1. INTRODUCTION
Compression wood (CW) is defined as reaction wood on the lower sides of branches and leaning or crooked stems of coniferous trees and are characterized anatomically by highly lignified tracheids (Timell, 1986; Gardiner et al., 2014). CW functions to shift the leaning stem back to a vertical position by producing compression stress, which pushes the stem back to an upright position (Westing, 1965; Gardiner et al., 2014). It can be recognized by a darkened color on the transverse surface, typically in a wide crescent-shape (Kim et al., 2016). Lateral wood (LW) is defined as an intermediate between opposite wood (OW) and CW, also mentioned as normal wood (Timell, 1986). OW that is light brown in color is directly across from the pith of CW. Anatomical characteristics of CW are distinctively different than those of OW and LW (Timell, 1978; 1983; 1986; Eom, 1991; 1992; Xu and Eom, 2005). The existence of helical cavities, excessive lignification in the S2 layers, roundness of tracheid cells in cross sections, thicker cell walls, differently shaped pit borders, higher microfibril angles in the S2 layers, absence of S3 layer, and intercellular space were all considered as the anatomical characteristics of CW (Fujita et al., 1979; Yumoto et al., 1983; Timell, 1986).
As an ancient gymnosperm, many researchers have long been interested in the anatomical characteristics of Ginkgo biloba L. Scott et al. (1962) reported that tracheids in the wood of Ginkgo biloba has conifer-like characteristics, such as bordered pits and uniseriate rays; however, the tracheids were distinctly heterogeneous and had a disordered appearance in the transverse sections. Srivastava (1963) also reported that in young and old stems of Ginkgo contained two types of tracheids: one had wide lumens with thin cell walls and many pits, and another had narrow lumens with thicker cell walls and few or no pits. Idioblasts with druse were frequently found in Ginkgo biloba (Scott et al., 1962; Srivastava, 1963; Timell, 1978; Lee and Eom, 1987; 1988). The star shape of druses could be caused by many crystal components extending to the surface (IAWA committee, 2004).
A few studies had been reported the anatomical characteristics of CW and OW in Ginkgo biloba. Timell (1978) examined the ultrastructure of CW and normal wood in Ginkgo biloba using branch wood as the material. He reported that CW in Ginkgo biloba showed varied tracheids in size, angular outline of cell cross section, helical cavities, and similar ultrastructure to the CW of other gymnosperms. Eom (1991) investigated the anatomical characteristics between CW and OW in a branch of Ginkgo biloba and observed distinct differences in both qualitative and quantitative aspects.
In previous studies, the branch wood of Ginkgo biloba was usually used to investigate the anatomical characteristics of its CW (Timell, 1978; Eom, 1991). There was still a lack of information regarding the anatomical characteristics of CW, LW and OW in a stem of Ginkgo biloba. Therefore, this study aimed to understand the qualitative aspects of the anatomical characteristics for CW in a stem of Ginkgo biloba. These characteristics were then compared to those of LW and OW.
2. MATERIALS and METHODS
A Ginkgo biloba tree of 24 y was obtained from Hongcheon-gun, Gangwon-do, Republic of Korea (37.6970°N, 127.8887°E). The CW in a stem of Ginkgo biloba was dark brown in color, while LW and OW were light brown (Fig. 1). The fundamental information of experimental woods is shown in Table 1.
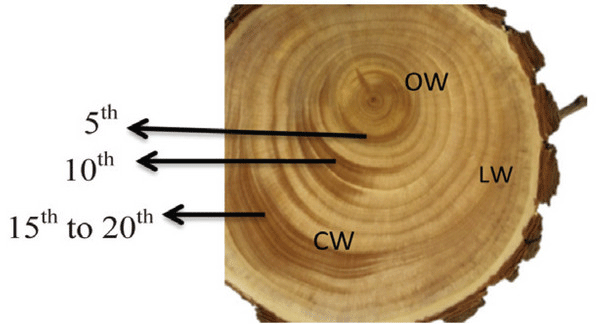
Samples | Age | DBH (cm) | Type | Examined annual ring | Density (g/cm3) |
---|---|---|---|---|---|
Ginkgo biloba | 24 | 15 | CW | 5th, 10th , and 15th to 20th | 0.50 |
LW | 5th, 10th , and 15th to 20th | 0.47 | |||
OW | 5th, 10th , and 15th to 20th | 0.51 |
Wood discs were converted to small blocks and softened in a boiling mixture of glycerine and water (50:50). The cross, tangential, and radial sections were cut into pieces with a 15-20 μm thickness using a microtome. All sections were stained with 1% safranin solution and dehydrated by grade series of alcohol (50%, 70%, 90%, 95%, and 99%) and xylene. Permanent slides were prepared with Canada balsam. All thin sections were observed under an optical microscope (Eclipse E600, Nikon, Japan) connected to an images analysis system (IMT i-Solution Lite).
