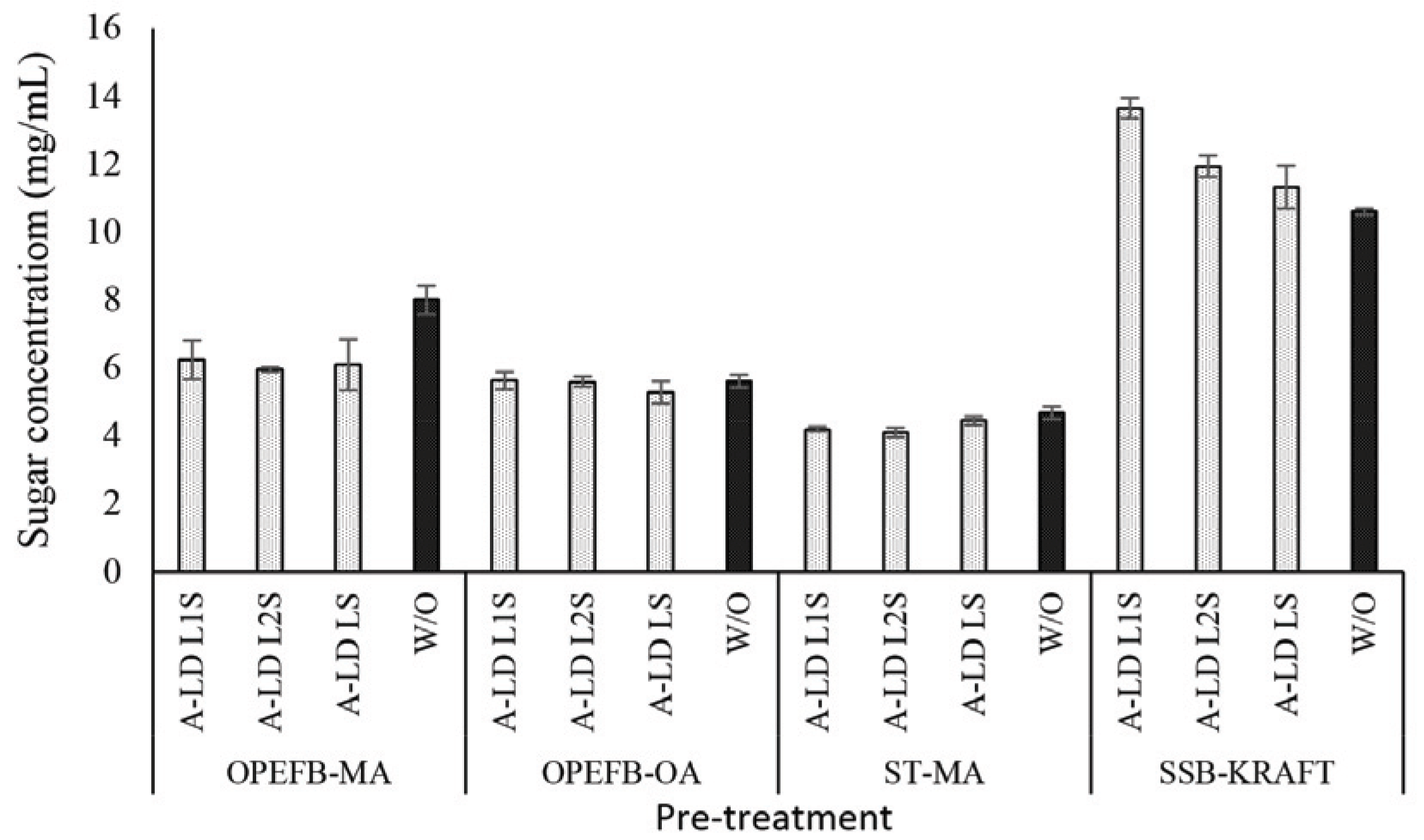
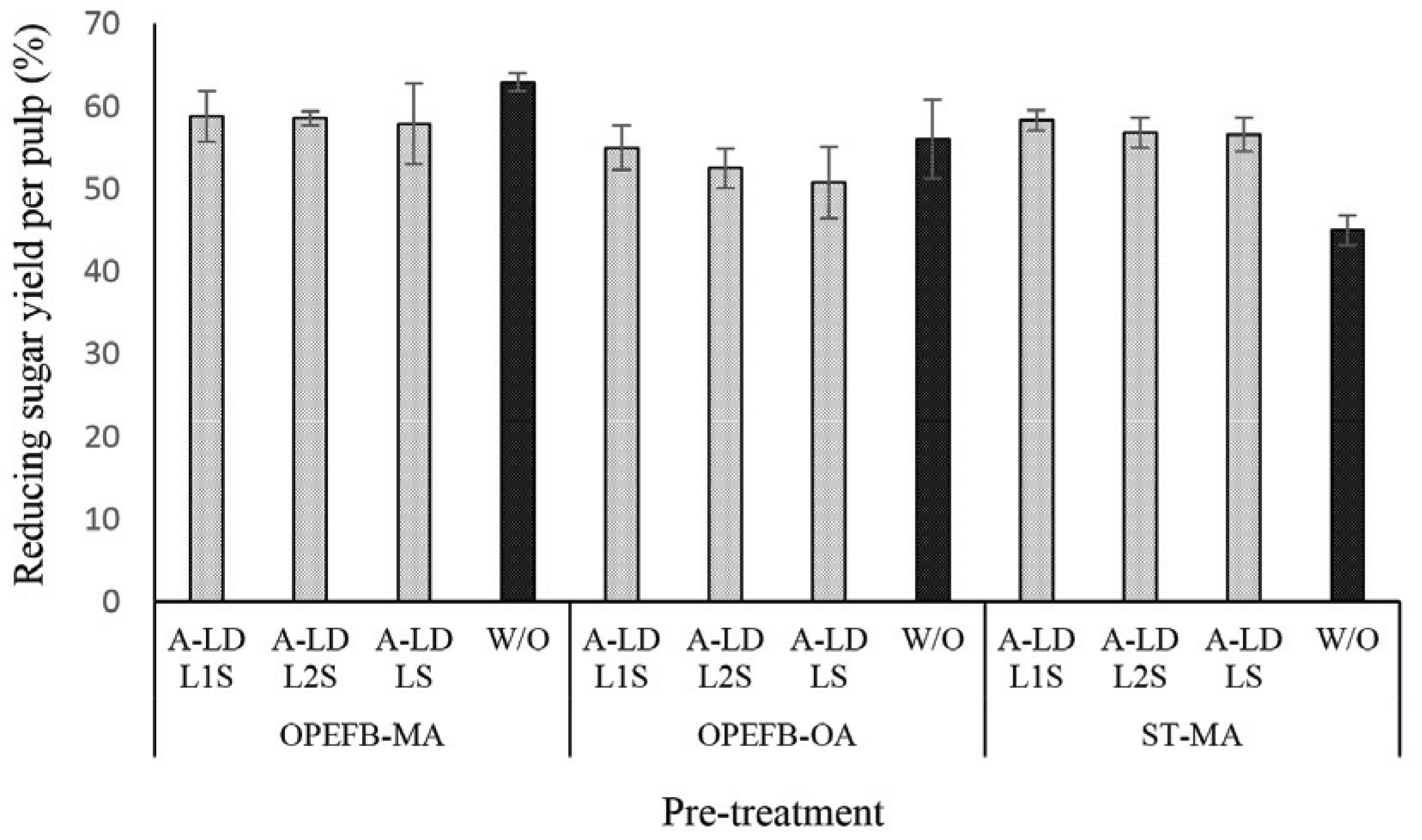
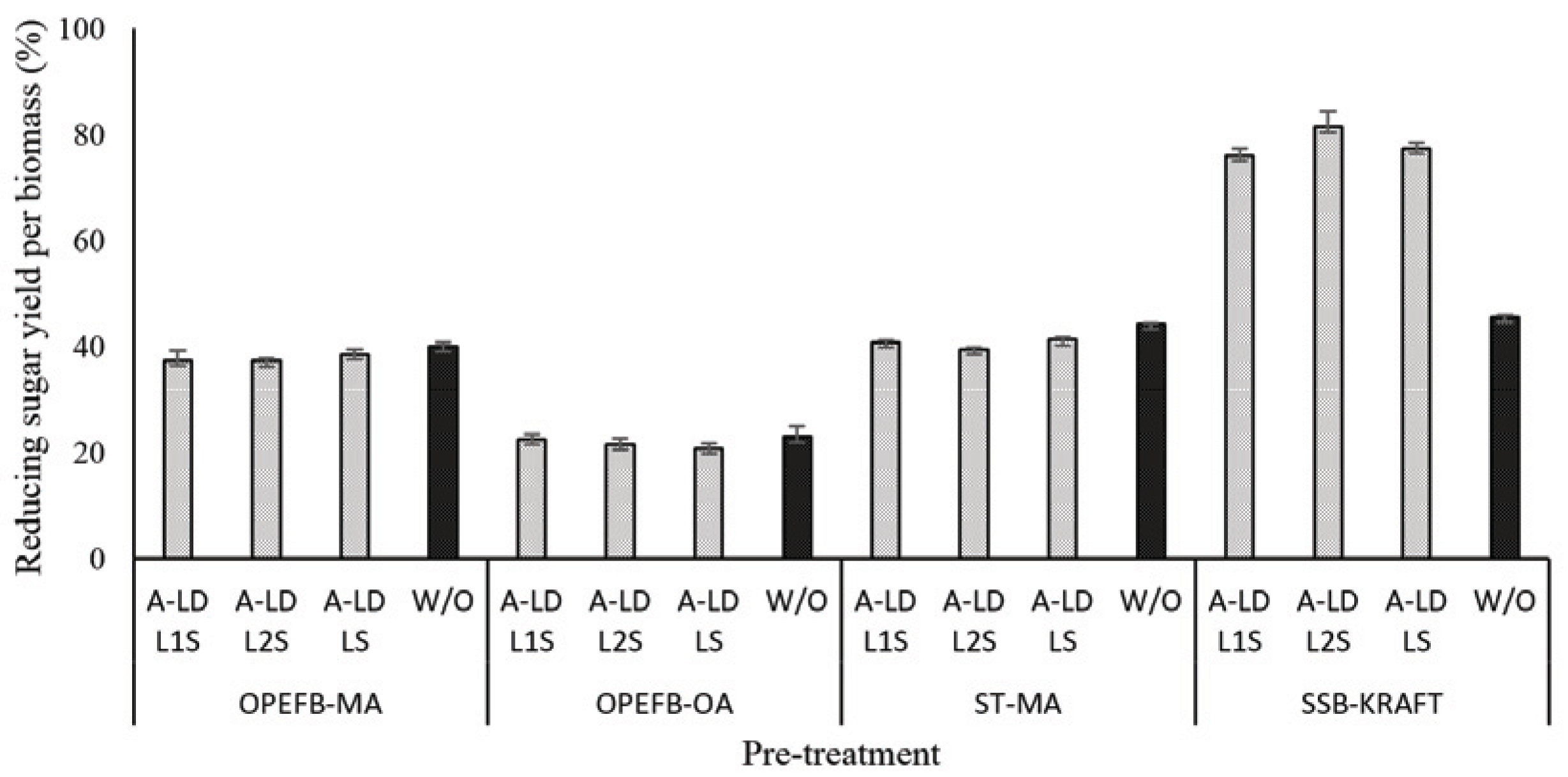
In OPEFB and ST, the addition of A-LD cannot improve the sugar concentration of pre-treated OPEFB and ST. It is affected by the relative high lignin content in pre-treated OPEFB and ST that can inhibit the enzyme to access the cellulose. In the enzymatic hydrolysis system, lignin adsorbs the cellulase via hydrophobic interactions, electrostatic and hydrogen bonding interaction (Pareek et al., 2013; Nakagame et al., 2011). However, previous study reported that lignin isolation methods affect the lignin properties (Li et al., 2016). Consequently, the biosurfactant synthesized was also affected, so that this A-LD did not give different performance in sugar concentration. Based on FTIR analysis of A-LD in Fatriasari et al. (2020), A-LD L2S and A-LD LS have similar functional groups while L1S has different functional groups with the A-LD L2S and LS, as well as shows the lowest surface tension. Lignin presence in pre-treated biomass decreased the maximum adsorption capacity of cellulases on cellulose, which was related with the reduction of available surface area of cellulose for cellulases due to adsorption of lignin on cellulose (Li et al., 2016). In addition, Li et al. (2018) reported that lignin is more easily absorbed the enzyme compared to cellulose, so that the enzymatic hydrolysis cannot perform well to result high sugar yield (Cheng et al., 2016).
