1. INTRODUCTION
Thymelaeceae is the major plant family producing agarwood, also called Gaharu in Indonesian. Agarwood have been used as one of the essential ingredients in fragrance, aromatherapy, pharmaceutical and herbal medicines for centuries. It also hold social, cultural, and economic values in local communities (Beek and Philips, 1999; Barden et al., 2000; Donovan and Puri, 2004). Indonesian agarwood has been known worldwide and retained long historical story. It was first recorded as the main commodity bartered between the empires of China and the kingdoms of Indonesia since the Silk Road era (Turjaman et al., 2016). Wider spectrums of agarwood uses have been known for sedative in oriental medicine, antimicrobial, antitumor and antioxidant activity (Takemoto et al., 2008; Wetwitayaklung et al., 2009; Chen et al., 2011). Agarwood is the resinous and fragrant heartwood that may not be formed in normal wood tissue but formed in wounded trees. Among them, Aquilaria and Gyrinops are the most common agarwood-producing genus in Indonesia (Gong and Guo, 2009). Different parts of various plant have been known to have various benefit (Jung et al., 2017. Ham and Kim, 2018; Li et al., 2018), healthy wood of Aquilaria and Gyrinops have been acknowledge to contain bioactive compound; leaves demonstrated inhibition activity against polymorphonuclear neutrophils (PMNs) respiratory burst stimulated by PMA (Qi et al., 2009), anti-diabetic, anti-HIV, anti-cancer, immuno-modulatory anti-inflammatory and laxative effects (Yoshimi et al., 2001); while fresh steam exhibited cytotoxicity against SGC-7901 and SMMC- 7721 cell lines (Wang et al., 2010).
Parallel with the huge loss of agarwood producing-tree in the wild, the losses of endophytic fungi associated with the trees automatically determine the loss of Indonesia biodiversity as well. Agarwood-producing tress are host for many endophytic fungi which produce similar or even higher amount of bioactive substrates compare to their host plant (Stierle et al., 1993; Strobel et al., 1996; Li et al., 1998). Previous studies have recorded that 1) Fusarium spp. isolated from leaves, steam and root of A. sinensis showed the most potent antimicrobial activity, 2) several endophytes fungi (Gong and Guo, 2009) isolated form A. crassna showed capability to produce indole-3-acetic acid (IAA) and siderophores (Nimnoi et al., 2010), 3) F. verticillioides SHTr3 and Colletotrichum truncatum SHTrHc7 isolated from A. crassna showed comparable scavenging abilities on DPPH-free radicals (Chi et al., 2016), 4) Xylaria mali, Lasiodiplodia theobromae and Phaeoacremonium rubrigenum isolated form A. sinensis exhibited cytotoxicity against 293-T, 293-T, and SKVO3 cells lines (Cui et al., 2011), and 5) fungus AL-2 strain isolated from A. malaccensis had antibacterial activities against gram positive and negative bacteria as well as general toxicity based on brine shrimp lethality (Shoeb et al., 2010). A. sinensis have been used as traditional Chinese medicinal, a tropical evergreen tree distributed in Hainan, Guangdong, Guangxi, Yunnan and Taiwan (Chen et al., 2016); A. crassna used also as traditional medical plant and widely distributed in Indochina and Thailand (Turjaman and Hidayat, 2017); and so that for A. malaccensis which is the most popular species for its agarwood production and distributed from different countries, Bangladesh, Bhutan, Cambodia, India, Indonesia, Iran, Lao PRD, Malaysia, Myanmar, Philippines, Singapore, Thailand, Vietnam and Papua New Guinea (Oldfield et al., 1998; Premalatha and Kalra, 2013).
The genus Aquilaria has 27 species spread worldwide, which 6 are found in Indonesia (Soehartono and Newton, 2000; Saikia and Khan, 2013). The genus Gyrinops is distributed in at least 12 countries, and 7 species were found naturally in Indonesia (Barden et al., 2000). Several studies have been previously conducted to those species, particularly on exploration and investigation of their phytochemical constituents and their respective endophytic fungi bioactivities. There was vast variations of endophytic fungal communities related to living conditions, host plant species, as well as host tissue types (Lamit et al., 2014); indicating that further investigations are important. For this reason, this study aimed to isolate the understudied endophytic fungi associated with three Indonesia-native species of agarwood (A. malaccensis and A. microcarpa, G. versteegii) and one exotic species (A. crassna), as well as their antioxidant and antifungal activities.