The CW, LW, and OW samples were cut to a dimension of 10 mm3, air-dried, coated with gold using a Cressington sputter coater (ULVAC G-50DA, Japan), and observed under a scanning electron microscope (SEM) (JSM-5510, Japan, 15 kV).
3. RESULTS and DISCUSSION
Macroscopically, the CW in a disc of Ginkgo biloba had a dark brown color, while the LW and OW had a light brown color (Fig. 1). The CW in Coniferales, Ginkgoales, and Taxales was easily recognized by its color, which was darker than the surrounding tissues, while LW was considered as either normal wood or an intermediate between OW and CW (Timell, 1986).
The transition from earlywood to latewood in a stem of Ginkgo biloba is shown in Fig. 2. The CW and OW had an abrupt transition from earlywood to latewood, while the transition was gradual in LW. The transition was recognizable by the structural differences, usually in wall thickness and radial diameter of tracheid (IAWA Committee 2004). Lee and Eom (1987) reported that Ginkgo biloba exhibited a gradual transition from earlywood to latewood in normal wood stems. The transition from earlywood to latewood could be affected by many factors-one of which was CW (IAWA Committee 2004). Latewood portions in the cross surface have been measured according to Mork's definition under an optical microscope (Denne, 1989). In this study, CW had higher portion of latewood than OW and LW.
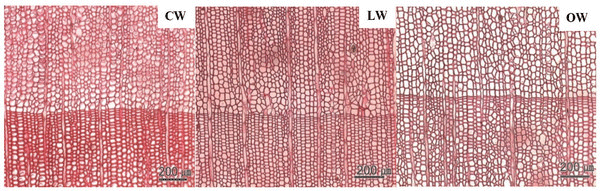
Scanning electron micrographs of Ginkgo biloba on transverse sections are shown in Fig. 3. The tracheids in CW had angular shape, varying lumen size, and several intercellular spaces. The CW in Ginkgo biloba had different anatomical characteristics from CW in other softwood species (Timell, 1986). Generally, CW showed distinctive anatomical characteristics, such as spiral checks, excessive lignification in the S2 layer, circular tracheid shape in the cross section, thicker cell walls, changes in the shape of pit borders, higher microfibril angles in the S2 layers, absence of S3 layers, and intercellular spaces (Timell, 1978; 1983; 1986; Fujita et al., 1979; Yumoto et al., 1983; Donaldson et al., 2004). LW and OW showed rectangular tracheids of varying sizes and fewer intercellular spaces compared to CW in cross section. Druses, as common characteristics of Ginkgo biloba, were shown in CW, LW, and OW. Idioblasts with druse were found in Ginkgo biloba, usually one idioblast contained one druse (Srivastava, 1963; Lee and Eom, 1988). Druse was defined as a compound crystal of calcium oxalate (Scott et al., 1962; Srivastava, 1963; IAWA committee, 2004). The star shape of druses could be caused by many crystal components extending to the surface (IAWA committee, 2004).