Compared to pre-treated OPEFB and ST with higher lignin content, A-LD can work well in kraft pulp of SSB contained low lignin content. It means that lignin contributes significantly in inhibiting the enzyme work on the substrate. The mechanism of A-LD from kraft lignin as cellulase-aid agent to protect cellulase work effectively is shown in Fig. 3. These supported results were also reported in previous research (Winarni et al., 2013; Fatriasari et al., 2018; Fatriasari et al., 2020; Cheng et al., 2016).
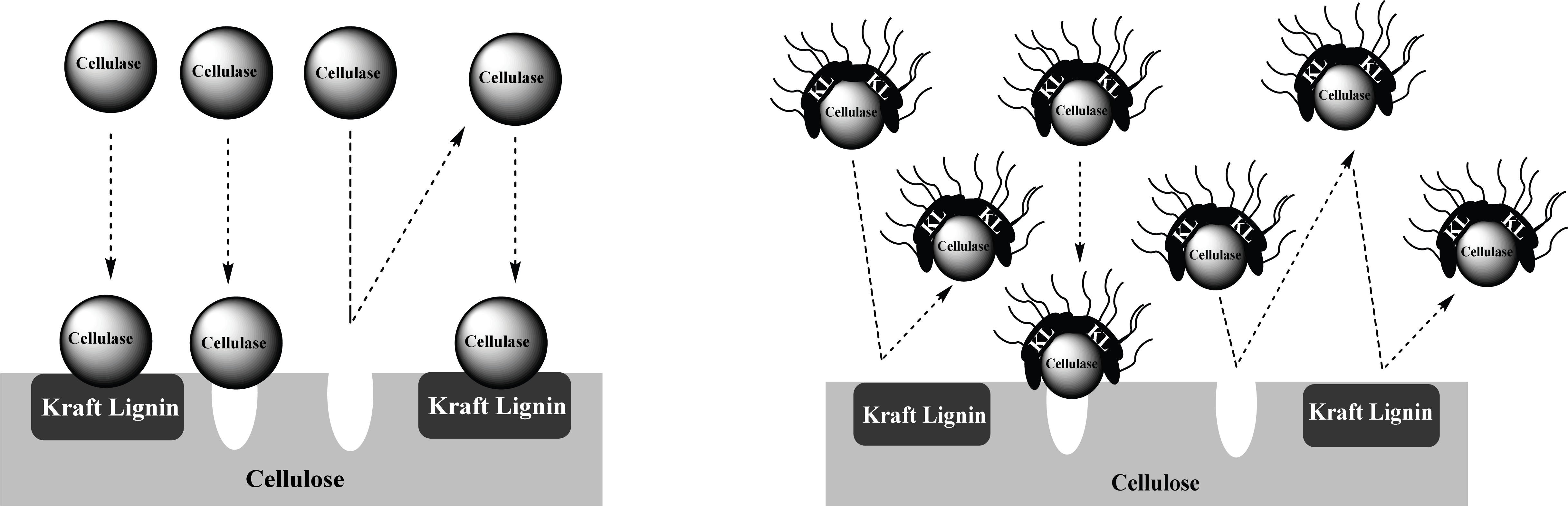
Fig. 4 presents the performance of reducing sugar yield per pulp of pretreated biomass. Based on these results, the similar tendency is presented on the reducing sugar yield per pulp after A-LD addition. This means that A-LD does not give significant improvement on reducing sugar yield per pulp except on ST pre-treated maleic acid. Removing lignin after pretreatment contributes on reducing sugar yield per pulp results. Previous study of Fatriasari et al. (2020) reported that addition of A-LD on kraft pulp of SSB successfully improved the reducing sugar yield. In this study, pretreated biomass that was used as substrates contained high lignin compared to kraft pulp of SSB.