2. MATERIALS and METHODS
Alcohol, NaClO 4%, Dextrose, KOH 10%, HCl, Glycerol 20%, acetic acid anhydrite, magnesium (Mg), H2SO4, Folin–Ciocalteu (FC), ethyl acetate and methanol were purchased from Merck chemical (Germany). 1,1-diphenyl-2-picrylhydrazyl (DPPH) was purchased from Sigma-Aldrich chemical (USA) and other chemicals were provided by Himedia chemicals (India).
Different parts of plant materials (healthy segment; bark, leaves, shoot and root) from Aquilaria and Gyrinops trees originated from West Java and Bangka were collected (diameter breast high /dbh > 15 cm, either grown naturally or planted). The potato dextrose agar (PDA), which consist of potato extract 20%, dextrose 2%, and agar 2% was used for isolation. Pure fungal strains were sub-cultured and maintained on PDA prior to use. Malt Yeast Glucose Agar (MYGA, containing yeast extract 0.4%, malt extract 1%, sucrose 0.4%, and agar 2%), and Malt Yeast Glucose Pepton Agar (MYGPA, which consist of yeast extract 0.3%, malt extract 0.3%, peptone 0.5%, sucrose 1.05%, and agar 2%) were also used to obtain optimum fungal growth (Atlas, 2004).
Fungal strains were isolated from bark, leaves, shoot, and root of A. malaccensis, A. microcarpa, A. crasna and G. verstigii trees. The tree parts were collected at least from three different trees. In order to minimize the growth of epiphytic fungi during internal tissue sampling, tree parts were surface-sterilized by washing, followed by soaking in alcohol 70% for 2 min, and lastly in sodium hypochlorite for 2 min (4% Cl active). The outer tissues were removed, and the inner tissues of 0.5 x 0.5 cm size were carefully dissected and placed in PDA media plates. Chloramphenicol (100 mg/L) was added to media to suppress bacterial growth. The plates were then incubated at room temperature for up to 14 days, during which the development of different colonies was periodically observed and verified. Each colonies of different fungi appeared from tissue fragment were cut out by dissecting microscope and purified on PDA plates. All pure cultures obtained were used for further investigation.
To select the most suitable medium for each isolate, the pure cultures were tested on PDA, MYGA and MYGPA media. A disk (5 mm, diameter) of actively growing fungal colony on agar media was obtained with a cork-borer and was sub-cultured into petri dish containing PDA, MYGA and MYGPA media. The plate was incubated for 7 d, and the fungal growth was observed daily. All the experiments were conducted in triplicate.
F. solani was obtained from Laboratory of Forest Microbiology (INTROF-CC 00509, Forest Research and Development Centre, Bogor, Indonesia) All pure strains of fungal endophytes were screened against F. solani in dual cultures on PDA. An agar disk (5 mm diameter) of endophytic and F. solani was co-inoculated 4 cm apart on PDA in petri dish. The plates were incubated at room temperature up to 7 days. All experiments were carried out in triplicates. Growth inhibition percentage was determined by the following formula (Dennis and Webster, 1971).
Where J1 is radial covered by pathogen toward petri dish wall (outward), and J2 is radial covered by pathogen (F. solani) toward endophytic fungi.
Each of endophytic fungal isolates was grown in 250 mL Erlenmeyer flasks containing 50 ml of selected liquid medium (previously described) and incubated for 14 days in stationary condition. After incubation, mycelia biomass was harvested by filtering through Whatman No. 1 filter paper. The filter papers containing mycelia mat were oven dried until constant weight was reached, then extracted by ethyl acetate. The filtrate was also extracted by ethyl acetate using funnel separation. Each organic phase was mixed, and the solvent was then removed under reduced pressure using rotary vacuum evaporator to obtain a concentrate. The yield of ethyl acetate soluble was determined by calculation with dried weight of fungal mycelium (%, w/w) and volume of culture liquid medium (%, w/v).