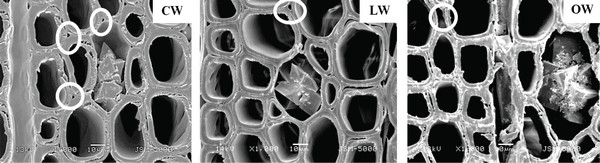
Scanning electron micrographs of Ginkgo biloba on the radial section are shown in Fig. 4. A radial section of CW revealed spiral or helical checks in the tracheid cell walls (Fig. 4). It was reported that the spiral or helical checks were present in CW of G. biloba (Onaka, 1949). However, several studies reported that the helical checks were absent in Ginkgo biloba (Timell, 1978; 1983; 1986; Yoshizawa, 1987a). Spiral checks are defined as the slopes of slits or helical lines on the cell wall and could be used to measure the microfibril angle in the S2 layer, as the slits of bordered pits were parallel to microfibril orientation occurring in the inner-tracheid walls (Timell, 1986; Hoadley, 1990). LW and OW showed bordered pits with a rounded and slightly oval-shaped pit apertures. The crossfields of CW had slit-like and often extended apertures (known as piceoid pits), while the LW and OW had cupressoid pits in the crossfields. Pit apertures could be affected by the existence of CW, as it could be intergrading between piceoid and cupressoid pits and between cupressoid and taxodioid pits (IAWA committee, 2004). The crossfields of Ginkgo biloba consisted of two to seven cupressoid pits in each field (Lee and Eom, 1987). The CW showed that the arrangement of tracheids was heavily distorted in earlywood (Fig. 5). The occurrence of abnormal or distorted tracheids gradually decreased during the transition from earlywood to latewood in CW (Yoshizawa et al., 1987b). Distorted tracheids in CW could be an effort to restore normal tracheid function by sliding or forcing the growth of the tracheids (Timell, 1986). A lack of space for growth made some tracheids expand abnormally, and they were displaced by the proximate cells (Yoshizawa et al., 1987b).
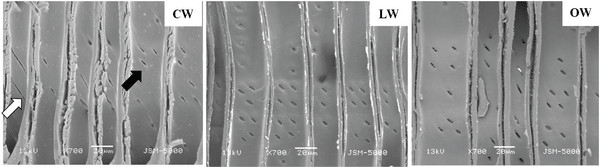
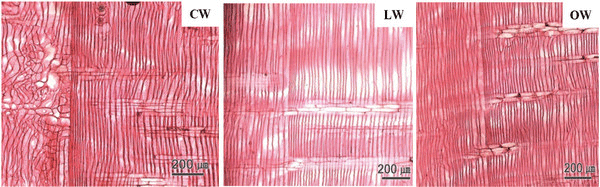
The types of rays can be investigated through a tangential section as shown in Fig. 6. CW, LW, and OW of Ginkgo biloba primarily contain uniseriate rays, while few biseriate rays were found (Fig. 6). Ginkgo biloba showed almost exclusively uniseriate rays, similar to other conifers (Scott et al., 1962).
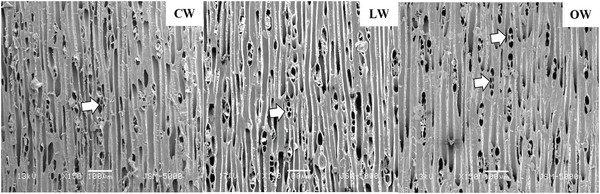
The tips of tracheid in CW were branched, while those of LW and OW presented a normal appearance (Fig. 7). The bifurcated tracheid tips were common characteristics in CW tracheids (Timell, 1986). The rapidity and quantity of elongation were determined by the interaction between inherent forces among each cell, which influenced the structural change of the tracheid tips in CW (Yoshizawa et al., 1987b).
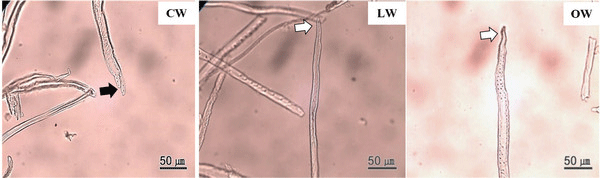
4. CONCLUSION
A cross section of CW and OW showed an abrupt transition from earlywood to latewood, while that of LW showed a gradual transition. A cross section of CW revealed circularly shape tracheids with or without angular outlines, many intercellular spaces, and varying lumen sizes. LW and OW showed rectangularly shape tracheids and fewer intercellular spaces compared to the CW, but they also presented varying lumen sizes. In a radial section, CW showed an irregular arrangement of earlywood tracheids, while LW and OW showed comparatively regular arrangements. CW possessed slit-like bordered pits along the tracheid cell wall, piceoid pits in the crossfield, and few spiral checks. LW and OW had bordered pits with slightly oval-shaped pit apertures and cuppresoid pits in the crossfields. Primarily uniseriate rays and few biseriate rays were observed in a tangential section of CW, LW, and OW. Tracheids of CW had bifurcated tips, while tracheids of LW and OW did not.