The presence of lignin in pretreated biomass has affected in the enzyme work on substrate as can be seen in Fig. 4 and 5. The difference of A-LD types did not always give the same effects on reducing sugar yield per pulp and per biomass. It means that the effectiveness of A-LD is influenced not only by the chemical composition of biomass but also by the other factors such as crystallinity of biomass, the degree of disruption of pretreated biomass, the possibility re-deposited lignin on the surface of pretreated biomass after pretreatment that needs to be further investigated. SSB kraft pulp has the highest degree of crystallinity, which was caused by high removal of amorphous fractions (lignin and hemicellulose) after kraft pulping and provide high cellulose (Table 3). Even though ST pre-treated maleic acid and OPEFB pre-treated oxalic acid have lower crystallinity compared to the others, but it does not always improve on the reducing yield per biomass.
Pre-treatment method | Crystallinity index (%) |
---|---|
OPEFB pre-treated maleic acid | 44.97 |
OPEFB pre-treated oxalic acid* | 40.61 |
ST pre-treated maleic acid | 34.21 |
SSB pre-treated kraft pulping | 54.80 |
The different trend on reducing sugar yield per biomass after addition of A-LD indicated that the possibility of specific interaction between types of biosurfactant and the substates. A-LD was not able to inhibit the lignin binding on the substrate with active site of enzyme during enzymatic hydrolysis. The addition of A-LD in the enzymatic hydrolysis of kraft pulp of SSB leads to improve reducing sugars productivity, compared to control. In kraft pulp of SSB, the competitive adsorption between cellulase and A-LD might be won by A-LD. There is significant difference on the reducing sugar yield per biomass with high and low lignin content in pre-treated biomass. Lignin can be absorbed by cellulase that could be released by reaching new adsorption equilibrium between lignin and supernatants. On the other hand, desorption of cellulases from lignin occurred by hydrolytic capacity. The maximum absorption capacity of cellulases on cellulose presence of lignin decreased (Li et al., 2016). These results supported that lignin content contributed in improving reducing sugar yield besides the bio-surfactant effect. The reducing sugar yield per biomass of SSB kraft pulp is higher than that of jabon alkali pulp (42%) at the optimum condition of enzymatic hydrolysis using Tween 80 as surfactant (Nababan et al., 2020).
4. CONCLUSION
The evaluation of the effect of lignin content in pre-treated biomass on enzymatic hydrolysis performance of biomass has been performed. Lignin content in biomass has influence on the A-LD performance during enzymatic hydrolysis. The possibility of inhibition of lignin binding in substrate with A-LD addition could not be observed; therefore, there was no improvement of reducing sugar yield from substrates with high lignin content. A-LD can work effectively in improving enzymatic hydrolysis performance in biomass with low lignin content. This study confirmed that lignin has dominant effect in the effectiveness of cellulase activity during enzymatic hydrolysis. The types of A-LD did not give significant effects on reducing sugar yield improvement.