Antioxidant activity of each extract was determined according to published methods with slight modifications (Kamonwannasit et al., 2013; Marson Ascêncio et al., 2014; Sadeghi et al., 2015). In a series of test tubes, extract was diluted with ethyl acetate to obtain in the range of concentration into which 0.25 mL of a DPPH (1 M) and added methanol to obtain 2.5 mL of final reaction volume. After 30 min incubation at room temperature, the absorbance was monitored at 517 nm. The ethyl acetatesolution without extracts was selected as control. All experiments were conducted in triplicated test. The inhibitory activity was calculated from [(A0 – A1)/A0] × 100, where A0 is the absorbance of the control, and A1 is the absorbance of the extract or standard. The antioxidant activity was expressed as IC50, in which the amount of sample necessary to reduce the initial DPPH concentration by half.
Phytochemical assay was carried out according to Tapwal et al. (2016) with slight modification, including detection of some important constituents (phenolic, flavonoid, tannin, steroid, terpenoid, and alkaloid). All ethyl acetate soluble obtained from extraction (previously described) with concentration of 1000 μg/L was subjected for phytochemical assay. In general, phenolic and tannin were reacted by ferric chloride test, flavonoid was determined by addition of magnesium and hydrochloric acid, steroid was monitored by addition of acetate anhydrate and sulfuric acid, terpenoid was determined by addition of sulfuric acid, and alkaloid was monitored by using Mayer’s and Wagner’s reagents.
Fungal identification was done with molecular methods, focusing on fungi with antioxidant and antifungal properties. DNA was obtained by extraction of mycelia cultured in 7 d grown on potato dextrose broth (PBD) using DNA Wizard Kit (Promega, USA) according to the published method (Kumar and Shukla, 2005). The internal transcribed spacer ITS region (Jellison and Jasalavich, 2000) was amplified by polymerase chain reaction (PCR) using ITS1 (5’-CTTGGTCATTTAG AGGAAGTAA-3’) and ITS4-B (5’-CAGGAGACTT GTACAGGTCCAG-3’). The PCR was performed in 20 μl of solution containing 10 ng of genomic DNA, 5 pmol each of the forward and reverse primers, and 10 μl of Go Taq® Hot Start Colourless Master Mix (Promega, Wisconsin USA) according to the manufacturer’s instructions. Prior to sequencing, the PCR products were purified using rAPid Alkaline Phosphatase (Roche, Germany) and exo-nuclease I (New England Biolabs, Massachusetts, USA). The purified PCR products were Sanger sequenced (First Base Sequencing Service, Singapore). Homology searches were conducted by using the BLASTn program in the National Center for Biotechnology Information (NCBI) GenBank database (http://www.ncbi.nlm.nih.gov/).
3. RESULTS and DISCUSSION
Total of 50 endophytic fungal isolate was successfully purified from 232 different part of fresh and healthy plant material belonging to A. malaccensis, A. microcarpa, A. crassna and G. Versteegii collected from West Java and Bangka Belitung Provinces. There were 24, 16, 7 and 3 pure endophytic fungal isolates from bark, leaves, shoot, and root, respectively. All fungal isolates were able to grow in all three media, with most of them showed highest colony growth in PDA (80%), MYGA (14%) and MYGPA (6%) (data not shown here). Hence, PDA and its equivalent liquid medium, potato dextrose broth (PDB), were selected for further investigation on antioxidant and antifungal properties. Endophytic microorganisms, mostly fungi in general, are localized internally in their host plants and become an important issue in the past few decades (Faeth, 2002; Staniek et al., 2008). Endophytic fungi thrive asymptomatically in the tissues of plants including stems, leaves, barks and/or roots. They may help clarify the role and biological change in stressful environments. Pure endophytic fungal isolates from G. versteegii were most abundant (21 strains) among other host plant species in this study; A. crassna (14 strain), A. microcarpa (7 strains) and A. malaccensis (3 strains). The isolation of endophytic fungi by traditional method in this study have revealed a new finding, particularly originated from G. verstegii and A. microcarpa, which has never been conducted before and could serve as a reference for the next studies. Whereas the diversity of endophytic fungi and their various potential bioactive metabolites from A. crassna and A malaccensis in this study has contributed to wider knowledge of the potential use of endophytic fungi for agarwood-producing trees as has been previously reports (Nimnoi et al., 2010; Shoeb et al., 2010; Chi et al., 2016).
In this study, 50 endophytic fungal isolates were evaluated for antioxidant activity. The results revealed that among 50 isolates, six had ability to reduce the absorbance of DDPH free radical with the range was 36% - 82% (Table 1); P1C1S3 (39.87 ± 2.61), P2CD3B (36.25 ± 0.01), P1DS1[C] (65.25 ± 0.73), P3DS1[A] (46.5 ± 2.33), P1B1C (40.67 ± 0.73), and R2MC3A (82.08 ± 5.99). Further analysis (Table 1) indicated that the ethyl acetate extracts from two isolates, R2MC3A and P1DS1[C] had IC50 of 60.92 μg/mL and 76.65 μg/mL, fall into the range of strong category (50-100 μg/mL). Whereas other isolates those had IC50 fall under the range of medium category (100-150 μg/mL). The ethyl acetate extract of R2MC3A resulted in higher yield, 0.06% (w/v) or 11.97% w/w of dried mycelia, than of P1DS1[C] (Table 2). These results indicated that the highest potential antioxidant was generated from endophytes isolated from fresh leaves (P1DS1[C]) and roots (R2MC3A) of G. verstegii and A. microcarpa trees.
Molecular identification clearly identified Diaporthe sp. (P1DS1[C]) and Apodus oryzae. (R2MC3A), both have IC50 less than 80 μg/mL (Table 3). Interestingly, Diaporthe sp. has been known as a genus of plant pathogenic, saprobic and endophytic fungi (Gomes et al., 2013); while Apodus oryzae (R2MC3A) has been known as aminor endophytic and rhizospheric fungi associated with several plants (Cai et al., 2006; Zhou et al., 2015). In contrast, Apodus oryzae isolate (R2MC3A) showed greater scavenging activity (IC50 60.92 μg/mL) than Diaporthe sp. (P1DS1[C]) isolate. This study is the first report to discover that Apodus oryzae (R2MC3A) and Diaporthe sp. played as endophytic fungi which resided in the leaves tissue of G. verstegii and the roots of A. microcarpa. Previous studies reported that only Fusarium, Colletotrichum, Xylaria, Lasiodiplodia, Phaeoacremonium, have been isolated from A. sinensis, A. crassna and A malaccensis (Gong and Guo, 2009; Nimnoi et al., 2010; Shoeb et al., 2010; Cui et al., 2011; Chi et al., 2016). Diaporthe sp. has been previously described as endophyte and produce bioactive secondary metabolites which are antioxidant potential. However, antioxidant activity between isolates varies greatly. For example, Diaporthe sp isolated from Costus spiralis have shown antioxidant activity (IC50) between 500-1750 μg/mL (Marson Ascêncio et al., 2014), whereas IC50 of 76.65 μg/mL were obtained from Diaporthe sp (P1DS1[C]) isolated from leaves tissue of G. verstegii. Diaporthe sp. was reported to produce bioactiverelated to phenolic compounds, such as benzene acetaldehyde, benzyl benzoate, salicylaldehyde, benzoin and benzyl cinnamate (Tanapichatsakul et al., 2018).
Other interesting finding is the antioxidant capacity of Apodus sp. Apodus sp. was previously reported to be found in Hedyotis diffusa and Pseudotsuga menziesii (Zhou et al., 2015; Daniels, 2017). However, the information on its antioxidant activity was not yet investigated. The antioxidant activity of Apodus oryzae (R2MC3A), among other isolates in this study, was the highest (60.92 μg/mL) and confirmed to contain a phenolic compound (Table 2); achieving the total phenolic content (TPC) 96.10 mg gallic acid equivalent per g sample (data not shown here). The detection of TPC content clearly supported other findings on antioxidant capacity closely related with TPC (Sadeghi et al., 2015; Jeong et al., 2017; Hidayat et al., 2018; Kim et al., 2018). Although, the specific phenolic compound was not yet identified, the phenolic content itself is important due to its unique structure for redox property which acts as high reducing agents, hydrogen donators, and singlet oxygen quenchers which may lead to antioxidant activity (Khoddami et al., 2013; Kim et al., 2017).
Antifungal activity tested by means of dual culture/confrontation against F. solani. Fusarium was recorded as a significant plant pathogen and an inducer of agarwood formulation (Sitepu et al., 2011; Turjaman et al., 2016). This pathogenic fungi was commonly used to induce agarwood formation and one of the most effective agent for agarwood formation in Indonesia (Turjaman et al., 2016). The effectiveness of agarwood formation was affected by many factors such as host tree, type of fungal pathogen, and environment (Santoso, 2013), as well as the presence of endophytic fungi inside the host tree (Nimnoi et al., 2011; Zhang et al., 2014). In this study, 50 endophytic fungi showed variation in the capability to inhibit the growth of F. solani (9-78%). Seven isolates inhibited the growth of F. solani over than 70%, (Table 1), those were P2CK1B (73%), P1D2A (72%), P1CD2B (72%), P2CD3A (77%), P3BS3[B] (78%), P3C2S3[B] (71%), and LMC3D11 (70%). Most above-ground tree isolates had strong antifungal activity against F. solani, while R2MC3A, which was originated from the root, had a weak antifungal activity. It revealed that R2MC3A was not compatible to work against F. solani, which produced symptoms on the infected root (Sitepu et al., 2011; Turjaman et al., 2016). More detailed observation on tissue origin and host plant species revealed that the strongest inhibitions (> 75%) were originated from shoot (P3BS3[B]) and bark (P2CD3A) of G. verstegii and A. crassna trees. According to molecular identification (Table 3), isolate P3BS3[B] and P2CD3A were clearly identified as P. theae and Curvularia sp.
The interaction between two potential endophytic and pathogenic fungi showed in Fig. 1. It was categorized as type E interaction (Wheeler and Hocking, 1993), where the mycelia of P. theae and Curvularia sp. continued to grow, covered and reduced pathogen’s colony. In the Curvularia sp - F.solani interaction, green black color appeared at the border of the two colonies. Yellow brown was appeared circling the mycelium of P. theae interacted with F.solani. This phenomenon showed that both colonies competed to access nutrients, secreted certain enzymes, and produced bioactive compounds to cease the opponent’s growth by changing of fungal cell membrane (Hamzah et al., 2018; Kim et al., 2016). As an indicator of bioactive compound production, the phytochemical assay from ethyl acetate extract of fungal culture of P. theae and Curvularia sp. assayed and showed a positive result to flavonoid, terpenoid and/or steroid constituent (Table 2). Compounds constituents from endophytes of Genus Pestalotiopsis and Curvularia have been previously reported (Tan and Zou, 2001; Kaul et al., 2012; Mondol et al., 2017; Alurappa and Chowdappa, 2018; Chutulo and Chalannavar, 2018; Deshmukh et al., 2018). Pestalotiopsis sp. with the huge diversity of endophytic fungi has appeared as potential source of bioactive natural compounds. They have been reported to produce various compounds such as alkaloids, terpenoids, flavonoids, and phenols, which has been reported to have antimicrobial, antifungal, antiviral antineoplastic, and antioxidant activities (Deshmukh et al., 2017). The terpenoid compounds isolated from the endophyte Pestalotiopsis sp. was the most abundant compound among other constituents, which are moderate to high antifungal property, such as 10-Deacetylbaccatin III, Pestalofone C, Pestalofone E, (3R,4R,6R,7S)-7- hydroxyl-3,7-dimethyl-oxabicyclo[3.3.1]nonan-2-one, and (3R,4R)-3-(7-methylcyclohexenyl)-propanoic acid (Deshmukh et al. 2017, 2018). Curvularia sp. isolated from Rauwolfia tetraphylla L. was reported to contain alkaloid and terpenoid, and had antifungal activity (Alurappa and Chowdappa, 2018). Pyrenolide A produced from Curvularia sp., strain M12, was isolated from Murraya koenigii and showed strong activity against Phytophthora capsici (Mondol et al., 2017). Studies on agarwood production in genera Aquilaria and Gyrinops reported that only 7-10% of tree contains agarwood, which sesquiterpenes and 2-(2-phenylethyl)- 4H-chromen-4-one was the major chemical constituent (Naef, 2011; Chen et al., 2012). Although terpenoids have been found in the host plant (agarwood) as well as on the potential endophytic fungi in this study, the relationship between them are still unclear; whether it is mutualistic, symbiotic, antagonistic, or slightly pathogenic.
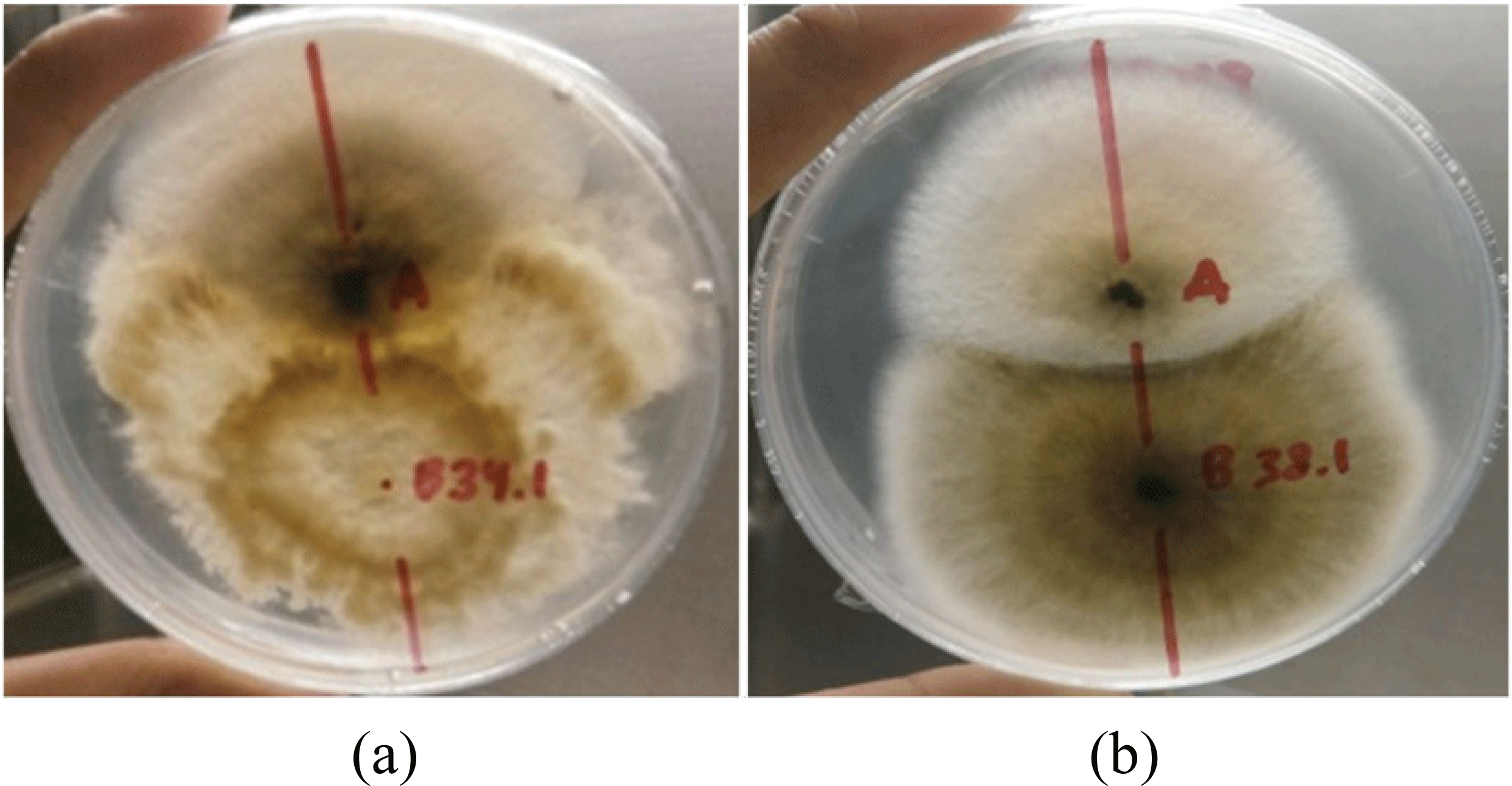
4. CONCLUSION
Fitty endophytic fungi were successfully isolated from different parts of A. malaccensis, A. microcarpa, A. crassna and G. verstegii trees grown in West Java and Bangka Belitung Provinces, Indonesia. Among them, Diaporthe sp. (P1DS1[C]) and A. oryzae. (R2MC3A) originated from fresh leave G. verstegii and root of A.microcarpa produced phenolic chemical groups which have antioxidant activities (IC50) less than 80 μg/mL. Two other isolates, P. theae (P3BS3[B]) and Curvularia sp (P2CD3A) originated from fresh shoot of G. verstegii and bark of A. crassna showed antifungal activity against the phytopathogenic fungi F. solani, potentially by producing terpenoid chemical groups constituent. New finding of the occurrence of endophytic fungi (Diaporthe sp., A. oryzae, P. theae, Curvularia sp.) may be used as the bioresources for antioxidant and also as antifungal agents for wider biotechnology applications in the future.